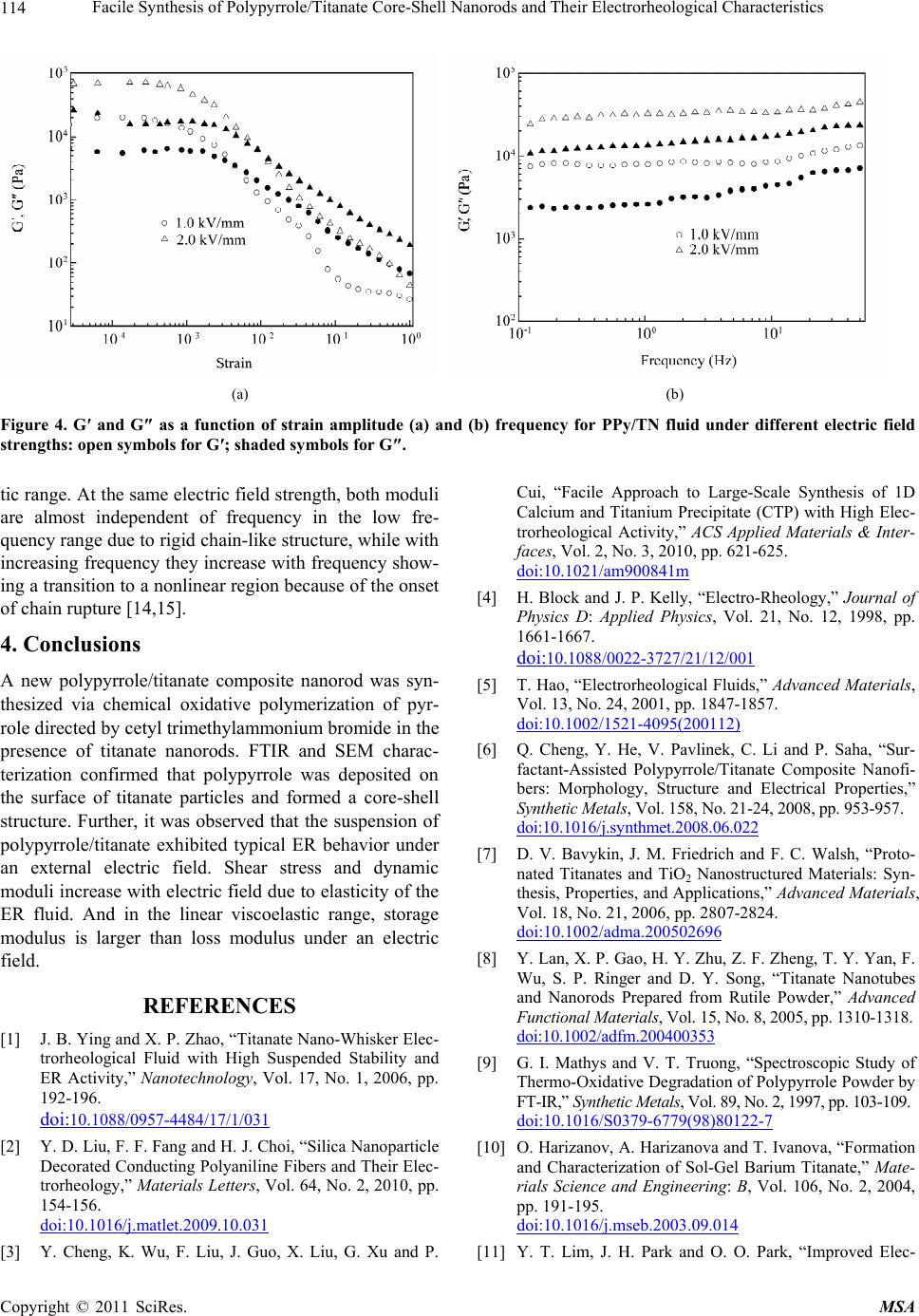
Facile Synthesis of Polypyrrole/Titanate Core-Shell Nanorods and Their Electrorheological Characteristics
Copyright © 2011 SciRes. MSA
114
(a) (b)
Figure 4. G′ and G″ as a function of strain amplitude (a) and (b) frequency for PPy/TN fluid under different electric field
strengths: open symbols for G′; shaded symbols for G″.
tic range. At the same electric field strength, both moduli
are almost independent of frequency in the low fre-
quency range due to rigid chain-like structure, while with
increasing frequency they increase with frequency show-
ing a transition to a nonlinear region because of the onset
of chain rupture [14,15].
