 Open Journal of Ophthalmology, 2013, 3, 127-133 Published Online November 2013 (http://www.scirp.org/journal/ojoph) http://dx.doi.org/10.4236/ojoph.2013.34027 Open Access OJOph 127 Nanobiotechnology in the Management of Glaucoma* Pho Nguyen1, Alex Huang1, Samuel C. Yiu2,3# 1Department of Ophthalmology, Keck School of Medicine of the University of Southern California, Los Angeles, USA; 2The Wilme r Eye Institute, Baltimore, USA; 3King Khaled Eye Specialist Hospital, Riyadh, KSA. Email: #syiu2@jhmi.edu Received September 2nd, 2013; revised October 5th, 2013; accepted October 15th, 2013 Copyright © 2013 Pho Nguyen et al. This is an open access article distributed under the Creative Commons Attribution License, which permits unrestricted use, distribution, and reproduction in any medium, provided the original work is properly cited. ABSTRACT As the prevalence of glaucoma continues to rise, clinicians and researchers are confronted with an age-old problem: how to reduce risk factors and preserve vision in glaucoma. Current management options revolve around a validated paradigm—intraocular pressure reduction. Active investigations to improve drug delivery efficacy and surgical out- comes are flourishing. This article aims to provide the interested readers with a review of recent discoveries in nano- biotechnology for the management of glaucoma. Targeted drug-delivery systems using mesoscale vectors demonstrate promising delivery profiles. The utility of nanoparticulate therapies to support retinal ganglion cell survival is being investigated. Studies to modulate tissue regeneration and remodeling and improve post-trabeculectomy outcomes are underway. Though these modalities promise new avenues to manage glaucoma, immediate market availability is not anticipated soon. Keywords: Glaucoma; Nanotechnology; Nanomedicine; Drug Delivery; Wound Healing; Nanoparticle 1. Introduction Glaucoma is a group of diseases having characteristic optic neuropathy with associated visual function deficits. Early disease detection and vision preservation con stitute the management of glaucoma. Currently, therapeutic strategies involve medical and surgical reduction of in- traocular press ure (IOP). However, maintaining lo cal ther- apeutic bioavailability of hypotensive agents remains a challenge in the field of ophthalmic drug delivery. Sur- gical modalities are effective but have associated com- plications [1]. Given that 60 million people worldwide are afflicted by this disease [2] and 27% of those af- flicted are at risk of developing glaucoma-related blind- ness in one eye after 20 years [3], the demand for viable treatment alternatives, particularly improved drug deliv- ery models, is self-evident. 2. Nanobiotechnologies for Glaucoma Management Previously, many clinical trials demonstrated that initial medical management is an effective option for IOP re- duction and vision preservation [4-11]. Although topical instillation is the predominant route, ensuring effective local concentration is a fundamental challenge. Precor- neal factors such as small load, poor tissue penetration, mechanical removal by tears and blinking, and nasolac- rimal elimination prevent the medication from reaching a sustained therapeutic level and necessitate multiple dos- ings per day, ultimately, leading to poor patient adher- ence [12-14]. Other studies further report that many pa- tients waste much of the topical medications because of incorrect instillation techniques [13-15]. Systemic ab- sorption may precipitate adverse effects [16-21]. There- fore, efforts are directed toward developing better drug delivery systems, e.g. ophthalmic inserts, smart hydrogel, vesicular and particulate carrier systems. *Financial Support(s): Alex Huang is supported by the Heed Ophthal- mic Foundation. Pho Nguyen is supported by the Heed Ophthalmic Foundation and the Fletcher Jones Foundation. Financial Disclosure(s): The authors have no financial interests in the topic of this manuscript. No conflicting relationship exists for any author. #Corresponding author. Nanoscale drug delivery systems offer the therapeutic advantages of targeted tissue penetration, enhanced re- lease kinetics, and increased local biodistribution [22-24]. In the field of tissue engineering and regenerative medi-
