 Journal of Cancer Therapy, 2013, 4, 1341-1354 http://dx.doi.org/10.4236/jct.2013.48159 Published Online October 2013 (http://www.scirp.org/journal/jct) 1341 Cks1: Structure, Emerging Roles and Implications in Multiple Cancers Vinayak Khattar1, Jaideep V. Thottassery1,2 1Southern Research Institute, Birmingham, USA; 2University of Alabama Comprehensive Cancer Center, Birmingham, USA. Email: thottassery@sri.org Received August 12th, 2013; revised September 10th, 2013; accepted September 17th, 2013 Copyright © 2013 Vinayak Khattar, Jaideep V. Thottassery. This is an open access article distributed under the Creative Commons Attribution License, which permits unrestricted use, distribution, and reproduction in any medium, provided the original work is properly cited. ABSTRACT Deregulation of the cell cycle results in loss of normal control mechanisms that prevent aberrant cell proliferation and cancer progression. Regulation of the cell cycle is a highly complex process with many layers of control. One of these mechanisms involves timely degradation of CDK inhibitors (CKIs) like p27Kip1 by the ubiquitin proteasomal system (UPS). Cks1 is a 9 kDa protein which is frequently overexpressed in different tumor subtypes, and has pleiotropic roles in cell cycle progression, many of which remain to be fully characterized. One well characterized molecular role of Cks1 is that of an essential adaptor that regulates p27Kip1 abundance by facilitating its interaction with the SCF-Skp2 E3 ligase which appends ubiquitin to p27Kip1 and targets it for degradation through the UPS. In addition, emerging research has uncovered p27Kip1-independent roles of Cks1 which have provided crucial insights into how it may be involved in cancer progression. We review here the structural features of Cks1 and their functional implications, and also some re- cently identified Cks1 roles and their involvement in breast and other cancers. Keywords: Cks1; Cks2; Skp2; Cdk1; p27; Kip1; p130; Rb2; CKI; Ubiquitination; Proteasome; ERK1/2 1. Introduction Regulation of the cell cycle is crucial for cellular prolif- eration and survival [1,2]. The engines that drive the cell cycle include cyclin dependent kinases (CDKs) and their activating cyclin subunits which oscillate during the cell cycle and thus modulate the activity of CDKs at specific points in various cell cycle phases [3]. Another level of control requires the action of cyclin dependent kinase inhibitors (CKIs) on CDKs [4]. These CKIs, along with other cell cycle substrates are regulated by precise and coordinated proteasomal degradation [5,6]. This in turn can regulate the activities and abundance of other sub- strates [5,6]. The ubiquitin proteasomal system (UPS) employs a series of activating (E1), conjugating (E2) and ligase (E3) enzymes and appends ubiquitin chains to substrates, and directs them to timely degradation by the proteasomal machinery [7]. For instance a crucial step in regulating mammalian G1-S transition is one which involves the degradation of p27Kip1 by the UPS, which is required for full activation of cyclin E-CDK2 complexes [8,9]. Fol- lowing its phosphorylation on Thr-187 by cyclin E-Cdk2, p27Kip1 is ubiquitinated by the SCF-Skp2 E3 ligase [10]. Ganoth et al. demonstrated that fully reconstituted SCF- Skp2 only ubiquitinates p27Kip1 when it is supplemented with Cks1 [11,12]. Investigations revealed that Cks1 in- teracts with the substrate recognition component in this complex, Skp2, and facilitates its p27Kip1 ubiquitination activity [12,13]. Until recently this was the only well characterized molecular role for Cks1 in mammalian systems. However, emerging research reveals many more diverse and p27Kip1 independent roles of Cks1 that en- compass growth signaling pathways [14-25], apoptosis [25] and even DNA damage responses [26,27]. Cks1 was discovered in 1986 in a screen that identified genes that allow temperature sensitive cdc2 mutants in yeast to grow at the restrictive temperature [28-30]. The screen identified a molecule called Suc1 (Suppressor of cdc2) which when present on multicopy plasmids in Schizosaccharomyces pombe could rescue cells mutated in their cdc2 [28]. The study hinted that the action of- Suc1 was specific to cdc2 suggesting direct interaction between the two [28,29]. Indeed it is now recognized that Cks1 (Suc1 in S. pombe) does interact with CDKs at a site that is distinct from their ATP binding or cyclin binding sites [31-33]. Further characterization revealed Copyright © 2013 SciRes. JCT
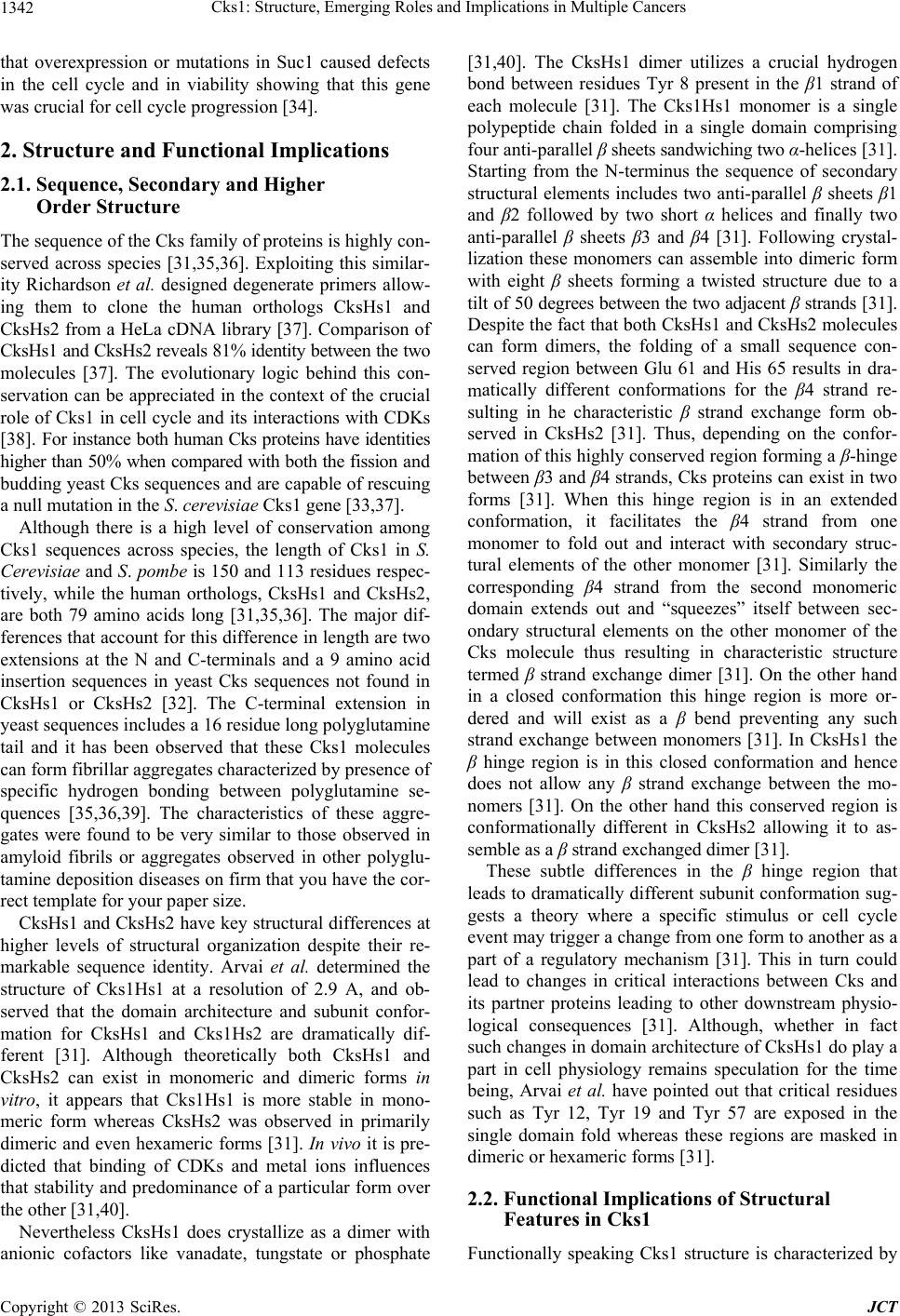 Cks1: Structure, Emerging Roles and Implications in Multiple Cancers 1342 that overexpression or mutations in Suc1 caused defects in the cell cycle and in viability showing that this gene was crucial for cell cycle progression [34]. 2. Structure and Functional Implications 2.1. Sequence, Secondary and Higher Order Structure The sequence of the Cks family of proteins is highly con- served across species [31,35,36]. Exploiting this similar- ity Richardson et al. designed degenerate primers allow- ing them to clone the human orthologs CksHs1 and CksHs2 from a HeLa cDNA library [37]. Comparison of CksHs1 and CksHs2 reveals 81% identity between the two molecules [37]. The evolutionary logic behind this con- servation can be appreciated in the context of the crucial role of Cks1 in cell cycle and its interactions with CDKs [38]. For instance both human Cks proteins have identities higher than 50% when compared with both the fission and budding yeast Cks sequences and are capable of rescuing a null mutation in the S. cerevisiae Cks1 gene [33,37]. Although there is a high level of conservation among Cks1 sequences across species, the length of Cks1 in S. Cerevisiae and S. pom be is 150 and 113 residues respec- tively, while the human orthologs, CksHs1 and CksHs2, are both 79 amino acids long [31,35,36]. The major dif- ferences that account for this difference in length are two extensions at the N and C-terminals and a 9 amino acid insertion sequences in yeast Cks sequences not found in CksHs1 or CksHs2 [32]. The C-terminal extension in yeast sequences includes a 16 residue long polyglutamine tail and it has been observed that these Cks1 molecules can form fibrillar aggregates