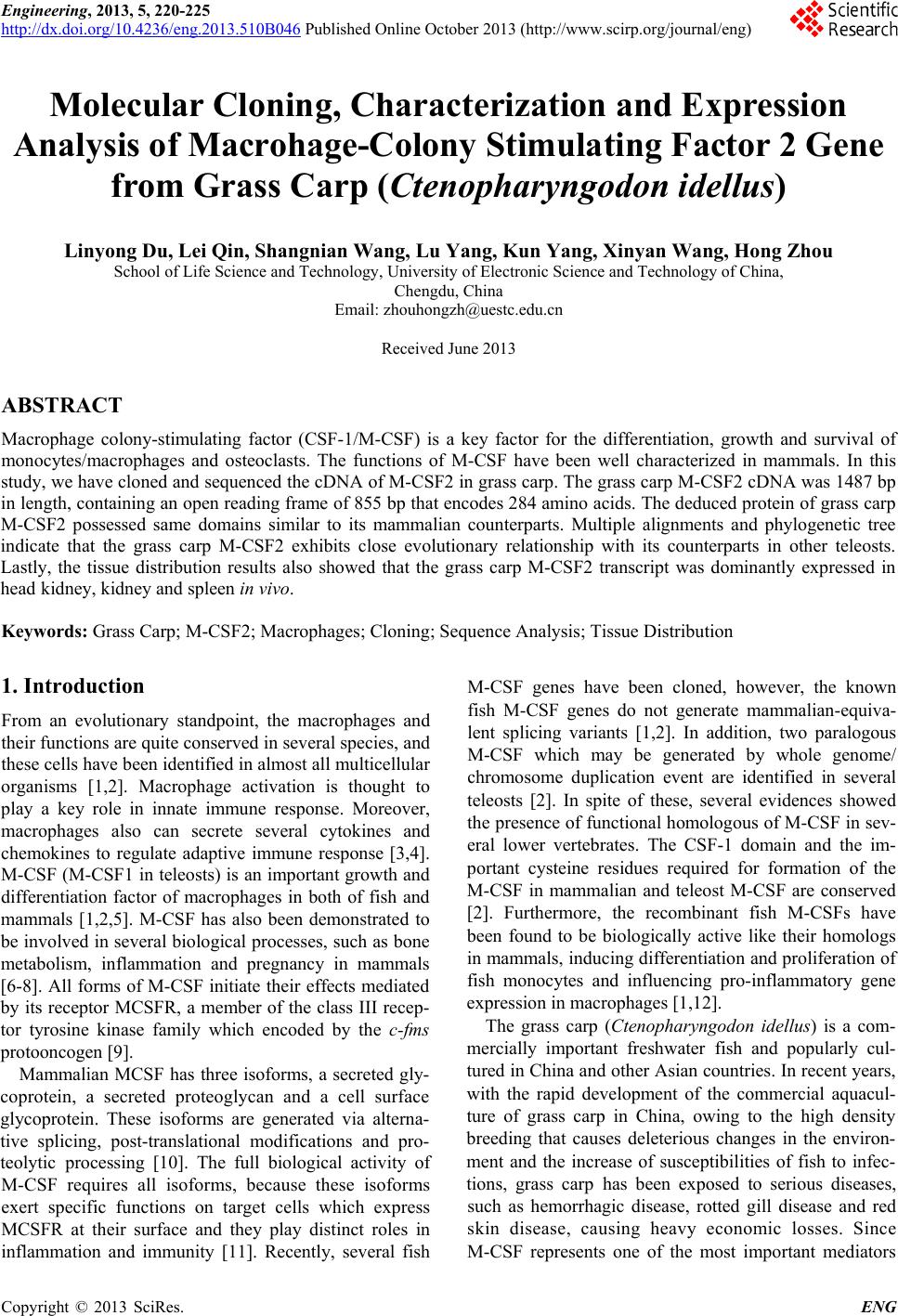 Engineering, 2013, 5, 220-225 http://dx.doi.org/10.4236/eng.2013.510B046 Published Online October 2013 (http://www.scirp.org/journal/eng) Copyright © 2013 SciRes. ENG Molecular Cloning, Characterization and Expression Analysis of Macrohage-Colony Stimulating Factor 2 Gene from Grass Carp (Ctenopharyngodon idellus) Linyong Du, Lei Qin, Shangnian Wang, Lu Yang, Kun Yang, Xinyan Wang, Hong Zho u School of Life Science and Technology, University of Electronic Science and Technology of China, Chengdu, China Email: zhouhongzh@uestc.edu.cn Received June 2013 ABSTRACT Macrophage colony-stimulating factor (CSF-1/M-CSF) is a key factor for the differentiation, growth and survival of monocytes/macrophages and osteoclasts. The functions of M-CSF have been well characterized in mammals. In this study, we have cloned and sequenced the cDNA of M-CSF2 in grass carp. The grass carp M-CSF2 cDNA was 1487 bp in length, containing an open read ing fr ame of 855 bp that encodes 284 amino acids. The deduced protein of grass carp M-CSF2 possessed s ame domains similar to its mammalian counterparts. Multiple alignments and phylogenetic tree indicate that the grass carp M-CSF2 exhibits close evolutionary relationship with its counterparts in other teleosts. Lastly, the tissue distr ibution results also showed that the grass carp M-CSF2 transcript was dominantly expressed in head kidney, kidney and spleen in vivo. Keywords: Grass Carp; M-CSF2; Macrophages; Cloning; Sequence Analysis; Tissue Distribution 1. Introduction From an evolutionary standpoint, the macrophages and their functions are quite conser ved in several species, and these cells have been identified in almos t all multicellular organisms [1,2]. Macrophage activation is thought to play a key role in innate immune response. Moreover, macrophages also can secrete several cytokines and chemokines to regulate adaptive immune response [3,4]. M-CSF (M-CSF1 in teleosts) is an important growth and differentiation factor of macrophages in both of fish and mammals [1,2,5]. M-CSF has also been demonstrated to be involved in several biological processes, such as bone metabolism, inflammation and pregnancy in mammals [6-8]. All fo r ms of M-CSF initiate their effects mediated by its receptor MCSFR, a memb er of the class III recep- tor tyrosine kinase famil y which encoded by the c-fms protooncogen [9]. Mammalian MCSF has three isoforms, a secreted gly- coprotein, a secreted proteoglycan and a cell surface glycoprotein. These isoforms are genera ted via a lterna- tive splicing, post-trans lational modifications and pro- teolytic processing [10]. The full biological activity of M-CSF requ ir es all isof or ms, because these isoforms exert specific functions on target cells which express MCSFR at their surface and they play distinct roles in inflammation and immunity [11]. Recently, several fish M-CSF genes have been cloned, however, the known fish M-CSF genes do not generate mammalian-equiva- lent splicing