 Nanobiotechnology in the Management of Glaucoma 128 cine, the biologic length scale of nanostructures promotes meaningful interactions for tissue repa ir and regeneration [23]. “Nano” was originally defined for the semiconduc- tor industry as having at least one dimension less than 100 nanometer; but the definition of “nano-” has been recast in an operational fashion and many authors use “nano-” to include biologically relevant length scale, such as larger macromolecules and organelles for the fields of nanobiotechnology and nanomedicine [22-24]. Below, we summarize the recent discoveries in nanobio- technology for the management of glaucoma. 2.1. Drug Delivery Systems 2.1.1. Vesicular Platforms—Liposomes, Discomes , and Niosomes Modeled after biologic organelles, liposomal systems are designed fo r encapsulation of therapeutic agen ts for drug delivery and have found to have increased efficacy and decreased toxicity. Their diverse systemic applications encompasses liposomal daunorubicin for Kaposi’s sar- coma, micellar paclitaxel for ovarian and breast cancer, and liposomal amphotericin B for systemic fungal infec- tions or leishmaniasis. Surface modifications, such as PEGylation of the liposome, extend systemic circulating time and reduce toxicity. Liposomal preparations of topical ophthalmic medica- tions are particularly well studied for ocular surface in- fections. Use of liposomal amphotericin B for the treat- ment of fungal keratitis has been repo rted to significan tly reduce toxicity compared to non-liposomal formulation [25,26]. Topical liposomal acyclovir shows better deliv- ery than commercial acyclovir ointment in in vitro stud- ies and animal models [27]. Liposomal formulations of gatifloxacin, ciprofloxacin and fluconazole have shown prolonged delivery profiles, leading to better in vitro transcorneal permeation compared to aqueous formula- tions [28-30]. Other investigators are evaluating the feasibility of liposome-encapsulated ocular antihypertensive drugs. Topical liposomal and niosomal formulations of aceta- zolamide, a carbonic anhydrase inhibitor, are being in- vestigated for their ability to overcome acetazolamide’s limited aqueous solubility and improve its corneal per- meation [31-34 ]. In vitro and in vivo studies demonstrate sustained release kinetics with good intraocular hypoten- sive effect [32]. In addition to liposomes, other vesicular platforms, such as discomes and niosomes, are also being explored for ocular antihypertensive medications. Discomes are non-ionic surfactant-based discoidal vesicles, which also improve drug delivery. Some authors have reported that discoidal vesicles containing timolol maleate produce a sustained activity profile upon introduction to the ocular cavity [35]. Niosomes are another non-ionic bilayered vesicle that can entrap both hydrophilic and lipophilic drugs, either in the aqueous layer or in the vesicular membrane. Compared to liposomes, niosomes offer some advantages, such as chemical stability and lower produc- tion cost. A recent study reported that a mucoadhesive- coated niosomal system for timolol maleate can achieve higher peak concentrations for an extended period com- pared to the conventional dose form [36]. Sizes of these discomes and niosomes are in the micron range [35,36]. Lastly, a more recent nanovesicular formulation of bri- monidine tartrate has been constructed using sorbitan stearate and cholesterol, and the authors report signifi- cant intraocular pressure-lowering activity for a pro- longed period of time compared to the commercial pre- paration [37-39]. Most of the above studies report no short-term toxicity to the ocular surface of the animal models. 2.1.2. Nanop a rti cul ate Platforms Development of nanoparticulate platforms for drug de- livery has gained significant traction over recent years. The nanoscale size range and surface functionalization offer the advantages of targeted delivery and resistance to degradation. Particulate delivery systems also provide more stable storage media, compared to vesicular sys- tems. Biodegradable polymeric platforms present an ex- citing opportunity for investigators, where the pharma- cokinetics is determined by the particulate size and car- rier material, which is ultimately controlled by synthesis technologies. Biodegradable poly(lactic-co-glycolic) acid (PLGA) polymers have promise as potential couriers for anti- hypertensive agents. Timolol maleate integrated into PLGA polymers via a solvent evaporation method has been reported to have sustained release kinetics [40]. The release mechanism is proposed to be polymeric degrada- tion, rather than microporous diffusion. Another study suggests that PLGA and poly(l-lactide) acid (PLLA) mi- cro- and nanoparticles can deliver timolol maleate con- tinually over a 3-month period [41]. Intravitreal injection of PLGA is safe in a rabbit model, where only a localized foreign body reaction is reported [42]. In this study, the authors observed that the choroid and retina maintain normal appearence and no clinical inflammation was detected. Accordingly, some investigators are optimistic that these biodegradable polymeric micro- and nano- spheres may find application as subconjunctival depots to improve patient adherence [41]. Similarly, nanoparticu- late platforms for carbonic anhydrase inhibitors are being investigated as well. Methazolamide nanoparticles are reported to have longer and higher therapeutic efficacy compared to suspension formulation and commercial eye drops [43,44]. Open Access OJOph