characterized by presence of specific hydrogen bonding between polyglutamine se- quences [35,36,39]. The characteristics of these aggre- gates were found to be very similar to those observed in amyloid fibrils or aggregates observed in other polyglu- tamine deposition diseases on firm that you have the cor- rect template for your paper size. CksHs1 and CksHs2 have key structural differences at higher levels of structural organization despite their re- markable sequence identity. Arvai et al. determined the structure of Cks1Hs1 at a resolution of 2.9 A, and ob- served that the domain architecture and subunit confor- mation for CksHs1 and Cks1Hs2 are dramatically dif- ferent [31]. Although theoretically both CksHs1 and CksHs2 can exist in monomeric and dimeric forms in vitro, it appears that Cks1Hs1 is more stable in mono- meric form whereas CksHs2 was observed in primarily dimeric and even hexameric forms [31]. In vivo it is pre- dicted that binding of CDKs and metal ions influences that stability and predominance of a particular form over the other [31,40]. Nevertheless CksHs1 does crystallize as a dimer with anionic cofactors like vanadate, tungstate or phosphate [31,40]. The CksHs1 dimer utilizes a crucial hydrogen bond between residues Tyr 8 present in the β1 strand of each molecule [31]. The Cks1Hs1 monomer is a single polypeptide chain folded in a single domain comprising four anti-parallel β sheets sandwiching two α-helices [31]. Starting from the N-terminus the sequence of secondary structural elements includes two anti-parallel β sheets β1 and β2 followed by two short α helices and finally two anti-parallel β sheets β3 and β4 [31]. Following crystal- lization these monomers can assemble into dimeric form with eight β sheets forming a twisted structure due to a tilt of 50 degrees between the two adjacent β strands [31]. Despite the fact that both CksHs1 and CksHs2 molecules can form dimers, the folding of a small sequence con- served region between Glu 61 and His 65 results in dra- matically different conformations for the β4 strand re- sulting in he characteristic β strand exchange form ob- served in CksHs2 [31]. Thus, depending on the confor- mation of this highly conserved region forming a β-hinge between β3 and β4 strands, Cks proteins can exist in two forms [31]. When this hinge region is in an extended conformation, it facilitates the β4 strand from one monomer to fold out and interact with secondary struc- tural elements of the other monomer [31]. Similarly the corresponding β4 strand from the second monomeric domain extends out and “squeezes” itself between sec- ondary structural elements on the other monomer of the Cks molecule thus resulting in characteristic structure termed β strand exchange dimer [31]. On the other hand in a closed conformation this hinge region is more or- dered and will exist as a β bend preventing any such strand exchange between monomers [31]. In CksHs1 the β hinge region is in this closed conformation and hence does not allow any β strand exchange between the mo- nomers [31]. On the other hand this conserved region is conformationally different in CksHs2 allowing it to as- semble as a β strand exchanged dimer [31]. These subtle differences in the β hinge region that leads to dramatically different subunit conformation sug- gests a theory where a specific stimulus or cell cycle event may trigger a change from one form to another as a part of a regulatory mechanism [31]. This in turn could lead to changes in critical interactions between Cks and its partner proteins leading to other downstream physio- logical consequences [31]. Although, whether in fact such changes in domain architecture of CksHs1 do play a part in cell physiology remains speculation for the time being, Arvai et al. have pointed out that critical residues such as Tyr 12, Tyr 19 and Tyr 57 are exposed in the single domain fold whereas these regions are masked in dimeric or hexameric forms [31]. 2.2. Functional Implications of Structural Features in Cks1 Functionally speaking Cks1 structure is characterized by Copyright © 2013 SciRes. JCT
 Cks1: Structure, Emerging Roles and Implications in Multiple Cancers 1343 the presence of three regions including a cdc2/CDK binding region, a Skp2 binding region and an anion/ phosphate binding pocket [31,32,41-43] (see Table 1 for the key residues mediating the interactions in these func- tional regions). Another striking feature of Cks1 structure is that the CksHs1 molecule shows a strong homology to the N-lobe domain of CDK2 [31,32,41-43]. That Cks1 binds to cdc28 and cdc2 was already established during early coimmunoprecipitation studies with yeast homo- logs of Cks1 [44]. Similarly it was later demonstrated that Xe-p9 could also interact with Cdc2/cyclinB com- plexes [45]. The crystal structure of human CDK2 in complex with CksHS1 revealed that Cks1 utilizes all four beta sheets for this interaction and binds specifically to the C-terminal lobe of CDK [32]. Also it was revealed that the CDK domain does not interact with the cyclin binding sites or regulatory phosphate binding sites [32]. Cks1 can bind to CDK only in its monomer conformation and uses specific hydrophobic residues (see Table 1) as well as the conserved β-hinge region [32]. On the other hand the opening of this β hinge, which is the character- istic feature of the beta strand exchanged dimer does not allow Cks to bind to CDK2 [32]. The ternary complex of Skp1-Skp2-Cks1 in associa- tion with a phosphorylated p27Kip peptide elucidated by Pavletich et al. has provided significant insights into how Cks1 influences recognition of p27Kip1 by the SCF com- plex [42]. It is a sickle shaped binary structure where Skp1 and the F-box domain of Skp2 form the handle and the Skp2 leucine rich repeat (LRR) domain forms the curved blade [42]. The C-terminal tail of Skp2 at the end of tenth LRR curves inwards and interacts with first LRR. The Cks1 molecule docks into this concave groove form- Table 1. Cks1 structural features and their functional im- plications. Property Residue Region and Contacts Anion Binding Pocket (Binds pThr187 of p27Kip1) K11 R20 S51 W54 R71 β1 β2 Between α2 and β3 β3 β4 (all involved in charge-stabilized H-bonding) Cdk binding region Y12 