variants [1,2]. In addition, two paralogous M-CSF which may be generated by whole genome/ chromosome duplication event are identified in several teleosts [2]. In spite of these, several evidences showed the presence of functional homologous of M-CSF in sev- eral low er vertebrates. The CSF-1 d oma in and the im- portant cysteine residues required for formation of the M-CSF in mammalian and teleost M-CSF are conserved [2]. Furthermore, the recombinant fish M-CSFs have been found to be biologically active like their homologs in mammals, inducing differentiation and proliferation of fish monocytes and influencing pro-inflammatory gene expression in macrophages [1,12]. The grass carp (Ctenopharyngodon idellus) is a com- mercially important freshwater fish and popularly cul- tured in China and other Asian countries. In recent years, with the rapid development of the commercial aquacul- ture of grass carp in China, owing to the high density breeding that causes deleterious changes in the environ- ment and the increase of susceptibilities of fish to infec- tions, grass carp has been exposed to serious diseases, such as hemorrhagic disease, rotted gill disease and red skin dise ase, causing heavy economic loss es. Sinc e M-CSF represents one of the most important mediators
 L. Y. DU ET AL. Copyright © 2013 SciRes. ENG of innate immune response to eliminate microorganism infections, the studies on grass carp M-CSF would give a valuable insight into the control of diseases in aquacul- ture industry. In our study, we iso lated grass carp M- CSF2 cDNA using the homology cloning approach, per- formed sequence analysis and phylogeny analysis, and revealed its expression patterns in various organs. 2. Materials and Methods 2.1. Animals One-year-old Chinese grass carp, weighting 0.5 - 0.75 kg, were purchased fro m Chengdu Tongwei Aquatic Science and Technology Company (Chengdu, China). Tissues for expression analysis were obtained from the freshly killed fish according to the Regulation of Animal Use in Si- chuan province, China. 2.2. Total RNA Extraction and cDNA Synthesis Total RNA in the organs for expression analysis was extracted by using Tripure (Roche, USA) according to the manufacturer’s instructions. Five μg of total RNA was subjected to reverse transcription by using Oligo (dT)18 as the prime r with M-MLV reverse transcriptase (Promega, Madison, MI) and s tored the cDNA at −80˚C. 2.3. Cloning of Grass Carp M-CSF2 cDNA Partial sequences of grass carp M-CSF2 was identified by homology-based PCR using other known teleost M-CSF2 sequences as references, the degenerated pri- mers were shown in Table 1. The PCR fragments were sequenced. Based on the obtained fragment, RACE was performed with gene specific primers (Table 1) follow- ing the manufacturer’s instructions of GeneRacer Kit (Life Technologies, Shanghai, China). The PCR frag- ments was s equenced and assembled. Finally, we vali- dated the full-length coding sequences (CDSs) of grass carp M-CSF2 with Pfu DNA polymerase (Promega). 