 Nanobiotechnology in the Management of Glaucoma 129 Another polymer of ophthalmic interest is chitosan, a polycationic biodegradable polymer composed of re- peating glucosamine units. The chitosan nanoparticles are thought to have longer precorneal residence time and increased corneal penetration. Gatifloxacin-loaded chi- tosan nanoparticles show sustained released kinetics [45]. Chitosan/polylactic acid nanoparticles containing rapa- mycin demonstrate improved corneal allograft survival in a rabbit model compared to aqueous suspension [46]. With respect to ocular anti-hypertensive agents, nanopar- ticulate formulations of chitosan and timolol maleate or dorzolamide hydrochloride have also been described [47]. These investigators find that modification of these nanoparticles with hyaluronic acid, which improves mu- coadhesion, significantly decreases intraocular pressure in a rabbit model, compared to plain solutions of these drugs. 2.1.3. Dendrimeric Platforms Dendrimers comprise a fascinating drug carrier system. These hyperbranched globular macromolecules with den- dritic subunits have modifiable properties depending on their subunits and surface terminal groups. Therapeutic agents can either be encapsulated in the core of the den- drimer scaffold or conjugated on the surface. The den- drimer diameter can be customized based on its genera- tion and terminal groups. Some therapeutic agents being investigated include propranolol, sulfasalazine, folic acid, adriamycin, meth- otrexate, paclitaxel, and penicillin. Photodynamic ther- apy and gene therapy using dendrimers for corneal, reti- nal, and choroidal neovascularization have been pub- lished [48-50]. For glaucoma, poly(amidoamine) den- drimeric constructs have been constructed to deliver pi- locarpine and tropicamide [51]. These dendrimers appear to have greater corneal residence time, increased bio- availability, and low ocular irritation index in animal model. A recent study reported increased uptake of ti- molol maleate and brimonidine using poly(amidoamine) dendrimer hydrogel as delivery modality in bovine cor- neal model [52]. 2.2. Tissue Protection Currently, IOP control drives glaucoma management. However, some investigators are evaluating the feasibil- ity of neuroprotection as well. Apoptosis of retinal gan- glion cells (RGC) has b een associated with glaucomatous optic neuropathy [53]. Studies using RGC death after optic nerve injury in an animal mode l suggest that n euro- trophic factors, e.g. brain-derived growth factors, insu- lin-like growth factors, glial-derived neurotrophic factor, and ciliary neurotrophic factors (CNTF) may support RGC survival [54-56]. Accordingly, the roles of neuro- trophic factors in neuroprotection in glaucoma are being explored. Lentiviral-mediated transfer of CNTF into Schwann cells for optic nerve repair has been found to significantly increase RGC survival in animal models [57]. Others investigators have used biodegradable PLGA polymers to construct nano- and microspheres for CNTF encapsulation [58]. These authors report bioactivity in an in vitro neural stem cell model and note that the process of protein encapsulation with the polymer did not reduce its potency. Others incorporate CNTF nanospheres into photopolymerizable hydrogel to create a tissue engineer- ing scaffold with intrinsic sustained drug delivery capa- bility [59]. This scaffold provides an enhanced substra- tum for neural tissue repair and regeneration. Recently, induction of heat shock protein for neuroprotection using superparamagnetic nanoparticles has also been reported [60]. In the near future, successful commercial deploy- ment of these and other developments will add another set of tools in the armamentarium of the glaucoma spe- cialist to preserve vision. 