4. Conclusions
A new polypyrrole/titanate composite nanorod was syn-
thesized via chemical oxidative polymerization of pyr-
role directed by cetyl trimethylammonium bromide in the
presence of titanate nanorods. FTIR and SEM charac-
terization confirmed that polypyrrole was deposited on
the surface of titanate particles and formed a core-shell
structure. Further, it was observed that the suspension of
polypyrrole/titanate exhibited typical ER behavior under
an external electric field. Shear stress and dynamic
moduli increase with electric field due to elasticity of the
ER fluid. And in the linear viscoelastic range, storage
modulus is larger than loss modulus under an electric
field.
REFERENCES
[1] J. B. Ying and X. P. Zhao, “Titanate Nano-Whisker Elec-
trorheological Fluid with High Suspended Stability and
ER Activity,” Nanotechnology, Vol. 17, No. 1, 2006, pp.
192-196.
doi:10.1088/0957-4484/17/1/031
[2] Y. D. Liu, F. F. Fang and H. J. Choi, “Silica Nanoparticle
Decorated Conducting Polyaniline Fibers and Their Elec-
trorheology,” Materials Letters, Vol. 64, No. 2, 2010, pp.
154-156.
doi:10.1016/j.matlet.2009.10.031
[3] Y. Cheng, K. Wu, F. Liu, J. Guo, X. Liu, G. Xu and P.
Cui, “Facile Approach to Large-Scale Synthesis of 1D
Calcium and Titanium Precipitate (CTP) with High Elec-
trorheological Activity,” ACS Applied Materials & Inter-
faces, Vol. 2, No. 3, 2010, pp. 621-625.
doi:10.1021/am900841m
[4] H. Block and J. P. Kelly, “Electro-Rheology,” Journal of
Physics D: Applied Physics, Vol. 21, No. 12, 1998, pp.
1661-1667.
doi:10.1088/0022-3727/21/12/001
[5] T. Hao, “Electrorheological Fluids,” Advanced Materials,
Vol. 13, No. 24, 2001, pp. 1847-1857.
doi:10.1002/1521-4095(200112)
[6] Q. Cheng, Y. He, V. Pavlinek, C. Li and P. Saha, “Sur-
factant-Assisted Polypyrrole/Titanate Composite Nanofi-
bers: Morphology, Structure and Electrical Properties,”
Synthetic Metals, Vol. 158, No. 21-24, 2008, pp. 953-957.
doi:10.1016/j.synthmet.2008.06.022
[7] D. V. Bavykin, J. M. Friedrich and F. C. Walsh, “Proto-
nated Titanates and TiO2 Nanostructured Materials: Syn-
thesis, Properties, and Applications,” Advanced Materials,
Vol. 18, No. 21, 2006, pp. 2807-2824.
doi:10.1002/adma.200502696
[8] Y. Lan, X. P. Gao, H. Y. Zhu, Z. F. Zheng, T. Y. Yan, F.
Wu, S. P. Ringer and D. Y. Song, “Titanate Nanotubes
and Nanorods Prepared from Rutile Powder,” Advanced
Functional Materials, Vol. 15, No. 8, 2005, pp. 1310-1318.
doi:10.1002/adfm.200400353
[9] G. I. Mathys and V. T. Truong, “Spectroscopic Study of
Thermo-Oxidative Degradation of Polypyrrole Powder by
FT-IR,” Synthetic Metals, Vol. 89, No. 2, 1997, pp. 103-109.
doi:10.1016/S0379-6779(98)80122-7
[10] O. Harizanov, A. Harizanova and T. Ivanova, “Formation
and Characterization of Sol-Gel Barium Titanate,” Mate-
rials Science and Engineering: B, Vol. 106, No. 2, 2004,
pp. 191-195.
doi:10.1016/j.mseb.2003.09.014
[11] Y. T. Lim, J. H. Park and O. O. Park, “Improved Elec-