Y19, H21, M23 Y57, M58 H60, P62, I66, L68, R70 E63 β1 (hydrophobic) β2 (hydrophobic) Between β2 and β3 β4 (hydrophobic) β4 (H-bonding) Skp2 binding region E40, S41, E42, N45 L31, P33 H36, M38 α2 (H-bonding) α1 (van der Waals) α2 (van der Waals) Dimer interaction sites Y8 I6, Y7, D10 H21 M23, Q49 β1 (H-bonding) β1 β2 between α2 and β3 ed by the LRR and C-terminal tail of Skp2. Three key residues (see Table 1) of Cks1 are required for H-bond- ing interactions with Skp2 and map into the 2 helix of Cks1 [41,42]. Phosphorylated p27Kip1 is recognized by phosphate binding pocket of Cks1 and p27Kip1 forms re- gions of contacts with both Cks1 and Skp2 by inserting a crucial Glu185 residue in the interface formed between Cks1 and Skp2 [41,42]. Hydrogen bonding between highly conserved Skp2 residues (Trp265, Arg294, Asp319, and Arg344 side chains) and those of Cks1 (Ser41, Glu40, and Asn45 side chains and Ser41 car- bonyl group) is a crucial prerequisite for Cks1-Skp2 in- teraction and hence altering these Cks1 residues is known to compromise the p27Kip1 ubiquitination activity of the SCF complex [41,42]. The residues Ser41 and Asn 45, are replaced by Glu and Arg residues in CksHs2 pre- cluding its interaction with Skp2 [41,42]. Yeast Skp2 is not known to interact with Cks1, although Cks1 in yeast does play a role in the multi-site phosphorylation of Sic1, the CDK inhibitor that is homologous to mammalian p27Kip1 [46]. The ubiquitination of p27Kip1 is preceded by its phos- phorylation at residue Thr187 which is then recognized by the phosphate binding pocket present in Cks1 [31,41,42, 47,48]. Two anion binding pockets are present near the dimer interface within a crevice formed by the dimer association [31]. In fact negatively charged moieties like vanadate or tungstate were used to facilitate crystalliza- tion of CksHs1 by Arvai et al. [40]. Crucial van der Walls contacts and hydrogen bonding contacts from residues Lys11, Arg20 and Arg71 and to the main chain nitrogen atom of Ser51 stabilize the interaction with these moieties [42]. 3. Diverse Cellular Functions of Cks1 Cks1 roles can be broadly classified into four categories: 1) roles in modulating cell cycle, 2) roles in transcription, 3) roles in growth signaling pathways, and 4) other emerging roles. Excellent reviews describing some of these functions of Cks1 are available [49-53]. 3.1. Cks1 Roles in Modulating the Cell Cycle The evidence for Cks1 modulating the cell cycle was provided by Tang and Reed where it was reported that loss of Cks1 in S. cerevisiae causes defects in both G1-S and G2-M transition [54]. However the mechanism by which Cks1 regulates G1-S transitions in mammalian systems was elucidated in two independent reports by Ganoth et al. and Spruck et al. which showed that Cks1 is indispensable for proteasomal degradation of p27Kip1 [12,13]. Ganoth et al. showed that a fully reconstituted SCF-Skp2 complex cannot ubiquitinate p27 in-vitro unless it was supplemented by a crucial factor (Factor I) Copyright © 2013 SciRes. JCT
 Cks1: Structure, Emerging Roles and Implications in Multiple Cancers 1344 derived from the unbound fraction of the HeLa cell ex- tracts fractionated on DEAE–cellulose columns [12]. Further purification and characterization of this unknown factor revealed that this factor was Cks1 [12]. Further- more this report established that Cks1’s role in p27Kip1 degradation was specific to the SCF-Skp2 ligase since it had no impact on the activity of other ubiquitin ligases like SCF β-TrCP [12]. Spruck et al. utilized MEFs de- rived from Cks1−/− mice [13]. Cks1−/− mice exhibit a smaller body size, which is possibly due to proliferative abnormalities (due to accumulation of p27Kip1 in somatic cells during embryonic development) [13]. Interestingly the roles of Cks1 in p27Kip1 degradation have been sug- gested to be independent of its interactions with CDKs. A Cks1 mutant defective in CDK binding (E63Q) has been shown to be capable of p27Kip1 ubiquitination [13]. On the other hand other mutations in the CDK2 binding site of Cks1 do reduce the ability of cyclin A-CDK2 to promote p27Kip1 ubiquitination [41]. Although phos- phorylation of p27Kip1 on residues Thr-187 by cyclin E/A-CDK2 complex is a crucial prerequisite for its rec- ognition and proteasomal targeting by the SCF-Skp2 ubiquitin ligase, whether the interactions of Cks1 with CDK2 itself have any impact on its p27Kip1 ubiquitination activity remains controversial [13,41,42]. Three models have been suggested to describe the role of Cks1 in p27Kip1 ubiquitination [50]. The first model suggests that binding of Cks1 to the C-terminal of Skp2 results in a necessary conformational change that allows the Skp2 to interact efficiently with its phosphorylated substrate, p27Kip1. This model depicts a binding site of Cks1 wherein Cks1 docks in a concave groove formed by the LRR region and the C-terminal tail of Skp2 [42,50]. It is suggested that interaction of Cks1 with the C-ter- minal tail region of the Skp2 molecule “nudges” the oc- cluding LRR region and allows efficient interaction be- tween LRR and the phosphorylated substrate [42,50,55]. The second model suggests that Cks1 may physically act as an adaptor or bridge between phosphorylated substrate p27Kip1 and F-box protein Skp2 [42]. It is possible that the phosphate binding site of Cks1 recognizes phos- phorylated p27Kip1 which is then brought in close prox- imity to Skp2 for ubiquitination [42]. Although it has been shown that Cks1 interaction with CDK2 is not nec- essary for p27Kip1 degradation, it has been suggested in a third model that Cks1-CDK2 binding pulls out the p27Kip1-cyclin A-Cdk2 complex and favors its interaction with SCF-Skp2 complex [42]. Although further genetic and biochemical studies are required to distinguish be- tween these possibilities, the role of Cks1 in p27Kip1 deg- radation is indisputable. Cks1 also regulates the proteasomal degradation of p130/Rb2 which is both a pocket protein and an inhibitor of CDK2 [56,57]. The expression of p130 is largely re- stricted to G0 phase of the cell cycle [56,57]. Like p27Kip1 the turnover of p130 is regulated proteasomally [58]. As proliferating cells enter from G0 phase to G1, p130 gets phosphorylated by CDK4/6 complexes and is