2.4. Sequence Analysis and Phylogeny Analysis The open reading frames and deduced protein sequences of grass carp M-CSF2 was identified by ORF finder [13]. The signal peptide was predicted using the SignalP 4.1 program and the transmembrane domain was predicted using the TMpred program [14,15]. Characteristic do- mains of the deduced proteins were predicted by SMART program [16]. The identity analysis by using nucleotide and protein BLAST programs (www.ncbi.nlm.nih.gov/BLAST/). Mul tip le alignments between the deduced amino acid sequence of grass carp M-CSF2 and those of other known species M-CSF was conducted with DNAMAN software (Lynnon Biosoft, Table 1. Primers used for gene cloning and expression analysis. Na me Sequence (5’to 3’) Objects M-CSF2 F CCAAGGGGAAGAATGTGTGTG cloning M-CSF2 R GGGAGTAGATGTTGAGGATGAG M-CSF2 5N1 CCATGCTCAGGTGTTTTCTCTC 5’-RACE M-CSF2 5N2 CATCATGGAAAGGGGAACACACAG M-CSF2 3N1 GAGAGAAAACACCTGAGCATGG 3’-RACE M-CSF2 3N2 GCTTGAACTGTTTAACGTCCACTTC M-CSF2 Realtime F CCTTGCAAACATGCCATAACCAT MCSF2 expression M-CSF2 Realtime R CCATGCTCAGGTGTTTTCTCT B-actin QF AGCCATCCTTCTTGGGTATG β-actin expression B-actin QR GGTGGGGCGATGATCTTGAT Quebec, Canada). Phylogenetic tree was constructed by using MEGA 4 (www.megasoftware.net) based on Neigh- bor-Joining method with the bootstrapping of 1000 repe- titions [17]. The 3D structure model of CSF-1 domain of grass carp M-CSF2 was performed using the SWISS- MODEL program (http:// swissmodel.expasy.org). 2.5. Expression Patterns of M-CSF in Vivo Total RNA was extracted from the thymus, head kidney, liver, spleen, gill, intestine and muscle , the cDNAs were prepared by reverse transcription as described previously. The gene-specific primers for grass carp M-CSF2 were designed (Table 1) and used for detecting its transcript in different tissues by real-time quantitative PCR (qPCR). In our study, qPCR was performed on the Bio-Rad CFX96 Real-time detection system (Bio-Rad Laborato- ries, Inc., Hercules, CA) in a final volume of 20 μl with 10 μl iQTMSYBRTM Green Su pe rmi x (Bio-Rad Labora tories, Inc., Her cules, CA) following the procedures in our previous study. β-actin was used as a internal control. Data were analyzed using the CFX manager and nor ma- lized to β-actin after correcting fo r differences in amp li- fication efficiency. 3. Results 3.1. Molecular Cloning Grass Carp M-CSF2 cDNA The complete cDNA and deduced amin o acid sequences of grass carp M-CSF2 (Genebank ID: KF113858) are shown in Figure 1. The grass carp M-CSF2 cDNA se- quence is 1487 bp in length with a 216 bp 5’-untran- slated region (UT R ), a 416 bp 3’-UTR with the latter containing a cytokine RNA instability moti f (ATTTA) and a polyadenylation signal (AATAAA) nucleotide up-
