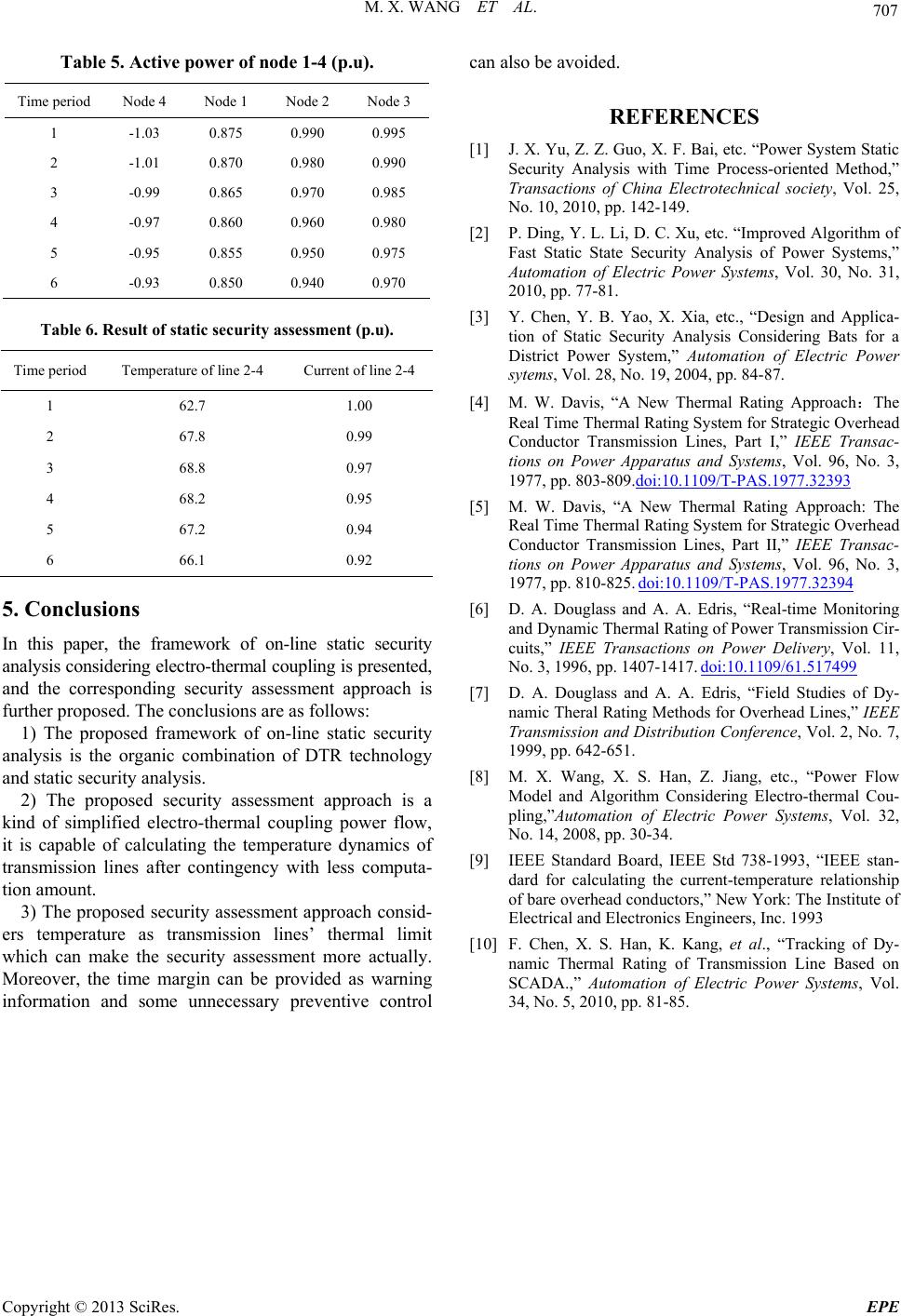
M. X. WANG ET AL.
Copyright © 2013 SciRes. EPE
707
Table 5. Active power of node 1-4 (p.u).