proteasomally degraded by a process which employs the SCF-Skp2 E3 ubiquitin ligase complex [58]. In fact loss of either Skp2 or Cks1 impairs proteasomal stability of p130 as evidenced by its accumulation in asynchronous or thymidine arrested Skp2−/− and Cks1−/− fibroblasts [58]. Thus Cks1 is responsible not only for regulating the p27Kip1 degradation but also plays a pivotal role in de- termining p130 stability in proliferating cells [58]. Cks1 has also been implicated in the degradation of mitotic substrates by regulating the APC/C ubiquitin li- gase and spindle assembly checkpoint (SAC), which is crucial for orchestrating mitotic timing [59-62]. The SAC fine-tunes the timing of proteasomal degradation through APC/C ubiquitin ligase by inactivating its coactivator Cdc20 [63,64]. This in turn prevents premature degrada- tion of APC/C mitotic substrates like securin and cyclin B1 ensuring accurate sister chromatid separation before mitotic exit [63,64]. However other APC/C substrates like cyclin A are degraded even though the SAC is still active [65]. Studies by Di Fiore et al. and Wolthius et al. have explained this paradox by showing that the N-ter- minus of cyclin A competes with SAC proteins to bind to Cdc20 [66,67]. Following this Cks1 recruits the cyclin A-cdc20 complex to a phosphorylated APC/C ubiquitin ligase, triggering cyclin A ubiquitination and subsequent proteasomal degradation. [66,67]. Furthermore it was demonstrated that the anion binding site of Cks1 is cru- cial for the SAC independent degradation of cyclin A [66,67]. Loss of Cks1 also results in impaired mitotic passage in yeast and in Xenopu s egg extracts [45,54]. In Xenopus egg extracts, Cks1 is crucial for dephosphorylation of Y15 residue of CDK1 [45]. Cks1 promotes MPF depen- dent phosphorylation of cdc25c phosphatases, wee 1 and myt1 kinases, all molecules that participate in CDK1 activation by removing inhibitory phosphorylation from Cdk1 [45]. Xe-p9 (Xenopus homologue of Cks1) is also known to promote cyclin B1 degradation [68,69]. More specifically Xe-p9 regulates mitotic exit by stimulating phosphorylation of cdc27, another important component of the APC/C complex that targets cyclin B1 and several other mitotic molecules for proteasomal degradation, thus orchestrating mitotic exit in these cells [68,69]. Studies in our laboratory have shown that loss of Cks1 in MCF-7 breast cancer cells leads to blockade in mitotic entry with corresponding loss of CDK1 [70]. Further- more the mitotic block can be rescued by reintroduction of exogenous CDK1 [70]. A report by Martinsson-Ahl- zen et al. has also demonstrated that loss of Cks1 in MEFs and HeLa cells results in cell cycle arrest in G2 Copyright © 2013 SciRes. JCT
 Cks1: Structure, Emerging Roles and Implications in Multiple Cancers 1345 phases which can be reversed reintroduction of cyclin B1 [71]. Interestingly Cks1 loss does not alter cell cycle pro- files in human mammary epithelial cells (HMECs) or normal human lung fibroblasts [70,72]. Although G1-S defects following Cks1 loss in mam- malian cells can be accounted for by p27Kip1 accumula- tion, recent reports have indicated that some defects can also be explained in part by p27Kip1 independent mecha- nisms [73]. In studies reported by Keller et al. it was found that CDK1 kinase activity of the Cks1−/− MEF cells was not altered whereas there is considerable loss in CDK2 kinase activity [73]. Furthermore concomitant loss of p27Kip1 in Cks1−/− MEF did not rescue this loss in Cdk2 kinase activity indicating that part of G1-S defects observed are p27Kip1 independent and a direct cones- quence of Cks1 interaction with CDK2 activity [73]. In fact a report by Liberal et al. has shown that Cks1 can override DNA damage response by increasing CDK2 kinase activity and overriding the inhibitory effects of Y15 phosphorylation on CDK2 [27]. 3.2. Cks1 Roles in Transcription Recently Cks1 has also been implicated in transcriptional regulation [71,74-77]. In fact some of these transcrip- tional roles also indicate other ways by which Cks1 might be involved in regulating cell cycle transitions [71, 76]. For instance, Cdc20 has been shown to be a tran- scriptional target of Cks1 [76]. Cdc20 is a regulator which associates with and modulates the activity of the APC ubiquitin ligase during distinct phases of cell cycle [78]. It was shown that Cks1 is essential for dissociation of Cdc28 from Cdc20 promoter and recruitment of spe- cific proteasomal subunits like Rpt1, Pre1 on this pro- moter [76]. It has been suggested that this periodic asso- ciation and dissociation events on the Cdc20 promoter facilitates remodeling of transcriptional complexes dock- ed on the Cdc20 promoter [76]. It has also been recently reported that that Cks1 plays a role in GAL1 transcrip- tion, whereby Cks1, CDK1 and the 19S subunit of the proteasome are recruited to the GAL1 promoter, specifi- cally attaching to the histone H4 amino-terminal tail of the chromatin [74]. This activity has been reported to alter nucleosome density and evict nucleosomes from the chromatin region thereby inducing GAL1 transcription [74]. Cks1 has also been shown to transcriptionally regulate the expression of cdc2, cyclin B and cyclin A in mammalian cells [71]. The regulation of Cks1 gene expression itself is now known to be regulated through transcriptional mecha- nisms [79-87]. Cks1 mRNA levels start rising in late G1 reaching a peak before the onset of S-phase [88]. An- other peak is observed at G2/M phase of the cell cycle [37]. Various reports have provided insights into possible transcriptional regulators for Cks1 [79-86]. Mutating a potential CDE/CHR (cell cycle dependent element and cell cycle genes homology region) tandem repeat within Cks1 promoter compromises transcriptional activation of Cks1 indicating that this element acts as a possible tran- scriptional regulator [87]. Although Cks1 does not have p53 binding site, forced induction of p53 represses mRNA and protein expression of Cks1 possibly due to p53 dictated repression of Cks1 promoter [87]. On the other hand NF-Y, FoxM1, and Myc are known to act as transcriptional activators for Cks1 [80,81,83,85,87]. In fact Myc induced Cks1 activation has been proposed to be a switch that