2.3. Modulation of Postsurgical Wound Healing in Filtration Surgery Successful glaucoma filtering surgery necessitates ade- quate passage of the aqueous humor from the anterior chamber to an extraocular reservoir. Exuberant cicatricial changes to the conjunctiva following glaucoma filtration procedures present a threat to long-term success [61,62]. Antifibrotic agents have been demonstrated to promote longer bleb survival but are associated with severe com- plications such as leakage, infection, hypotony, and en- dophthalmitis [63-65]. Currently, antifibrotic agents are mostly frequently introduced intraoperatively via a soaked sponge. As with drug delivery of intraocular pressure lowering medications, nanobiotechnology can be directed toward improvement of bleb survival. A disc carrying biodegradable poly(lactic acid) mi- crospheres loaded with 5-fluorouracil for subconjunctival implant has been developed and tested in a rabbit model [66]. The authors show that delivery of 5-fluorouracil using the carrier resulted in greater decrease in intraocu- lar pressure, prolonged bleb persistence, and less corneal toxicity. Syntheses of various nanoparticulate 5-fluorour- acil and mitomycin have also been reported [67-69]. However, ophthalmic applications of these nanoparticles have yet to be investigated. Other investigators target the growth factor mediated cellular proliferation. In one study, glucosamine and glucosamine 6-sulfate dendrimers were used to target fibroblast growth factor-2 mediated endothelial cell pro- liferation and neoangiogenesis in a rabbit model of scar tissue formation after glaucoma filtration surgery [70]. The authors report that postoperative day 30 bleb sur- vival improves from 30% to 80%. Histologic examina- tion revealed less cicatricial proliferation in the den- Open Access OJOph
 Nanobiotechnology in the Management of Glaucoma 130 drimer group. In another approach, biodegradable porous PLGA microspheres containing antisense-TGF-β2 oli- gonucleotide nanocomplexes have been found to pro- mote bleb survival in a rabbit model of filtering surgery [71]. The antisense oligonucleotide reduces synthesis of the cytokine TGF-β2, which promotes wound healing. In this study, the nanocomplexes are encapsulated into po- rous microspheres and administered subconjunctivally. The authors report increased penetration of the encapsu- lated oligonucleo tides in conjunctival cells and increased time to filtering bleb failure. Steroids may also be pack- aged via nanoparticles to modulate postoperative wound healing. Dexamethasone entrapped in biodegradable poly(d,l-lactide-co-glyco lide) (PLGA) nanoparticles has been reported [72]. These nanoparticles can find applica- tions both in preventing filtering bleb scarring and in the investigation of steroid response in glaucoma. Viral vectors constitute another form of particulate nanoparticles that can be directed toward glaucoma sur- gical management. Adenovirus-mediated gene therapy has been performed to prevent bleb scarring in a rabbit model of glaucoma filtration surgery [73]. The authors find that topical, intraoperative application of recombi- nant adenovirus containing the human p21 gene demon- strates inhibition of wound healing and fibroproliferation after filtration surgery, comparable to mitomycin but with fewer adverse effects. Similar results are seen with an Ad-p27 vector [74]. Interestingly, attempts to use viral vectors to blunt steroid response are also underway. Novel glucocorticoid-inducible adenovirus vectors have been developed to overproduce metalloprotein 1, which degrades collagen type I after specific activation by dexamethasone [75]. Therefore, patients who presumably need long-term steroid treatment may benefit by having increased metalloproteinase activity to aid trabecular flow while on st eroi d s. 3. Conclusion This brief review highlights the recent advances and ad- vantages of nanobiotechnology for improving our under- standing of glaucoma and its management. In essence, nanobiotechnolo gy offers th e potential f or more effectiv e delivery of pharmaceutical agents that can influence both the medical and surgical arms of glaucoma management. REFERENCES [1] S. J. Gedde, J. C. Schiffman, W. J. Feuer, et al., (Tube versus Trabeculectomy Study Group), “Three-Year Fol- low-Up of the Tube versus Trabeculectomy Study,” Ame- rican Journal of Ophthalmology, Vol. 148, No. 5, 2009, pp. 670-684. http://dx.doi.org/10.1016/j.ajo.2009.06.018 [2] H. A. Quigley, “Glaucoma,” Lancet, Vol. 377, No. 9774, 2011, pp. 1367-1377. http://dx.doi.org/10.1016/S0140-6736(10)61423-7 [3] M. G. Hattenhauer, D. H. Johnson, H. H. Ing, et al., “The Probability of Blindness from Open-Angle Glaucoma,” Ophthalmology, Vol. 105, No. 9, 1998, pp. 2099-2104. http://dx.doi.org/10.1016/S0161-6420(98)91133-2 [4] M. A. Kass, D. K. Heuer, E. J. Higginbotham, et al., (For the Ocular Hypertension Treatment Study Group), “A Randomized Trial Determines That Topical Ocular Hy- potensive Medication Delays or Prevents the Onset of Primary Open-Angle Glaucoma,” Archives of Ophthal- mology, Vol. 120, No. 6, 2002, pp. 701-713. http://dx.doi.org/10.1001/archopht.120.6.701 [5] S. Miglior, T. Zeyen, N. Pfeiffer, et al., “Results of the European Glaucoma Prevention Study,” Ophthalmology, Vol. 112, No. 9, 2005, pp. 366-375. http://dx.doi.org/10.1016/j.ophtha.2005.06.020 [6] J. Burr, A. Azuara-Blanco and A. Avenell, “Medical ver- sus Surgical Interventions for Open Angle Glaucoma,” Cochrane Database of Systematic Reviews, Vol. 18, 2005, Article ID: CD004399. [7] M. C. Leske, A. Heijl, L. Hyman, et al., “Predictors of Long-Term Progression in the Early Manifest Glaucoma Trial,” Ophthalmology, Vol. 114, No. 11, 2007, pp. 1965- 1972. http://dx.doi.org/10.1016/j.ophtha.2007.03.016 [8] M. A. Kass, M. O. Gordon, F. Gao, et al., (For the Ocular Hypertension Treatment Study Group), “Delaying Treat- ment of Ocular Hypertension,” Archives of Ophthalmol- ogy, Vol. 128, No. 3, 2010, pp. 276-287. http://dx.doi.org/10.1001/archophthalmol.2010.20 [9] A. C. S. Crichton, P. Harasymowycz, C. M. L. Hutnik, et al., “Effectiveness of Dorzolamide-Timolol (Cosopt) in Patients Who Were Treatment Naive for Open-Angle Glaucoma or Ocular Hypertension: The COSOPT First- Line Study,” Journal of Ocular Pharmacology and Ther- apeutics, Vol. 26, No. 5, 2010, pp. 503-511. http://dx.doi.org/10.1089/jop.2010.0032 [10] D. C. Musch, B. W. Gillespie, P. R. Lichter, et al., “Vis- ual Field Progression in the Collaborative Initial Glau- coma Treatment Study: The Impact of Treatment and Other Baseline Factors,” Ophthalmology, Vol. 116, No. 2, 2009, pp. 200-207. http://dx.doi.org/10.1016/j.ophtha.2008.08.051 [11] L. J. Katz, W. C. Steinmann, A. Kabir, et al., “Selective Laser Trabeculoplasty versus Medical Therapy as Initial Treatment of Glaucoma: A Prospective, Randomized Trial,” Journal of Glaucoma, Vol. 21, No. 7, 2011, pp. 460-468. [12] N. M. Davies, “Biopharmaceutical Considerations in To- pical Ocular Drug Delivery,” Clinical and Experimental Pharmacology and Physiology, Vol. 27, No. 7, 2000, pp. 558-562. http://dx.doi.org/10.1046/j.1440-1681.2000.03288.x [13] T. Tsai, A. L. Robin and J. P. Smith III, “An Evaluation of How Glaucoma Patients Use Topical Medications: A Pilot Study,” Transactions of the American Ophthal- mological Society, Vol. 105, 2007, pp. 29-33. [14] C. M. Olthoff, J. G. Hoevenaars, B. W. van den Borne, et al., “Prevalence and Determinants of Non-Adherence to Topical Hypotensive Treatment in Dutch Glaucoma Pa- tients,” Graefe’s Archive for Clinical and Experimental Open Access OJOph
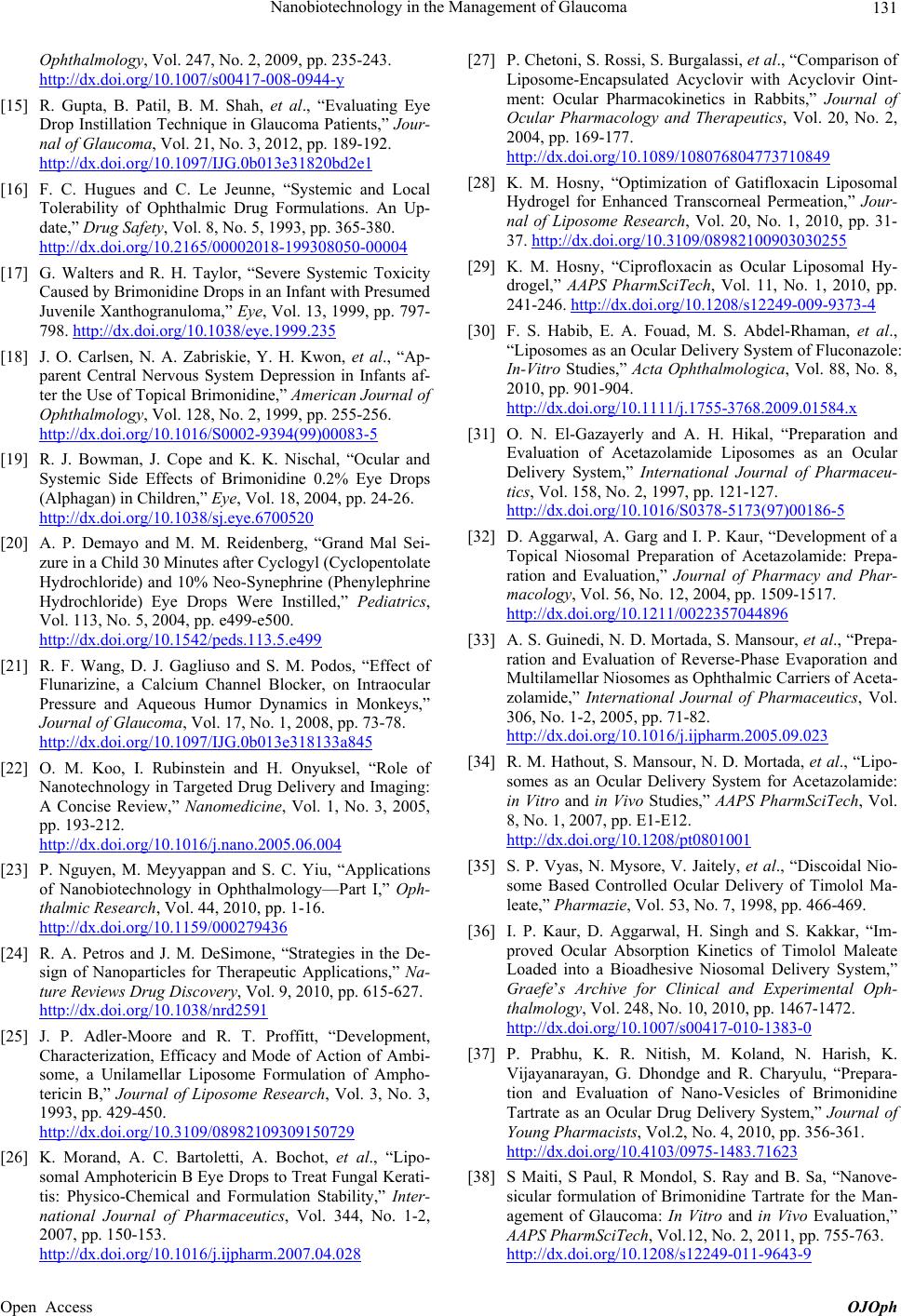 Nanobiotechnology in the Management of Glaucoma 131 Ophthalmology, Vol. 247, No. 2, 2009, pp. 235-243. http://dx.doi.org/10.1007/s00417-008-0944-y [15] R. Gupta, B. Patil, B. M. Shah, et al., “Evaluating Eye Drop Instillation Technique in Glaucoma Patients,” Jour- nal of Glaucoma, Vol. 21, No. 3, 2012, pp. 189-192. http://dx.doi.org/10.1097/IJG.0b013e31820bd2e1 [16] F. C. Hugues and C. Le Jeunne, “Systemic and Local Tolerability of Ophthalmic Drug Formulations. An Up- date,” Drug Safety, Vol. 8, No. 5, 1993, pp. 365-380. http://dx.doi.org/10.2165/00002018-199308050-00004 [17] G. Walters and R. H. Taylor, “Severe Systemic Toxicity Caused by Brimonidine Drops in an Infant with Presumed Juvenile Xanthogranuloma,” Eye, Vol. 13, 1999, pp. 797- 798. http://dx.doi.org/10.1038/eye.1999.235 [18] J. O. Carlsen, N. A. Zabriskie, Y. H. Kwon, et al., “Ap- parent Central Nervous System Depression in Infants af- ter the Use of Topical Brimonidine,” American Journal of Ophthalmology, Vol. 128, No. 2, 1999, pp. 255-256. http://dx.doi.org/10.1016/S0002-9394(99)00083-5 [19] R. J. Bowman, J. Cope and K. K. Nischal, “Ocular and Systemic Side Effects of Brimonidine 0.2% Eye Drops (Alphagan) in Children,” Eye, Vol. 18, 2004, pp. 24-26. http://dx.doi.org/10.1038/sj.eye.6700520 [20] A. P. Demayo and M. M. Reidenberg, “Grand Mal Sei- zure in a Child 30 Minutes after Cyclogyl (Cyclopentolate Hydrochloride) and 10% Neo-Synephrine (Phenylephrine Hydrochloride) Eye Drops Were Instilled,” Pediatrics, Vol. 113, No. 5, 2004, pp. e499-e500. http://dx.doi.org/10.1542/peds.113.5.e499 [21] R. F. Wang, D. J. Gagliuso and S. M. Podos, “Effect of Flunarizine, a Calcium Channel Blocker, on Intraocular Pressure and Aqueous Humor Dynamics in Monkeys,” Journal of Glaucoma, Vol. 17, No. 1, 2008, pp. 73-78. http://dx.doi.org/10.1097/IJG.0b013e318133a845 [22] O. M. Koo, I. Rubinstein and H. Onyuksel, “Role of Nanotechnology in Targeted Drug Delivery and Imaging: A Concise Review,” Nanomedicine, Vol. 1, No. 3, 2005, pp. 193-212. http://dx.doi.org/10.1016/j.nano.2005.06.004 [23] P. Nguyen, M. Meyyappan and S. C. Yiu, “Applications of Nanobiotechnology in Ophthalmology—Part I,” Oph- thalmic Research, Vol. 44, 2010, pp. 1-16. http://dx.doi.org/10.1159/000279436 [24] R. A. Petros and J. M. DeSimone, “Strategies in the De- sign of Nanoparticles for Therapeutic Applications,” Na- ture Reviews Drug Discovery, Vol. 9, 2010, pp. 615-627. http://dx.doi.org/10.1038/nrd2591 [25] J. P. Adler-Moore