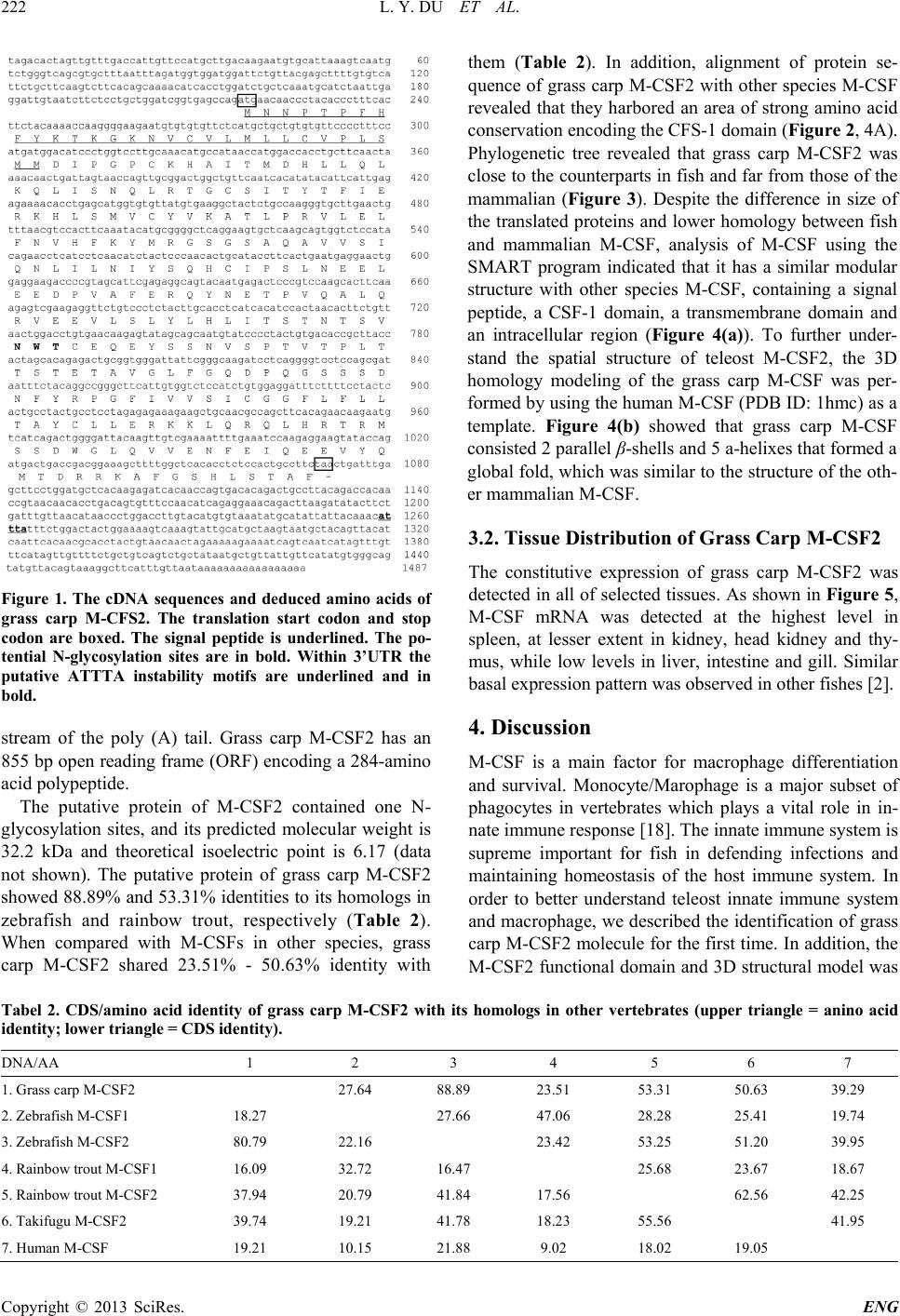 L. Y. DU ET AL. Copyright © 2013 SciRes. ENG Figure 1. The cDNA sequences and deduced amino acids of grass carp M-CFS2. The translation start codon and stop codon are boxed. The signal peptide is underlined. The po- tential N-glycosylation sites are in bold. Within 3’UTR the putative ATTTA instability motifs are underlined and in bold. stream of the poly (A) tail. Grass carp M-CSF2 has an 855 bp open reading f ra me (ORF) encoding a 284-ami no acid polypeptide. The putative protein of M-CSF2 contained one N- glycosylation sites, and its predicted molecular weight is 32.2 kDa and theoretical isoelectric point is 6.17 (data not shown). The putative protein of grass carp M-CSF2 showed 88.89% and 53.31% identities to its homologs in zebrafish and rainbow trout, respectively (Table 2). When compared with M-CSFs in other species, grass carp M-CSF2 shared 23.51% - 50.63% identity with them (Table 2). In addition, alignment of protein se- quence of grass carp M-CSF2 with other species M-CSF revealed that they harbored an area of strong amin o acid conservation encoding the CFS-1 d oma in (Figure 2, 4A). Phylogenetic tree revealed that grass carp M-CSF2 was close to the counterparts in fish and far from those of the mammalian (Figure 3). Despite the difference in size of the translated proteins and lower homology between fish and mammalian M-CSF, analysis of M-CSF using the SMART program indicated that it has a similar modular structure with other species M-CSF, containing a signal peptide, a CSF-1 domain, a tra ns memb r ane do main and an intracellular region (Figure 4(a)). To further under- stand the spatial structure of teleost M-CSF2, the 3D homology modeling of the grass carp M-CSF was per- formed by using the human M-CSF (PDB ID: 1h mc) as a template. Figure 4(b) showed that grass carp M-CSF consisted 2 parallel β-shells and 5 a-helixes that formed a global fold, which was similar to the structure of the oth- er mammal ian M-CSF. 3.2. Tissue Distribution of Grass Ca rp M-CSF2 The constitutive expression of grass carp M-CSF2 was detected in all of selected tissues. As shown in Figure 5, M-CSF mRNA was detected at the highest level in spleen, at lesser extent in kidney, head kidney and thy- mus , while low levels in liver, intestine and gill. Similar basal expression pattern was observed in other fishes [2]. 4. Discussion M-CSF is a main factor for macrophage differentiation and survival. Monocyte/Marophage is a major subset of phagocytes in vertebrates which plays a vital role in in- nate immune response [18]. The innate immune system is supreme important for fish in defending infections and maintaining homeostasis of the host immune system. In order to better understand teleost innate immune system and macrophage, we described the identification of grass carp M-CSF2 molecule for th e first time. In addition, the M-CSF2 functional domain and 3D structural model was Tabel 2. CDS/amino acid identity of grass carp M-CSF2 with its homologs in other vertebrates (upper triangle = anino acid identity; lower tria n g l e = CDS identity). DNA/AA 1 2 3 4 5 6 7 1. Grass carp M-CSF2 27.64 88.89 23.51 53.31 50.63 39.29 2. Zebrafish M-CSF1 18.27 27.66 47.06 28.28 25.41 19.74 3. Zebrafish M-CSF2 80.79 22.16 23.42 53.25 51.20 39.95 4. Rainbow trout M-CSF1 16.09 32.72 16.47 25.68 23.67 18.67 5. Rainbow trout M-CSF2 37.94 20.79 41.84 17.56 62.56 42.25 6. Takifugu M-CSF2 39.74 19.21 41.78 18.23 55.56 41.95 7. Human M-CSF 19.21 10.15 21.88 9.02 18.02 19.05