triggers p27Kip1 loss and subsequent cell proliferation and tumorigenesis by Keller et al. [81]. In primary T-lymphocytes CD28 along with T-cell re- ceptor (TCR) provides a co-stimulatory signal that is required for T cell activation and entry into S-phase [89, 90]. CD28 co-stimulatory signals downregulate p27Kip1 by inducing transcription of Skp2 and Cks1, thus en- hancing its proteasomal degradation [79]. TGF-treatment of mink lung epithelial cells and Hep3B cells also de- creases Cks1 mRNA transcripts, and this loss of Cks1 has been known to compromise p27Kip1 ubiquitination, and also triggers Skp2 autoubiquitination and degrada- tion [86]. Furthermore, B-Raf and cyclin D1 also down- regulate Cks1 expression at the mRNA and protein levels [15]. Despite many reports of Cks1 roles in transcription and the transcriptional regulation of Cks1 by other pro- teins, many gaps remain in our knowledge regarding the mechanisms of both these phenomena and require further investigation. 3.3. Cks1 Roles in Growth Signaling Pathways The roles of Cks1 in growth factor signaling pathways have started to emerge with few studies suggesting the involvement of Cks1 in MAPK, JAK-STAT and NF-B pathways [13-24]. Despite its crucial role in cell cycle and cancer progression, mechanistic studies regarding Cks1 role in growth signaling mechanisms are lacking. Cks1 siRNA knockdown decreases ERK1/2 phosphory- lation and triggers apoptosis in breast cancer cells MDA- MB-231 whereas its overexpression inhibits apoptosis and increases ERK1/2 phosphorylation hinting at a Cks1 modulated signaling event in this important pathway [25]. Another study showed that Cks1 shRNA mediated knockdown leads to decrease in ERK1/2, MEK and STAT phosphorylation in multiple myeloma cell lines KMS28PE, OCI-MY5 and XG-1 whereas forced over- expression of Cks1 activates ERK1/2 and STAT phos- phorylation in the later two cell lines [21]. Surprisingly Skp2 knockdown or p27Kip1 overexpression caused sup- pression of ERK/MEK and JAK/STAT signaling indi- cating that Cks1 may be involved at a crucial node of Copyright © 2013 SciRes. JCT
 Cks1: Structure, Emerging Roles and Implications in Multiple Cancers 1346 regulation of signaling events independently of Skp2 and p27Kip1 [21]. Although the role of Cks1 upstream of these kinases at the level of Ras GTPases and Raf kinases has not been investigated, squamous cell carcinomas and lung adeno- mas derived from RasH2 mice have been shown to ex- hibit fluctuations in levels of Cks1 when treated with genotoxic agents like 7,12-dimethylbenz[a]anthracene (DMBA), urethane and N-ethyl-N-nitrosourea (ENU) [91]. It has also been shown that adaptor protein FGF receptor 2 (FRS2) associates with Cks1 and FGF dependent phosphorylation of FRS2 releases Cks1 and causes con- comitant p27Kip1 degradation in 3T3 cells [24]. Recently the role of Cks1 in NF-B induced hepatocellular cancer has also been reported whereby Cks1 transcriptionally regulates IB and hence drives NF-B mediated IL-8 driv- en hepatocellular carcinoma [19]. Given that Cks1 is overexpressed in several different tumor types, its role as a signaling modulator remains a fruitful and unexplored avenue of research. 3.4. Other Roles of Cks1 Although the majority of research on Cks1 has been conducted using yeast and mammalian systems, there are a number of studies that have utilized other systems in- cluding Xenopus laevis [45], Caenorhabditis elegans [92], Drosophila melanogaster [93], Branchiostoma belcheri tsingtauense [94], Leishmania Mexicana [95] and Patella vulgate [96]. Cks1At, an A. thaliana ho- molog of Cks1 was shown by Montagu et al. to bind to arabidopsis CDKs Cdc2aAt and Cdc2bAt [97,98]. Fur- ther characterization of Cks1At showed that overexpres- sion of Cks1 in A. thaliana lead to a reduction in leaf growth and root growth rates due to elongated G1 and G2 phases of the cell cycle [99]. Similarly some parasitic animal models have also been used in certain studies to isolate and characterize homologs of the Cks1 protein. For instance Leishmania mexicana has been used to iso- late p12Cks1 which is the functional homolog of p13Suc1 counterpart found in yeast [95,100]. Not surprisingly this protein was found to interact with yeast and bovine cdc2 as well as with CRK1 and SBCRK1 which are cdc2- related kinases found in these parasites [95,100]. Recent studies with Branchiostoma belcheri (Amphioxus) have also suggested a potential developmental role of Cks1 [94]. Studies on Cks30 (Cks2 homologue in Drosophila) and Cks85A (Cks1 homologue in Drosophhila) have also demonstrated their role in female meiosis and mitosis during embryonic development, and maintenance of cell viability respectively [93,101-103]. In yet another report it was shown that Cks1 transcript levels gradually increase with increasing follicle size in bovine embryos possibly to ensure proper G2/M timing of cell cycle in the embryonic cells [82]. Skp2 and Cks1 also have been shown to play a crucial role in S/G2 phase transition during adipocyte differentiation in 3T3-L1 preadipocytes [104]. Yu et al. have also recently demon- strated that a balance between Cks1 and Cks2 regulates p27kip1 abundance and neuronal development in mouse embryonic cells [26]. Collectively these reports suggest novel roles of Cks family of protein in developmental biology which await further characterization. More re- cently Cks1 has also been shown to play a role in mito- chondrial DNA replication regulating the mitochondrial single-stranded DNA-binding protein (mtSSB) function [105]. In another report demonstrating a p27kip1 inde- pendent Cks1 role it has been shown that Cks1 and Cks2 overexpression are involved in overcoming the DNA damage response following oncoprotein activation in breast cancer cells [27]. 