and R. T. Proffitt, “Development, Characterization, Efficacy and Mode of Action of Ambi- some, a Unilamellar Liposome Formulation of Ampho- tericin B,” Journal of Liposome Research, Vol. 3, No. 3, 1993, pp. 429-450. http://dx.doi.org/10.3109/08982109309150729 [26] K. Morand, A. C. Bartoletti, A. Bochot, et al., “Lipo- somal Amphotericin B Eye Drops to Treat Fungal Kerati- tis: Physico-Chemical and Formulation Stability,” Inter- national Journal of Pharmaceutics, Vol. 344, No. 1-2, 2007, pp. 150-153. http://dx.doi.org/10.1016/j.ijpharm.2007.04.028 [27] P. Chetoni, S. Rossi, S. Burgalassi, et al., “Comparison of Liposome-Encapsulated Acyclovir with Acyclovir Oint- ment: Ocular Pharmacokinetics in Rabbits,” Journal of Ocular Pharmacology and Therapeutics, Vol. 20, No. 2, 2004, pp. 169-177. http://dx.doi.org/10.1089/108076804773710849 [28] K. M. Hosny, “Optimization of Gatifloxacin Liposomal Hydrogel for Enhanced Transcorneal Permeation,” Jour- nal of Liposome Research, Vol. 20, No. 1, 2010, pp. 31- 37. http://dx.doi.org/10.3109/08982100903030255 [29] K. M. Hosny, “Ciprofloxacin as Ocular Liposomal Hy- drogel,” AAPS PharmSciTech, Vol. 11, No. 1, 2010, pp. 241-246. http://dx.doi.org/10.1208/s12249-009-9373-4 [30] F. S. Habib, E. A. Fouad, M. S. Abdel-Rhaman, et al., “Liposomes as an Ocular Delivery System of Fluconazole: In-Vitro Studies,” Acta Ophthalmologica, Vol. 88, No. 8, 2010, pp. 901-904. http://dx.doi.org/10.1111/j.1755-3768.2009.01584.x [31] O. N. El-Gazayerly and A. H. Hikal, “Preparation and Evaluation of Acetazolamide Liposomes as an Ocular Delivery System,” International Journal of Pharmaceu- tics, Vol. 158, No. 2, 1997, pp. 121-127. http://dx.doi.org/10.1016/S0378-5173(97)00186-5 [32] D. Aggarwal, A. Garg and I. P. Kaur, “Development of a Topical Niosomal Preparation of Acetazolamide: Prepa- ration and Evaluation,” Journal of Pharmacy and Phar- macology, Vol. 56, No. 12, 2004, pp. 1509-1517. http://dx.doi.org/10.1211/0022357044896 [33] A. S. Guinedi, N. D. Mortada, S. Mansour, et al., “Prepa- ration and Evaluation of Reverse-Phase Evaporation and Multilamellar Niosomes as Ophthalmic Carriers of Aceta- zolamide,” International Journal of Pharmaceutics, Vol. 306, No. 1-2, 2005, pp. 71-82. http://dx.doi.org/10.1016/j.ijpharm.2005.09.023 [34] R. M. Hathout, S. Mansour, N. D. Mortada, et al., “Lipo- somes as an Ocular Delivery System for Acetazolamide: in Vitro and in Vivo Studies,” AAPS PharmSciTech, Vol. 8, No. 1, 2007, pp. E1-E12. http://dx.doi.org/10.1208/pt0801001 [35] S. P. Vyas, N. Mysore, V. Jaitely, et al., “Discoidal Nio- some Based Controlled Ocular Delivery of Timolol Ma- leate,” Pharmazie, Vol. 53, No. 7, 1998, pp. 466-469. [36] I. P. Kaur, D. Aggarwal, H. Singh and S. Kakkar, “Im- proved Ocular Absorption Kinetics of Timolol Maleate Loaded into a Bioadhesive Niosomal Delivery System,” Graefe’s Archive for Clinical and Experimental Oph- thalmology, Vol. 248, No. 10, 2010, pp. 1467-1472. http://dx.doi.org/10.1007/s00417-010-1383-0 [37] P. Prabhu, K. R. Nitish, M. Koland, N. Harish, K. Vijayanarayan, G. Dhondge and R. Charyulu, “Prepara- tion and Evaluation of Nano-Vesicles of Brimonidine Tartrate as an Ocular Drug Delivery System,” Journal of Young Pharmacists, Vol.2, No. 4, 2010, pp. 356-361. http://dx.doi.org/10.4103/0975-1483.71623 [38] S Maiti, S Paul, R Mondol, S. Ray and B. Sa, “Nanove- sicular formulation of Brimonidine Tartrate for the Man- agement of Glaucoma: In Vitro and in Vivo Evaluation,” AAPS PharmSciTech, Vol.12, No. 2, 2011, pp. 755-763. http://dx.doi.org/10.1208/s12249-011-9643-9 Open Access OJOph
 Nanobiotechnology in the Management of Glaucoma 132 [39] M. Sabyasachi, P. Sayon, M. Ranjit, R. Somasree and S. Biawanath, “Nanovesicular Formulation of Brimonidine Tartrate for the Management of Glaucoma: In Vitro and in Vivo Evaluation,” AAPS PharmSciTech, Vol. 12, No. 2, 2011, pp. 755-763. http://dx.doi.org/10.1208/s12249-011-9643-9 [40] C. Sturesson, J. Carlfors, K. Edsman and M. Andersson, “Preparation of Biodegradable Poly(lactic-co-glycolic) Acid Microspheres and Their in Vitro Release of Timolol Maleate,” International Journal of Pharmaceutics, Vol. 89, No. 3, 1993, pp. 235-244. http://dx.doi.org/10.1016/0378-5173(93)90249-F [41] J. P. Bertram, S.S. Saluja, J. McKain and E. B. Lavik, “Sustained Delivery of Timolol Maleate from Poly(lac- tic-co-glycolic acid)/Poly(lactic acid) Microspheres for over 3 Months,” Journal of Microencapsulation, Vol. 26, No. 1, 2009, pp. 18-26. http://dx.doi.org/10.1080/02652040802095250 [42] G. G. Giordano, P. Chevez-Barrios, M. F. Refojo and C. A. Garcia, “Biodegradation and Tissue Reaction to In- travitreous BiodeGradable Poly(D,L-lactic-co-glycolic)- Acid Microsphere,” Current Eye Research, Vol. 