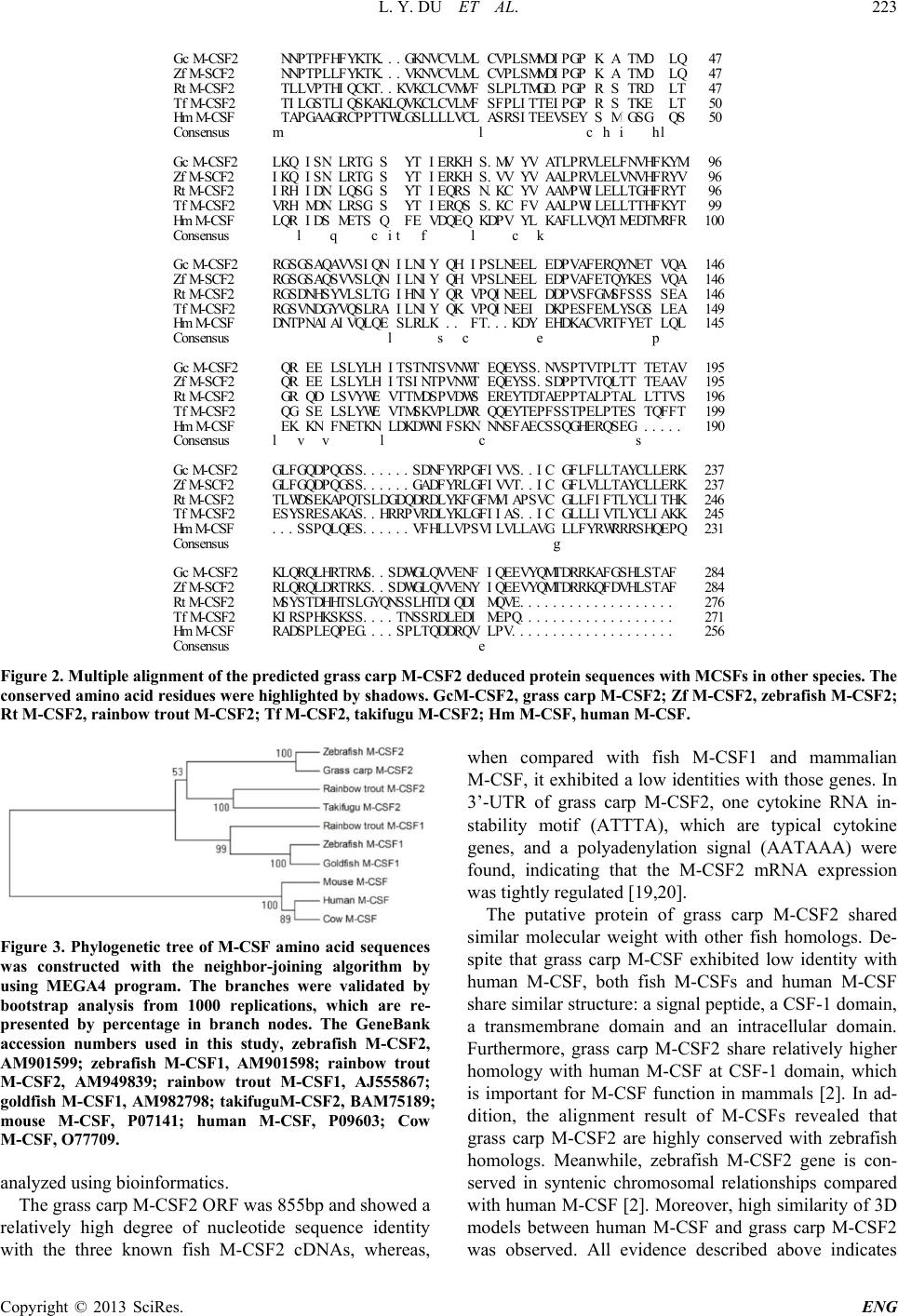 L. Y. DU ET AL. Copyright © 2013 SciRes. ENG Figure 2. Multiple alignment of the predicted grass carp M-CSF2 deduced protein sequences with MCSFs in other species. The conserved amino acid residues were highlighted by shadows. GcM-CSF2, grass carp M-CSF2; Zf M-CSF2, zebr afis h M-CSF2; Rt M-CSF2, rainbow trout M-CSF2; Tf M-CSF2, takifugu M-CSF2; Hm M-CSF, human M-CSF. Figure 3. Phylogenetic tree of M-CSF amino acid sequences was constructed with the neighbor-joining algorithm by using MEGA4 program. The branches were validated by bootstrap analysis from 1000 replications, which are re- presented by percentage in branch nodes. The GeneBank accession numbers used in this study, zebrafish M-CSF2, AM901599; zebrafish M-CSF1, AM901598; rainbow trout M-CSF2, AM949839; rainbow trout M-CSF1, AJ555867; goldfish M-CSF1, AM982798; takifuguM-CSF2, BAM75189; mouse M-CSF, P07141; human M-CSF, P09603; Cow M-CSF, O77709. analyze d using bioinform a tics. The grass carp M-CSF2 ORF was 855bp and showed a relatively high degree of nucleotide sequence identity with the three known fish M-CSF2 cDNAs, whereas, when compared with fish M-CSF1 and mamma li an M-CSF, it exhibited a low identities with those genes. In 3’-UTR of grass carp M-CSF2, one cytokine RNA in- stability motif (ATTTA), which are typical cytokine genes, and a p olyadenylation signal (AATAAA) were found, indicating that the M-CSF2 mR NA expression was tightly regulated [19,20]. The putative protein of grass carp M-CSF2 shared similar molecular weight with other fish homologs. De- spite that grass carp M-CSF exhibited low identity with human M-CSF, both fish M-CSFs and human M-CSF share similar structure: a signal peptide, a CSF-1 domain, a transmembrane domain and an intracellular domain. Furthermore, grass carp M-CSF2 share relatively higher homology with human M-CSF at CSF-1 domain, which is important for M-CSF function in mammals [2]. In ad- dition, the alignment result of M-CS F s r ev ea led th a t grass carp M-CSF2 are highly conserved with zebrafish homologs. Meanwhile, zebrafish M-CSF2 gene is con- served in syntenic chromosomal relationships compared with huma n M-CSF [2]. Moreover, high similarity of 3D models between human M-CSF and grass carp M-CSF2 was observed. All evidence described above indicates 47Gc M-CSF2 47Zf M-SCF2 47Rt M-CSF2 50Tf M-CSF2 50Hm M-CSF Consensus m M M M M M N N T T T N N L I A P P L L P T T V G G P P P S A F L T T A H L H L G F F I I R Y Y Q Q C K K C S P T T K K P K K T A T . . . K T . . . L W . . K Q L G V V V G K K K K S N N C C L V V L L L C C C C L V V V V L L L M L V M M V M C L L F F Ll L L L L L C C S S A V V L F S P P P P R L L L L S S S T I I M M M T T M M G T E D D D E E I I . I V P P P P S G G G G E P P