4. Cks1—Implications in Breast and Other Cancers The importance of Cks1 in cancer progression can be understood given its pleiotropic roles in diverse biologi- cal processes that are known to be deregulated in cancer. Cks1 is overexpressed in a majority of human cancers and its expression is strongly correlated to tumor aggres- siveness and dissemination of disease. Cks1 and its im- plications in cancers have been addressed herein by re- viewing studies of Cks1 transcript levels, protein expres- sion and gene amplification (the Cks1 gene is located on 1q21) in normal and/or cancer derived samples from pa- tient cohorts of different cancer subtypes and their corre- lation to cancer clinicopathologic parameters. Because of its known role in the SCF-Skp2 complex many of these studies have also examined correlation of Cks1 expres- sion to that of Skp2 and p27Kip1 or other cancer related markers (such as p53 and Ki-67) [106]. In general Cks1 expression is strongly correlated with Skp2 expression and inversely related to p27Kip1 expression [106-109]. However many studies including ours have reported dif- ferent trends in expression pattern of these three genes emphasizing potentially underappreciated p27Kip1 inde- pendent mechanisms of Cks1 in cancer progression [110-112]. Another area of focus involves studies that attempt to determine the effect of Cks1 perturbation on cancer phenotypes (e.g. colony formation, migration and invasion, resistance to therapy etc.). For this discussion we broadly focus on two areas a) Cks1 expression, roles and implications in breast cancer, and b) Cks1 in other cancers. 4.1. Cks1 and Breast Cancer We have found that normal mouse or rat tissues exhibit nearly undetectable levels of Cks1 protein, whereas both Copyright © 2013 SciRes. JCT
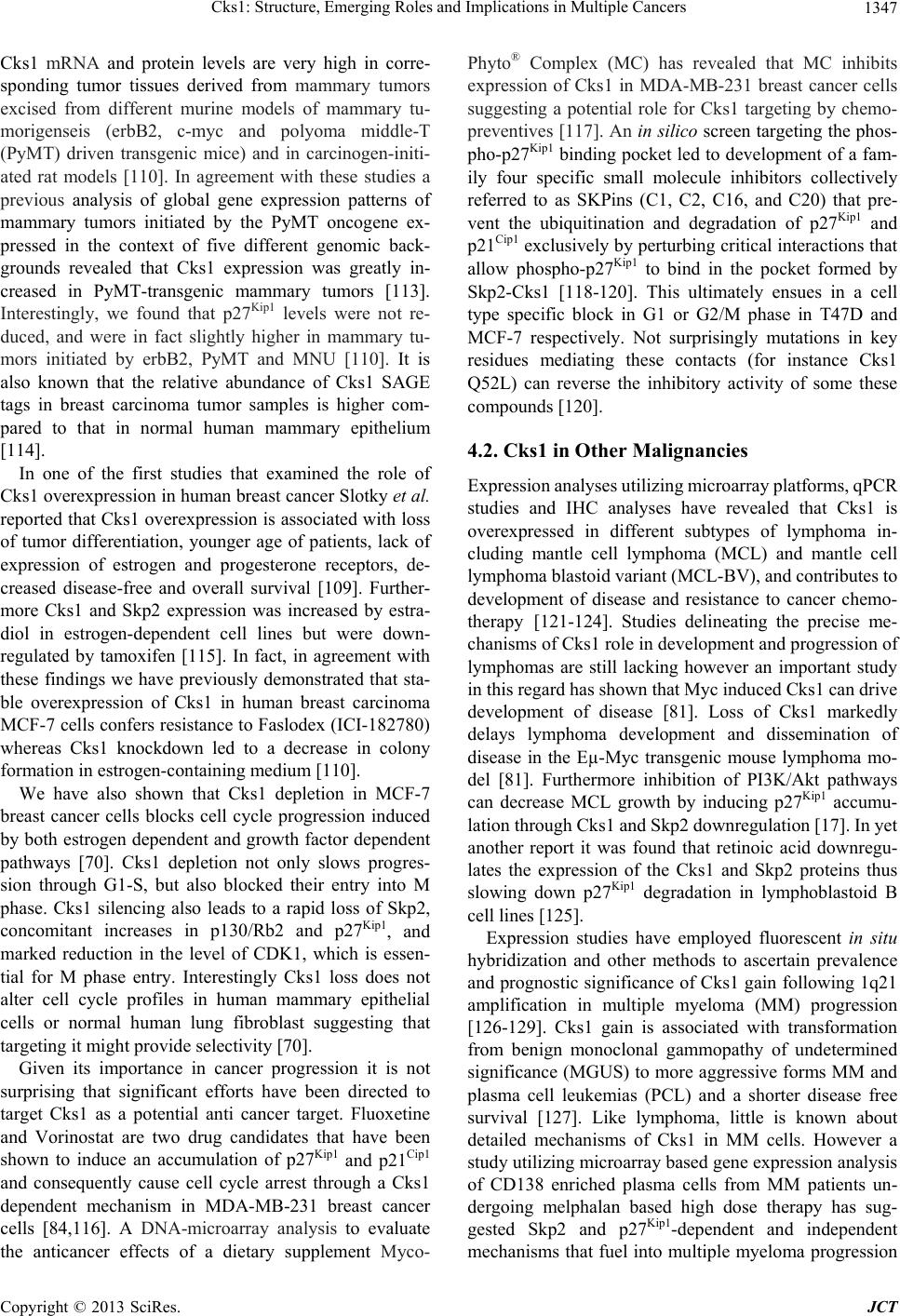 Cks1: Structure, Emerging Roles and Implications in Multiple Cancers 1347 Cks1 mRNA and protein levels are very high in corre- sponding tumor tissues derived from mammary tumors excised from different murine models of mammary tu- morigenseis (erbB2, c-myc and polyoma middle-T (PyMT) driven transgenic mice) and in carcinogen-initi- ated rat models [110]. In agreement with these studies a previous analysis of global gene expression patterns of mammary tumors initiated by the PyMT oncogene ex- pressed in the context of five different genomic back- grounds revealed that Cks1 expression was greatly in- creased in PyMT-transgenic mammary tumors [113]. Interestingly, we found that p27Kip1 levels were not re- duced, and were in fact slightly higher in mammary tu- mors initiated by erbB2, PyMT and MNU [110]. It is also known that the relative abundance of Cks1 SAGE tags in breast carcinoma tumor samples is higher com- pared to that in normal human mammary epithelium [114]. In one of the first studies that examined the role of Cks1 overexpression in human breast cancer Slotky et al. reported that Cks1 overexpression is associated with loss of tumor differentiation, younger age of patients, lack of expression of estrogen and progesterone receptors, de- creased disease-free and overall survival [109]. Further- more Cks1 and Skp2 expression was increased by estra- diol in estrogen-dependent cell lines but were down- regulated by tamoxifen [115]. In fact, in agreement with these findings we have previously demonstrated that sta- ble overexpression of Cks1 in human breast carcinoma MCF-7 cells confers resistance to Faslodex (ICI-182780) whereas Cks1 knockdown led to a decrease in colony formation in estrogen-containing medium [110]. We have also shown that Cks1 depletion in MCF-7 breast cancer cells blocks cell cycle progression induced by both estrogen dependent and growth factor dependent pathways [70]. Cks1 depletion not only slows progres- sion through G1-S, but also blocked their entry into M phase. Cks1 silencing also leads to a rapid loss of Skp2, concomitant increases in p130/Rb2 and p27Kip1, and marked reduction in the level of CDK1, which is essen- tial for M phase entry. Interestingly Cks1 loss does not alter cell cycle profiles in human mammary epithelial cells or normal human lung fibroblast suggesting that targeting it might provide selectivity [70]. Given its importance in cancer progression it is not surprising that significant efforts have been directed to target Cks1 as a potential anti cancer target. Fluoxetine and Vorinostat are two drug candidates that have been shown to induce an accumulation of p27Kip1 and p21Cip1 and consequently cause cell cycle arrest through a Cks1 dependent mechanism in MDA-MB-231 breast cancer cells [84,116]. A DNA-microarray analysis to evaluate the anticancer effects of a dietary supplement Myco- Phyto® Complex (MC) has revealed that MC inhibits expression of Cks1 in MDA-MB-231 breast cancer cells suggesting a potential role for Cks1 targeting by chemo- preventives [117]. An in silico screen targeting the phos- pho-p27Kip1 binding pocket led to development of a fam- ily four specific small molecule inhibitors collectively referred to as SKPins (C1, C2, C16, and C20) that pre- vent the ubiquitination and degradation of p27Kip1 and p21Cip1 exclusively by perturbing critical interactions that allow phospho-p27Kip1 to bind in the pocket formed by Skp2-Cks1 [118-120]. This ultimately ensues in a cell type specific block in G1 or G2/M phase in T47D and MCF-7 respectively. Not surprisingly mutations in key residues mediating these contacts (for instance Cks1 Q52L) can reverse the inhibitory activity of some these compounds [120]. 4.2. Cks1 in Other Malignancies Expression analyses utilizing microarray platforms, qPCR studies and IHC analyses have revealed that Cks1 is overexpressed in different subtypes of lymphoma in- cluding mantle cell lymphoma (MCL) and mantle cell lymphoma blastoid variant (MCL-BV), and contributes to development of disease and resistance to cancer chemo- therapy [121-124]. Studies delineating the precise me- chanisms of Cks1 role in development and progression of lymphomas are still lacking however an important study in this regard has shown that Myc induced Cks1 can drive development of disease [81]. Loss of Cks1 markedly delays lymphoma development and dissemination of disease in the Eµ-Myc transgenic mouse lymphoma mo- del [81]. Furthermore inhibition of PI3K/Akt pathways can decrease MCL growth by inducing p27Kip1 accumu- lation through Cks1 and Skp2 downregulation [17]. In yet another report it was found that retinoic acid downregu- lates the expression of the Cks1 and Skp2 proteins thus slowing down p27Kip1 degradation in lymphoblastoid B cell lines [125]. Expression studies have employed fluorescent in situ hybridization and other methods to ascertain prevalence and prognostic significance of Cks1 gain following 1q21 amplification in multiple myeloma (MM) progression [126-129]. Cks1 gain is associated with transformation from benign monoclonal gammopathy of undetermined significance (MGUS) to more aggressive forms MM and plasma cell leukemias (PCL) and a shorter disease free survival [127]. Like lymphoma, little is known about detailed mechanisms of Cks1 in MM cells. However a study utilizing microarray based gene expression analysis of CD138 enriched plasma cells from MM patients un- dergoing melphalan based high dose therapy has sug- gested Skp2 and p27Kip1-dependent and independent mechanisms that fuel into multiple myeloma progression Copyright © 2013 SciRes. JCT