14, No. 9, 1995, pp. 761-768. http://dx.doi.org/10.3109/02713689508995797 [43] R. Chen, Y. Qian, R Li,Q. Zhang, D. F. Liu, M. Wang and Q. W. Xu, “Methazolamide Calcium Phosphate Nano- particles in An Ocular Deli very Sy stem,” Yakugaku Zasshi, Vol. 130, No. 3, 2010, pp. 419-424. http://dx.doi.org/10.1248/yakushi.130.419 [44] R. Li, S. Jiang, D. Liu, X. Y. Bi, F. Z. Wang, Q. Zhang and Q. W. Xu, “A Potential New Therapeutic System for Glaucoma: Solid Lipid Nanoparticles Containing Metha- zolamide,” Journal of Microencapsulation, Vol. 28, No. 2, 2011, pp. 134-141. http://dx.doi.org/10.3109/02652048.2010.539304 [45] S. K. Motwani, S. Chopra, S. Talegaonkar, K. Kohli, F. J. Ahmad and R. K. Khar, “Chitosan-Sodium Alginate Nanoparticles as Submicroscopic Reservoirs for Ocular Delivery: Formulation, Optimisation and in Vitro Char- acterization,” European Journal of Pharmaceutics and Biopharmaceutics, Vol. 68, No. 3, 2008, pp. 513-525. [46] X. B. Yuan, Y. B. Yuan, W. Jiang, et al., “Preparation of Rapamycin-Loaded Chitosan/PLA Nanoparticles for Im- munosuppression in Corneal Transplantation,” Interna- tional Journal of Pharmaceutics, Vol. 349, No. 1-2, 2008, pp. 241-248. http://dx.doi.org/10.1016/j.ijpharm.2007.07.045 [47] S. Wadhwa, R. Paliwal, S. R. Paliwal and S. P. Vyas, “Hyaluronic Acid Modified Chitosan Nanoparticles for Effective Management of Glaucoma: Development, Cha- racterization, and Evaluation,” Journal of Drug Targeting, Vol. 18, No. 4, 2010, pp. 292-302. http://dx.doi.org/10.3109/10611860903450023 [48] R. J. Marano, N. Wimmer, P. S. Kearns, B. G. Thomas, I. Toth, M. Brankov and P. E. Rakoczy, “Inhibition of in Vitro VEGF Expression and Choroidal Neovasculariza- tion by Synthetic Dendrimer Peptide Mediated Delivery of a Sense Oligonucle otide,” Experimental Eye Research, Vol. 79, No. 4, 2004, pp. 525-535. http://dx.doi.org/10.1016/j.exer.2004.06.023 [49] R. Ideta, F. Tasaka, W. D. Jang, et al., Nanotechnology- Based Photodynamic Therapy for Neovascular Disease using a Supramolecular Nanocarrier Loaded with a Den- Dritic Photosensitizer,” Nano Letters, Vol. 5, No. 12, 2005, pp. 2426-2431. http://dx.doi.org/10.1021/nl051679d [50] K. Sugisaki, T. Usui, N. Nishiyama, W. D. Jang, Y. S. Yanagi, S. Yamagami, S. Amano and K. Kataoka, “Pho- todyna mic Ther apy for Cornea l Neovascularization Using Polymeric Micelles Encapsulating Dendrimer Porphy- rins,” Investigative Ophthalmology & Visual Science, Vol. 49, No. 3, 2008, pp. 894-899. http://dx.doi.org/10.1167/iovs.07-0389 [51] Th. F. Vandamme and L. Brobeck, “Poly(amidoamine) Dendrimers as Ophthalmic Vehicles for Ocular Delivery of Pilocarpine Nitrate and Tropicamide,” Journal of Con- trolled Release, Vol. 102, No. 1, 2005, pp. 23-38. http://dx.doi.org/10.1016/j.jconrel.2004.09.015 [52] C. A. Holden, P. Tyagi, A. Thakur, R. Kadam, G. Jadhav, U. B. Kompella and H. Yang, “Polyamidoamine Den- drimer Hydrogel for Enhanced Delivery of Antiglaucoma Drugs,” Nanomedicine, Vol. 8, No. 5, 2012, pp. 776-783. [53] H. A. Quigley, R. W. Nickells, L. A. Kerrigan, M. E. Pease, D. J. Thibault and D. J. Zack, “Retinal Ganglion Cell Death in Experimental Glaucoma and after Axotomy Occurs by Apoptosis,” Investigative Ophthalmology & Visual Science, Vol. 36, No. 5, 1995, pp. 774-786. [54] J. Weise, S. Isenmann, N. Klöcker, S. Kugler, S. Hirsch, C. Gravel and M. Bahr, “Adenovirus-Mediated Expres- sion of Ciliary Neurotrophic Factor (CNTF) Rescues Axotomized Rat Retinal Ganglion Cells but Does Not Support Axonal Regeneration in Vivo,” Neurobiology of Disease, Vol. 7, No. 3, 2000, pp. 212-223. http://dx.doi.org/10.1006/nbdi.2000.0285 [55] G. Parrilla-Reverter, M. Agudo, P. Sobrado-Calvo, et al., “Effects of Different Neurotrophic Factors on the Sur- vival of Retinal Ganglion Cells after a Complete Intraor- bital Nerve Crush Injury: A Quantitative in Vivo Study,” Experimental Eye Research, Vol. 89, No. 1, 2009, pp. 32-41. http://dx.doi.org/10.1016/j.exer.2009.02.015 [56] M. D. Pease, D. J. Zack, C. Berlinicke, et al., “Effect of CNTF on Retinal Ganglion Cell Survival in Experimental Glaucoma,” Investigative Ophthalmology & Visual Sci- ence, Vol. 50, No. 5, 2009, pp. 2194-2200. http://dx.doi.org/10.1167/iovs.08-3013 [57] Y. Hu, S. G. Leaver, G. W. Plant, W. T. J. He ndriks, S. P. Niclou, J. Verhaagen, A. R. Harvey and Q. Cui, “Lenti- viral-Mediated Transfer of CNTF to Schwann Cells within Reconstructed Peripheral Nerve Grafts Enhances Adult Retinal Ganglion Cell Survival and Axonal Regen- eration,” Molecular Therapy, Vol. 11, No. 6, 2005, pp. 906-915. http://dx.doi.org/10.1016/j.ymthe.2005.01.016 [58] M. K. Nkansah, S. Y. Tzeng, A. M. Holdt and E. B. Lavik, “Poly(lactic-co-glycolic acid) Nanospheres and Micro- spheres for Short and Long-Term Delivery of Bioactive Ciliary Neurotrophic Factor,” Biotechnology and Bioeng- ineering, Vol. 100, No. 5, 2008, pp. 1010-1019. http://dx.doi.org/10.1002/bit.21822 [59] S. Y. Tzeng and E. B. Lavik, “Photopolymerizable Na- noarray Hydrogels Deliver CNTF and Promote Differen- Open Access OJOph