P P Yc C C C C C K K R R Sh H H H H H A A S S M i I I I I I T T T T G M M R K S D D D E Gh H H H H Hl L L L L L L L L L Q Q Q T T S 96Gc M-CSF2 96Zf M-SCF2 96Rt M-CSF2 99Tf M-CSF2 100Hm M-CSF Consensus L I I V L K K R R Q Q Q H H Rl L L L L L I I I M I S S D D D N N N N Sq Q Q Q Q Q L L L L M R R Q R E T T S S T G G G G Sc C C C C C S S S S Qi I I I I It T T T T T Y Y Y Y F T T T T Ef F F F F F I I I I V E E E E D R R Q R Q K K R Q E H H S S Ql L L L L L S S N S K . . . . D M V K K P V V C C Vc C C C C C Y Y Y F Y V V V V Lk K K K K K A A A A K T A A A A L L M L F P P P P L R R W W L V V I I V L L L L Q E E E E Y L L L L I F V L L M N N T T E V V G T D H H H H T F F F F M K R R K R Y Y Y Y F M V T T R 146Gc M-CSF2 146Zf M-SCF2 146Rt M-CSF2 149Tf M-CSF2 145Hm M-CSF Consensus R R R R D G G G G N S S S S T G G D V P S S N N N A A H D A Q Q S G I A S Y Y A V V V V I V V L Q V S S S S Q I L L L L Q Q T R Q N N G A El L L L L L I I I I S L L H L L N N N N R I I I I L Y Y Y Y Ks S S S S S Q Q Q Q . H H R K .c C C C C C I V V V F P P P P T S S Q Q . L L I I . N N N N . E E E E K E E E E D L L L I Ye E E E E E E E D D E D D D K H P P P P D V V V E K A A S S A F F F F C E E G E V R T M M R Q Q S L T Y Y F Y F N K S S Y E E S G E T S S S Tp P P P P P V V S L L Q Q E E Q A A A A L 195Gc M-CSF2 195Zf M-SCF2 196Rt M-CSF2 199Tf M-CSF2 190Hm M-CSF Consensus l L L L L L Q Q G Q E R R R G Kv V V V V V E E Q S K E E D E Nv V V V V V L L L L F S S S S N L L V L E Y Y Y Y T L L W W K H H E E Nl L L L L L I I V V L T T T T D S S T M K T I M S D N N D K W T T S V N S P P P I V V V L F N N D D S W W W W K T T S R Nc C C C C C E E E Q N Q Q R Q N E E E E S Y Y Y Y F S S T T A S S D E E . . T P C N S A F S V D E S S S P P S Q P P P T G T T T P H V V A E E T T L L R P Q P P Q L L T T S T T A E E T T L S Gs S S S S S T T L T . E E T Q . T A T F . A A V F . V V S T . 237Gc M-CSF2 237Zf M-SCF2 246Rt M-CSF2 245Tf M-CSF2 231Hm M-CSF Consensus G G T E . L L L S . F F W Y . G G D S S Q Q S R S D D E E P P P K S Q Q Q A A L G G P K Q S S Q A E S S T S S . . S . . . . L . . . . D H . . . G R . . . D R . . . Q P . S G D V V D A R R F N D D D H F F L L L Y Y Y Y L R R K K V P L F L P G G G G S F F F F V I I M I I V V V I L V V I A V S T A S L . . P . L . . S . A I I V I V C C C C Gg G G G G G G G G G L F F L L L L L L L F F V F L Y L L I I R L L F V W T T T T R A A L L R Y Y Y Y R C C C C S L L L L H L L I I Q E E T A E R R H K P K K K K Q 284Gc M-CSF2 284Zf M-SCF2 276Rt M-CSF2 271Tf M-CSF2 256Hm M-CSF Consensus K R M K R L L S I A Q Q Y R D R R S S S Q Q T P P L L D H L H D H K E R R H S Q T T T K P R R S S E M K L S G S S G . . . . Y . . . . Q . . S S N . . D D S T S W W S N P G G L S L L L H S T Q Q T R Q V V D D D V V I L D E E Q E R N N D D Q F Y I I Ve E E E E E I I M M L Q Q Q E P E E V P V E E E Q . V V . . . Y Y . . . Q Q . . . M M . . . T T . . . D D . . . R R . . . R R . . . K K . . . A Q . . . F F . . . G D . . . S V . . . H H . . . L L . . . S S . . . T T . . . A A . . . F F . . .