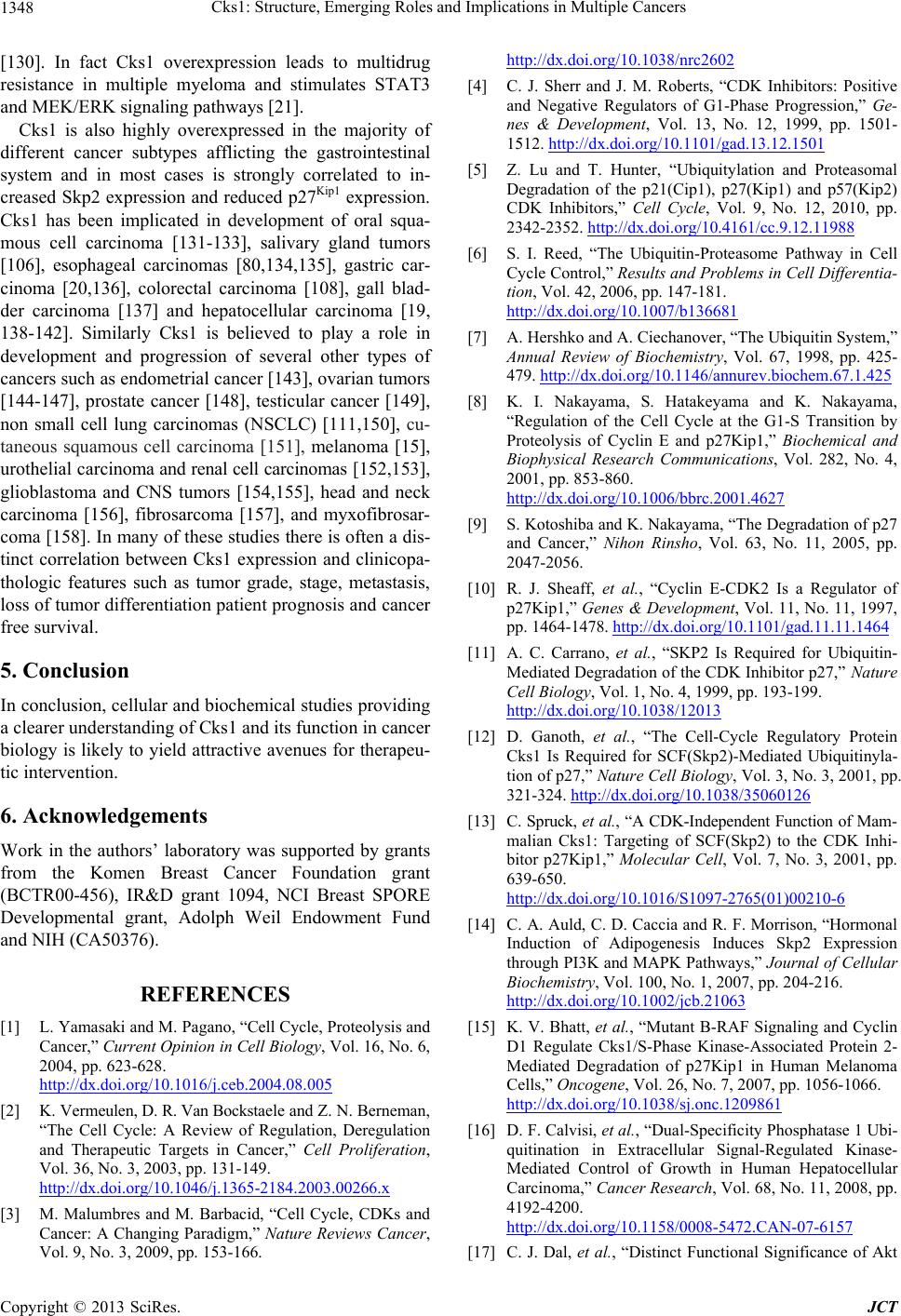 Cks1: Structure, Emerging Roles and Implications in Multiple Cancers 1348 [130]. In fact Cks1 overexpression leads to multidrug resistance in multiple myeloma and stimulates STAT3 and MEK/ERK signaling pathways [21]. Cks1 is also highly overexpressed in the majority of different cancer subtypes afflicting the gastrointestinal system and in most cases is strongly correlated to in- creased Skp2 expression and reduced p27Kip1 expression. Cks1 has been implicated in development of oral squa- mous cell carcinoma [131-133], salivary gland tumors [106], esophageal carcinomas [80,134,135], gastric car- cinoma [20,136], colorectal carcinoma [108], gall blad- der carcinoma [137] and hepatocellular carcinoma [19, 138-142]. Similarly Cks1 is believed to play a role in development and progression of several other types of cancers such as endometrial cancer [143], ovarian tumors [144-147], prostate cancer [148], testicular cancer [149], non small cell lung carcinomas (NSCLC) [111,150], cu- taneous squamous cell carcinoma [151], melanoma [15], urothelial carcinoma and renal cell carcinomas [152,153], glioblastoma and CNS tumors [154,155], head and neck carcinoma [156], fibrosarcoma [157], and myxofibrosar- coma [158]. In many of these studies there is often a dis- tinct correlation between Cks1 expression and clinicopa- thologic features such as tumor grade, stage, metastasis, loss of tumor differentiation patient prognosis and cancer free survival. 5. Conclusion In conclusion, cellular and biochemical studies providing a clearer understanding of Cks1 and its function in cancer biology is likely to yield attractive avenues for therapeu- tic intervention. 6. Acknowledgements Work in the authors’ laboratory was supported by grants from the Komen Breast Cancer Foundation grant (BCTR00-456), IR&D grant 1094, NCI Breast SPORE Developmental grant, Adolph Weil Endowment Fund and NIH (CA50376). REFERENCES [1] L. Yamasaki and M. Pagano, “Cell Cycle, Proteolysis and Cancer,” Current Opinion in Cell Biology, Vol. 16, No. 6, 2004, pp. 623-628. http://dx.doi.org/10.1016/j.ceb.2004.08.005 [2] K. Vermeulen, D. R. Van Bockstaele and Z. N. Berneman, “The Cell Cycle: A Review of Regulation, Deregulation and Therapeutic Targets in Cancer,” Cell Proliferation, Vol. 36, No. 3, 2003, pp. 131-149. http://dx.doi.org/10.1046/j.1365-2184.2003.00266.x [3] M. Malumbres and M. Barbacid, “Cell Cycle, CDKs and Cancer: A Changing Paradigm,” Nature Reviews Cancer, Vol. 9, No. 3, 2009, pp. 153-166. http://dx.doi.org/10.1038/nrc2602 [4] C. J. Sherr and J. M. Roberts, “CDK Inhibitors: Positive and Negative Regulators of G1-Phase Progression,” Ge- nes & Development, Vol. 13, No. 12, 1999, pp. 1501- 1512. http://dx.doi.org/10.1101/gad.13.12.1501 [5] Z. Lu and T. Hunter, “Ubiquitylation and Proteasomal Degradation of the p21(Cip1), p27(Kip1) and p57(Kip2) CDK Inhibitors,” Cell Cycle, Vol. 9, No. 12, 2010, pp. 2342-2352. http://dx.doi.org/10.4161/cc.9.12.11988 [6] S. I. Reed, “The Ubiquitin-Proteasome Pathway in Cell Cycle Control,” Results and Problems in Cell Differentia- tion, Vol. 42, 2006, pp. 147-181. http://dx.doi.org/10.1007/b136681 [7] A. Hershko and A. Ciechanover, “The Ubiquitin System,” Annual Review of Biochemistry, Vol. 67, 1998, pp. 425- 479. http://dx.doi.org/10.1146/annurev.biochem.67.1.425 [8] K. I. Nakayama, S. Hatakeyama and K. Nakayama, “Regulation of the Cell Cycle at the G1-S Transition by Proteolysis of Cyclin E and p27Kip1,” Biochemical and Biophysical Research Communications, Vol. 282, No. 4, 2001, pp. 853-860. http://dx.doi.org/10.1006/bbrc.2001.4627 [9] S. Kotoshiba and K. Nakayama, “The Degradation of p27 and Cancer,” Nihon Rinsho, Vol. 63, No. 11, 2005, pp. 2047-2056. [10] R. J. Sheaff, et al., “Cyclin E-CDK2 Is a Regulator of p27Kip1,” Genes & Development, Vol. 11, No. 11, 1997, pp. 1464-1478. http://dx.doi.org/10.1101/gad.11.11.1464 [11] A. C. Carrano, et al., “SKP2 Is Required for Ubiquitin- Mediated Degradation of the CDK Inhibitor p27,” Nature Cell Biology, Vol. 1, No. 4, 1999, pp. 193-199. http://dx.doi.org/10.1038/12013 [12] D. Ganoth, et al., “The Cell-Cycle Regulatory Protein Cks1 Is Required for SCF(Skp2)-Mediated Ubiquitinyla- tion of p27,” Nature Cell Biology, Vol. 3, No. 3, 2001, pp. 321-324. http://dx.doi.org/10.1038/35060126 [13] C. Spruck, et al., “A CDK-Independent Function of Mam- malian Cks1: Targeting of SCF(Skp2) to the CDK Inhi- bitor p27Kip1,” Molecular Cell, Vol. 7, No. 3, 2001, pp. 639-650. http://dx.doi.org/10.1016/S1097-2765(01)00210-6 [14] C. A. Auld, C. D. Caccia and R. F. Morrison, “Hormonal Induction of Adipogenesis Induces Skp2 Expression through PI3K and MAPK Pathways,” Journal of Cellular Biochemistry, Vol. 100, No. 1, 2007, pp. 204-216. http://dx.doi.org/10.1002/jcb.21063 [15] K. V. Bhatt, et al., “Mutant B-RAF Signaling and Cyclin D1 Regulate Cks1/S-Phase Kinase-Associated Protein 2- Mediated Degradation of p27Kip1 in Human Melanoma Cells,” Oncogene , Vol. 26, No. 7, 2007, pp. 1056-1066. http://dx.doi.org/10.1038/sj.onc.1209861 [16] D. F. Calvisi, et al., “Dual-Specificity Phosphatase 1 Ubi- quitination in Extracellular Signal-Regulated Kinase- Mediated Control of Growth in Human Hepatocellular Carcinoma,” Cancer Research, Vol. 68, No. 11, 2008, pp. 4192-4200. http://dx.doi.org/10.1158/0008-5472.CAN-07-6157 [17] C. J. Dal, et al., “Distinct Functional Significance of Akt Copyright © 2013 SciRes. JCT