Time period Node 4 Node 1 Node 2 Node 3
1 -1.03 0.875 0.990 0.995
2 -1.01 0.870 0.980 0.990
3 -0.99 0.865 0.970 0.985
4 -0.97 0.860 0.960 0.980
5 -0.95 0.855 0.950 0.975
6 -0.93 0.850 0.940 0.970
Table 6. Result of static security assessment (p.u).
Time period Temperature of line 2-4 Current of line 2- 4
1 62.7 1.00
2 67.8 0.99
3 68.8 0.97
4 68.2 0.95
5 67.2 0.94
6 66.1 0.92
5. Conclusions
In this paper, the framework of on-line static security
analysis considering electro-ther mal coupling is presented,
and the corresponding security assessment approach is
further proposed. The conclusions are as follows:
1) The proposed framework of on-line static security
analysis is the organic combination of DTR technology
and static security analysis.
2) The proposed security assessment approach is a
kind of simplified electro-thermal coupling power flow,
it is capable of calculating the temperature dynamics of
transmission lines after contingency with less computa-
tion amount.
3) The proposed security assessment approach consid-
ers temperature as transmission lines’ thermal limit
which can make the security assessment more actually.
Moreover, the time margin can be provided as warning
information and some unnecessary preventive control
can also be avoided.
REFERENCES
[1] J. X. Yu, Z. Z. Guo, X. F. Bai, etc. “Power System Static
Security Analysis with Time Process-oriented Method,”
Transactions of China Electrotechnical society, Vol. 25,
No. 10, 2010, pp. 142-149.
[2] P. Ding, Y. L. Li, D. C. Xu, etc. “Improved Algorithm of
Fast Static State Security Analysis of Power Systems,”
Automation of Electric Power Systems, Vol. 30, No. 31,
2010, pp. 77-81.
[3] Y. Chen, Y. B. Yao, X. Xia, etc., “Design and Applica-
tion of Static Security Analysis Considering Bats for a
District Power System,” Automation of Electric Power
sytems, Vol. 28, No. 19, 2004, pp. 84-87.
[4] M. W. Davis, “A New Thermal Rating Approach:The
Real Time Thermal Rating System for Strategic Overhead
Conductor Transmission Lines, Part I,” IEEE Transac-
tions on Power Apparatus and Systems, Vol. 96, No. 3,
1977, pp. 803-809.doi:10.1109/T-PAS.1977.32393
[5] M. W. Davis, “A New Thermal Rating Approach: The
Real Time Thermal Rating System for Strategic Overhead
Conductor Transmission Lines, Part II,” IEEE Transac-
tions on Power Apparatus and Systems, Vol. 96, No. 3,
1977, pp. 810-825. doi:10.1109/T-PAS.1977.32394
[6] D. A. Douglass and A. A. Edris, “Real-time Monitoring
and Dynamic Thermal Rating of Power Transmission Cir-
cuits,” IEEE Transactions on Power Delivery, Vol. 11,
No. 3, 1996, pp. 1407-1417. doi:10.1109/61.517499
[7] D. A. Douglass and A. A. Edris, “Field Studies of Dy-
namic Theral Rating Methods for Overhead Lines,” IEEE
Transmission and Distribution Conference, Vol. 2, No. 7,
1999, pp. 642-651.
[8] M. X. Wang, X. S. Han, Z. Jiang, etc., “Power Flow
Model and Algorithm Considering Electro-thermal Cou-
pling,”Automation of Electric Power Systems, Vol. 32,
No. 14, 2008, pp. 30-34.
[9] IEEE Standard Board, IEEE Std 738-1993, “IEEE stan-
dard for calculating the current-temperature relationship
of bare overhead conductors,” New York: The Institute of
Electrical and Electronics Engineers, Inc. 1993
[10] F. Chen, X. S. Han, K. Kang, et al., “Tracking of Dy-
namic Thermal Rating of Transmission Line Based on
SCADA.,” Automation of Electric Power Systems, Vol.
34, No. 5, 2010, pp. 81-85.