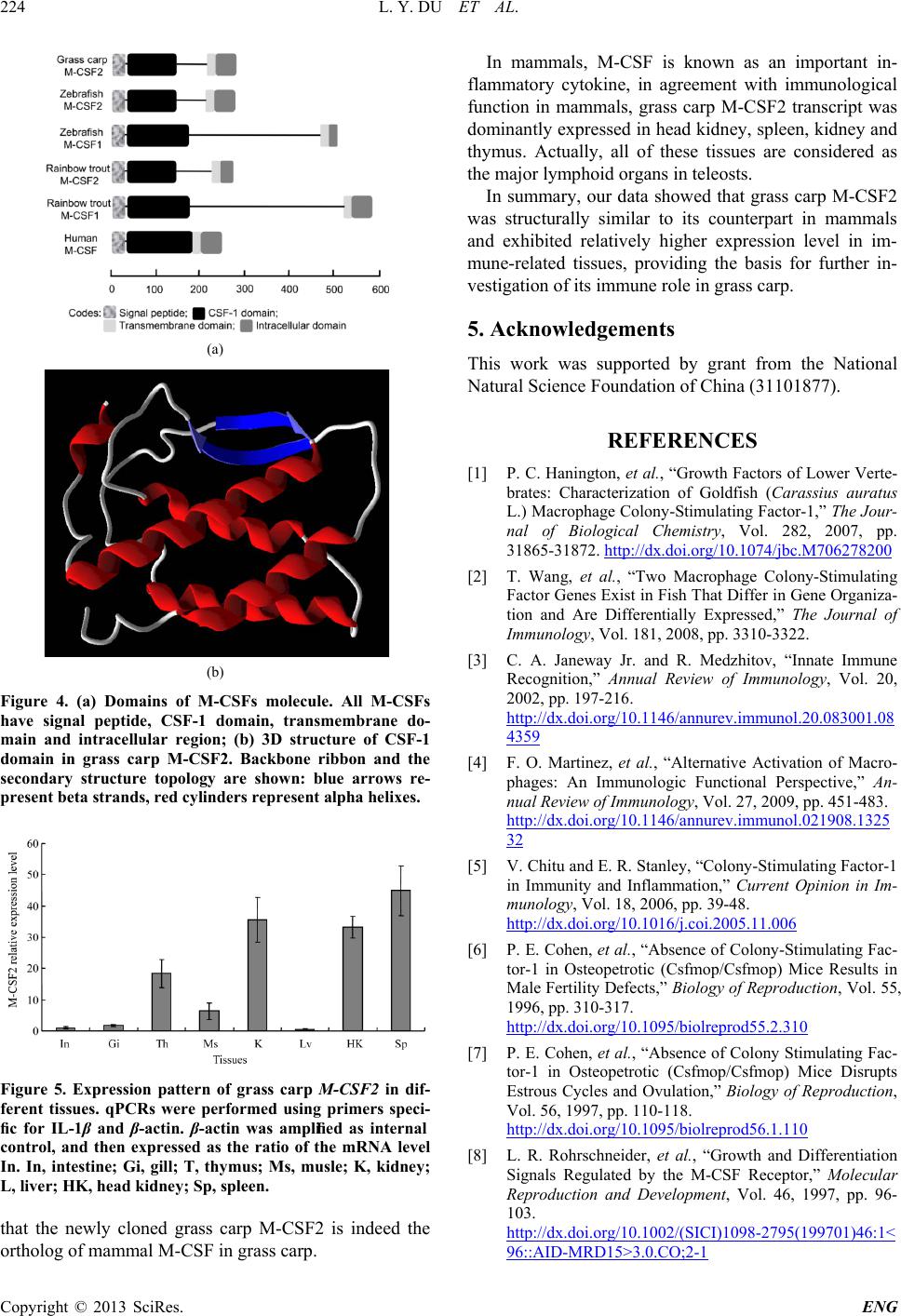 L. Y. DU ET AL. Copyright © 2013 SciRes. ENG (a) (b) Figure 4. (a) Domains of M-CSFs molecule. All M-CSFs have signal peptide, CSF-1 domain, transmembrane do- main and intracellular region; (b) 3D structure of CSF-1 domain in grass carp M-CSF2. Backbone ribbon and the secondary structure topology are shown: blue arrows re- present beta strands, red cylinders represent alpha helixes. Figure 5. Expression pattern of grass carp M-CSF2 in dif- ferent tissues. qPCRs were performed using primers speci- fic for IL-1β and β-actin. β-actin was amplified as internal control, and then expressed as the ratio of the mRNA level In. In, intestine; Gi, gill; T, thymus; Ms, musle; K, kidney; L, liver; HK, head ki dney; Sp, spleen. that the new ly cloned grass carp M-CSF2 is indeed the ortholog of mammal M-CSF in gras s carp. In mammals, M-CS F is known as an impor tant in- flammato ry cytokine, in agreement with immunological function in mammals, grass carp M-CSF2 transcript was dominantly expressed in head kidney, spleen , kidney and thymus. Actually, all of these tissues are considered as the major lymphoid organs in teleosts. In summa ry , our data showed that grass carp M-CSF2 was structurally similar to its counterpart in mammals and exhibited relatively higher expression level in im- mune-related tissues, providing the basis for further in- vestigation of its immu n e role in grass carp. 5. Acknowledgements This work was supported by grant from the National Natural Science Foundation of China (31101877). REFERENCES [1] P. C. Hanington, et al., “Growth Factors of Lower Verte- brates: Characterization of Goldfish (Carassius auratus L.) Macrophage Colony-Stimula ting Factor-1,” The Jour- nal of Biological Chemistry, Vol. 282, 2007, pp. 31865-31872. http://dx.doi.org/10.1074/jbc.M706278200 [2] T. Wang, et al., “Two Macrophage Colony-Stimulating Factor Genes Exist in Fish That Differ in Gene Organiza- tion and Are Differentially Expressed,” The Journal of Immunology, Vol. 181, 2008, pp. 3310-3322. [3] C. A. Janeway Jr. and R. Medzhitov, “Innate Immune Recognition,” Annual Review of Immunology, Vol. 20, 2002, pp. 197-216. http://dx.doi.org/10.1146/annurev.immunol.20.083001.08 4359 [4] F. O. Martinez, et al., “Alternative Activation of Macro- phages: An Immunologic Functional Perspective,” An- nual Review of Immunology, Vol. 27, 2009, pp. 451-483. http://dx.doi.org/10.1146/annurev.immunol.021908.1325 32 [5] V. Chitu and E. R. Stanley, “Colony-Stimulating Factor-1 in Immunity and Inflammation,” Current Opinion in Im- munology, Vol. 18, 2006, pp. 39-48. http://dx.doi.org/10.1016/j.coi.2005.11.006 [6] P. E. Cohen, et al., “Absence of Colony-Stimulating Fac- tor-1 in Osteopetrotic (Csfmop/Csfmop) Mice Results in Male Fe rtility Defects,” Biology of Reproduction, Vol. 55, 1996, pp. 310-317. http://dx.doi.org/10.1095/biolreprod55.2.310 [7] P. E. Cohen, et al., “Absence of Colony Stimulating Fac- tor-1 in Osteopetrotic (Csfmop/Csfmop) Mice Disrupts Estrous Cycles and Ovulation,” Biology of Reproduction, Vol. 56, 1997, pp. 110-118. http://dx.doi.org/10.1095/biolreprod56.1.110 [8] L. R. Rohrschneider, et al., “Growth and Differentiation Signals Regulated by the M-CSF Receptor,” Molecular Reproduction and Development, Vol. 46, 1997, pp. 96- 103. http://dx.doi.org/10.1002/(SICI)1098-2795(199701)46:1< 96::AID-MRD15>3.0.CO;2-1