 Nanobiotechnology in the Management of Glaucoma Open Access OJOph 133 tiation of Neural Stem Cells,” Soft Matter, Vol. 6, No. 10, 2010, pp. 2208-2215. http://dx.doi.org/10.1039/b923544b [60] M. Jeun, J. W. Jeoung, S. Moon, et al., “Engineered Su- perparamagnetic Mn0.5Zn0.5Fe2O4 Nanoparticles as a Heat Shock Protein Induction Agent for Ocular Neuroprotec- tion in Glaucoma,” Biomaterials, Vol. 32, No. 2, 2011, pp. 387-394. http://dx.doi.org/10.1016/j.biomaterials.2010.09.016 [61] G. L. Skuta and R. K. Parrish 2nd, “Wound Healing in Glaucoma Filtering Surgery,” Survey of Ophthalmology, Vol. 32, No. 3, 1987, pp. 149-170. [62] M. M. Tahery and D. A. Lee, “Pharmacologic Control of Wound Healing in Glaucoma Filtration Surgery,” Journal of Ocular Pharmacology and Therapeutics, Vol. 5, No. 2, 1989, pp. 155-179. http://dx.doi.org/10.1089/jop.1989.5.155 [63] D. A. Morris, M. O. Peracha, D. H. Shin, C. Kim, S. C. Cha and Y. Y. Kim, “Risk Factors for Early Filtration Failure Requiring Suture Release after Primary Glau- coma Triple Procedure with Adjunctive Mitomycin,” JAMA Ophthalmology, Vol. 117, No. 9, 1999, pp. 1149- 1154. http://dx.doi.org/10.1001/archopht.117.9.1149 [64] P. W. DeBry, T. W. Pe rkins, G. Hea tley , P. Ka ufman, ; L. C. Brumback, “Incidence of Late-Onset Bleb-Related Complications Following Trabeculectomy with Mitomy- cin,” JAMA Ophthalmology, Vol. 120, No. 3, 2002, pp. 297-300. http://dx.doi.org/10.1001/archopht.120.3.297 [65] A. M. Palanca-Capistrano, J. Hall, L. B. Cantor, L. Mor- qan, J. Hoop and D. Wudunn, “Long-Term Outcomes of Intraoperative 5-Fluorouracil versus Intraoperative Mito- mycin C in Primary Trabeculectomy Surgery,” Ophthal- mology, Vol. 116, No. 2, 2009, pp. 185-190. http://dx.doi.org/10.1016/j.ophtha.2008.08.009 [66] L. J. Cui, N. X. Sun, X. H. Li, J. Huang and J. G.Yang, “Subconjunctival Sustained Release 5-Fluorouracil for Glaucoma Filtration Surgery,” Acta Pharmacologica Si- nica, Vol. 29, No. 9, 2008, pp. 1021-1028. http://dx.doi.org/10.1111/j.1745-7254.2008.00833.x [67] Z. Hou, H. Wei, Q. Wang, et al., “New Method to Pre- pare Mitomycin C Loaded PLA-Nanoparticles with High Drug Entrapment Efficiency,” Nanoscale Research Let- ters, Vol. 4, No. 7, 2009, pp. 732-737. http://dx.doi.org/10.1007/s11671-009-9312-z [68] A. E. Yassin, M. K. Anwer, H. A. Mowafy, I. M. El- Bagory, M. A. Bayomi and I. A. Alsarra, “Optimization of 5-Fluorouracil Solid-Lipid Nanoparticles: A Prelimi- nary Study to Treat Colon Cancer,” International Journal of Medical Sciences, Vol.7, No. 6, 2010, pp. 398-408. http://dx.doi.org/10.7150/ijms.7.398 [69] A. J. Shuhendler, R. Y. Cheung, J. Manias, A. Connor, A. M. Rauth and X. Y. Wu, “A Novel Doxorubicin-Mitop- mycin C Co-Encapsulated Nano-Particle Formulation Ex- hibits Anti-Cancer Synergy in Multidrug Resistant Hu- man Breast Cancer Cells,” Breast Cancer Research and Treatment, Vol. 119, No. 2, 2010, pp. 255-269. http://dx.doi.org/10.1007/s10549-008-0271-3 [70] S. Shaunak, S. Thomas, E. Gianasi, et al., “Polyvalent Dendrimer Glucosamine Conjugates Prevent Scar Tissue Formation,” Nature Biotechnology, Vol. 22, No. 8, 2004, pp. 977-984. [71] A. L. Gomes dos Santos, A Bochot, A Doyle, et al., “Sus- tained Release of Nanosized Complexes of Polyethylen- imine and Anti-TGF-[beta]2 Oligonucleotide Improves the Outcome of Glaucoma Surgery,” Journal of Con- trolled Release, Vol. 112, No. 3, 2006, pp. 369-381. [72] C. Gómez-Gaete, N. Tsapis, M. Besnard, A. Bochot and E. Fattal, “Encapsulation of Dexamethasone into Biode- gradable Polymeric Nanoparticles,” International Jour- nal of Pharmaceutics, Vol. 331, No. 2, 2007, pp. 153-159. http://dx.doi.org/10.1016/j.ijpharm.2006.11.028 [73] T. W. Perkins, B. Faha , M. Ni, et al., “Adenovirus-Medi- ated Gene Therapy Using Human p21waf-1/cip-1 to pre- vent Wound Healing in a Rabbit Model of Glaucoma Fil- tration Surgery,” JAMA Ophthalmology, Vol. 120, No. 7, 2002, pp. 941-949. http://dx.doi.org/10.1001/archopht.120.7.941 [74] J. G. Yang, N. X. Sun, L. J. Cui, X. H. Wang and Z. H. Feng, “Adenovirus-Mediated Delivery of p27KIP1 to Prevent Wound Healing after Experimental Glaucoma Filtration Surgery,” Acta Pharmacologica Sinica, Vol. 30, No. 4, 2009, pp. 413-423. [75] M. G. Spiga and T. Borrás, “Development of a Gene Therapy Virus with a Glucocorticoid-Inducible MMP1 for the Treatment of Steroid Glaucoma,” Investigative Ophthalmology & Visual Science, Vol. 51, No. 6, 2010, pp. 3029-3041. http://dx.doi.org/10.1167/iovs.09-4918
|