 L. Y. DU ET AL. Copyright © 2013 SciRes. ENG [9] X. M. Dai, et al., “Targeted Disruption of the Mouse Colony-Stimulating Factor 1 Receptor Gene Results in Osteopetrosis, Mononuclear Phagocyte Deficiency, In- creased Primitive Progenitor Cell Frequencies, and Re- productive Defects,” Blood, Vol. 99, 2002, pp. 111-120. http://dx.doi.org/10.1182/blood.V99.1.111 [10] P. Fixe and V. Praloran, “M-CSF: Haematopoietic Growth Factor or Inflammatory Cytokine?” Cytokine, Vol. 10, 1998, pp. 32-37. http://dx.doi.org/10.1006/cyto.1997.0249 [11] M. J. Sweet and D. A. Hume, “CSF-1 as a Regulator of Macrophage Activation and Immune Responses,” Archi- vum Immunologiae et Therapia Experimentalis (Warsz), Vol. 51, 2003, pp. 169-177. [12] L. Grayfer, et al., “Macrophage Colony-Stimulating Fac- tor (CSF-1) Induces Pro-Inflammatory Gene Expression and Enhances Antimicrobial Responses of Goldfish (Ca- rassius auratus L.) Macrophages,” Fish and Shellfish Im- munology, Vol. 26, 2009, pp. 406-413. http://dx.doi.org/10.1016/j.fsi.2008.12.001 [13] I. T. Rombel, et al., “ORF-FINDER: A Vector for High- Throughput Gene Identification,” Gene, Vol. 282, 2002, pp. 33-41. http://dx.doi.org/10.1016/S0378-1119(01)00819-8 [14] J. D. Bendtsen, et al., “Improved Prediction of Signal Peptides: SignalP 3.0,” Journal of Molecular Biology, Vol. 340, 2004, pp. 783-795. http://dx.doi.org/10.1016/S0378-1119(01)00819-8 [15] M. Ikeda, et al., “Transmembrane Topology Prediction Methods: A Re-Assessment and Improvement by a Con- sensus Method Using a Dataset of Experimentally-Cha- racterized Transmembrane Topologies,” In Silico Biology, Vol. 2, 2002, pp. 19-33. [16] J. Schultz, et al., “SMART, a Simple Modular Architec- ture Research Tool: Identification of Signaling Domains,” Proceedings of the National Academy of Sciences of the United States of America, Vol. 95, 1997, pp. 5857-5864. http://dx.doi.org/10.1073/pnas.95.11.5857 [17] K. Tamura, et al., “MEGA4: Molecular Evolutionary Genetics Analysis (MEGA) Software Version 4.0,” Mo- lecular Biology and Evolution, Vol. 24, 2007, pp. 1596- 1599. http://dx.doi.org/10.1093/molbev/msm092 [18] D. A. Hume, et al., “The Mononuclear Phagocyte System Revisited,” Journal of Leukocyte Biology, Vol. 72, pp. 621-627. [19] A. Bonnieu, et al., “AUUUA Motifs Are Dispe nsable for Rapid Degradation of the Mouse c-myc RN A,” Oncogene, Vol. 5, 1990, pp. 1585-1588. [20] S. Savant-Bhonsale and D. W. Cleveland, “Evidence for Instability of mRNAs Containing AUUUA Motifs Me- diated through Translation-Dependent Assembly of a > 20S Degradation Complex,” Genes & Development, Vol. 6, 1992, pp. 1927-1939. http://dx.doi.org/10.1101/gad.6.10.1927
|