 Cks1: Structure, Emerging Roles and Implications in Multiple Cancers 1349 and mTOR Constitutive Activation in Mantle Cell Lym- phoma,” Blood, Vol. 111, No. 10, 2008, pp. 5142-5151. http://dx.doi.org/10.1182/blood-2007-07-103481 [18] R. Hu and A. E. Aplin, “AlphaB-Crystallin Is Mutant B- RAF Regulated and Contributes to Cyclin D1 Turnover in Melanocytic Cells,” Pigment Cell & Melanoma Research, Vol. 23, No. 2, 2010, pp. 201-209. http://dx.doi.org/10.1111/j.1755-148X.2010.00668.x [19] E. K. Lee, et al., “Cell-Cycle Regulator Cks1 Promotes Hepatocellular Carcinoma by Supporting NF-kappaB-De- pendent Expression of Interleukin-8,” Cancer Research, Vol. 71, No. 21, 2011, pp. 6827-6835. http://dx.doi.org/10.1158/0008-5472.CAN-10-4356 [20] S. W. Lee, et al., “Akt and Cks1 Are Related with Lymph Node Metastasis in Gastric Adenocarcinoma,” Hepato- gastroenterology, Vol. 60, 2013, p. 127. [21] L. Shi, et al., “Over-Expression of CKS1B Activates Both MEK/ERK and JAK/STAT3 Signaling Pathways and Promotes Myeloma Cell Drug-Resistance,” Onco- target, Vol. 1, No. 1, 2010, pp. 22-33. [22] K. E. Simon, H. H. Cha and G. L. Firestone, “Transform- ing Growth Factor Beta Down-Regulation of CKShs1 Transcripts in Growth-Inhibited Epithelial Cells,” Cell Growth & Differentiation, Vol. 6, No. 10, 1995, pp. 1261- 1269. [23] S. Suzuki, et al., “Up-Regulation of Cks1 and Skp2 with TNFalpha/NF-kappaB Signaling in Chronic Progressive Nephropathy,” Genes Cells, Vol. 16, No. 11, 2011, pp. 1110-1120. http://dx.doi.org/10.1111/j.1365-2443.2011.01553.x [24] Y. Zhang, et al., “Direct Cell Cycle Regulation by the Fibroblast Growth Factor Receptor (FGFR) Kinase through Phosphorylation-Dependent Release of Cks1 from FGFR Substrate 2,” Journal of Biological Chemistry, Vol. 279, No. 53, 2004, pp. 55348-55354. http://dx.doi.org/10.1074/jbc.M409230200 [25] X. C. Wang, et al., “Overexpression of Cks1 Is Associat- ed with Poor Survival by Inhibiting Apoptosis in Breast Cancer,” Journal of Cancer Research and Clinical On- cology, Vol. 135, No. 10, 2009, pp. 1393-1401. http://dx.doi.org/10.1007/s00432-009-0582-8 [26] M. Frontini, et al., “The CDK Subunit CKS2 Counteracts CKS1 to Control Cyclin A/CDK2 Activity in Maintaining Replicative Fidelity and Neurodevelopment,” Genes & Development, Vol. 23, No. 2, 2012, pp. 356-370. http://dx.doi.org/10.1016/j.devcel.2012.06.018 [27] V. Liberal, et al., “Cyclin-Dependent Kinase Subunit (Cks) 1 or Cks2 Overexpression Overrides the DNA Damage Response Barrier Triggered by Activated Oncoproteins,” Proceedings of the National Academy of Sciences of USA, Vol. 109, No. 8, 2012, pp. 2754-2759. http://dx.doi.org/10.1007/BF00331653 [28] J. Hayles, S. Aves and P. Nurse, “Suc1 Is an Essential Gene Involved in Both the Cell Cycle and Growth in Fis- sion Yeast,” EMBO Journal, Vol. 5, No. 12, 1986, pp. 3373-3379. [29] J. Hayles, et al., “The Fission Yeast Cell Cycle Control Gene cdc2: Isolation of a Sequence suc1 That Suppresses cdc2 Mutant Function,” Molecular and General Genetics, Vol. 202, No. 2, 1986, pp. 291-293. http://dx.doi.org/10.1007/BF00331653 [30] S. I. Reed, et al., “Analysis of the Cdc28 Protein Kinase Complex by Dosage Suppression,” Journal of Cell Sci- ence Supplement, Vol. 12, 1989, pp. 29-37. http://dx.doi.org/10.1242/jcs.1989.Supplement_12.4 [31] A. S. Arvai, et al., “Crystal Structure of the Human Cell Cycle Protein CksHs1: Single Domain Fold with Similar- ity to Kinase N-Lobe Domain,” Journal of Molecular Bi- ology, Vol. 249, No. 5, 1995, pp. 835-842. http://dx.doi.org/10.1006/jmbi.1995.0341 [32] Y. Bourne, et al., “Crystal Structure and Mutational Ana- lysis of the Human CDK2 Kinase Complex with Cell Cy- cle-Regulatory Protein CksHs1,” Cell, Vol. 84, No. 6, 1996, pp. 863-874. http://dx.doi.org/10.1016/S0092-8674(00)81065-X [33] J. A. Hadwiger, et al., “The Saccharomyces Cerevisiae CKS1 Gene, a Homolog of the Schizosaccharomyces Pombe suc1+ Gene, Encodes a Subunit of the Cdc28 Pro- tein Kinase Complex,” Molecular and Cellular Biology, Vol. 9, No. 5, 1989, pp. 2034-2041. [34] J. Hindley, et al., “Sucl+ Encodes a Predicted 13-Kilo- dalton Protein That Is Essential for Cell Viability and Is Directly Involved in the Division Cycle of Schizosacchar- omyces Pombe,” Molecular and Cellular Biology, Vol. 7, No. 1, 1987, pp. 504-511. [35] Y. Bourne, et al., “Crystal Structure and Mutational Ana- lysis of the Saccharomyces cerevisiae Cell Cycle Regu- latory Protein Cks1: Implications for Domain Swapping, Anion Binding and Protein Interactions,” Structure, Vol. 8, No. 8, 2000, pp. 841-850. http://dx.doi.org/10.1016/S0969-2126(00)00175-1 [36] N. Khazanovich, et al., “Crystal Structure of the Yeast Cell-Cycle Control Protein, P13suc1, in a Strand-Exchan- ged Dimmer,” Structure, Vol. 4, No. 3, 1996, pp. 299-309. http://dx.doi.org/10.1016/S0969-2126(96)00034-2 [37] H. E. Richardson, et al., “Human cDNAs Encoding Ho- mologs of the Small p34Cdc28/Cdc2-Associated Protein of Saccharomyces cerevisiae and Schizosaccharomyces pombe,” Genes & Development, Vol. 4, No. 8, 1990, pp. 1332-1344. http://dx.doi.org/10.1101/gad.4.8.1332 [38] A. Satyanarayana and P. Kaldis, “Mammalian Cell-Cycle Regulation: Several Cdks, Numerous Cyclins and Diverse Compensatory Mechanisms,” Oncogene, Vol. 28, No. 33, 2009, pp. 2925-2939. http://dx.doi.org/10.1038/onc.2009.170 [39] R. Bader, et al., “Folding and Fibril Formation of the Cell Cycle Protein Cks1,” The Journal of Biological Chemis- try, Vol. 281, No. 27, 2006, pp. 18816-18824. http://dx.doi.org/10.1074/jbc.M603628200 [40] A. S. Arvai, et al., “Crystallization and Preliminary Crys- tallographic Study of Human CksHs1: A Cell Cycle Re- gulatory Protein,” Proteins, Vol. 21, No. 1, 1995, pp. 70- 73. http://dx.doi.org/10.1002/prot.340210109 [41] D. Sitry, et al., “Three Different Binding Sites of Cks1 are Required for p27-Ubiquitin Ligation,” The Journal of Biological Chemistry, Vol. 277, No. 44, 2002, pp. 42233- 42240. http://dx.doi.org/10.1074/jbc.M205254200 [42] B. Hao, et al., “Structural Basis of the Cks1-Dependent Copyright © 2013 SciRes. JCT
 Cks1: Structure, Emerging Roles and Implications in Multiple Cancers 1350 Recognition of p27(Kip1) by the SCF(Skp2) Ubiquitin ligase,” Molecular Cell, Vol. 20, No. 1, 2005, pp. 9-19. http://dx.doi.org/10.1016/j.molcel.2005.09.003 [43] W. Wang, et al., “Molecular and Biochemical Charac- terization of the Skp2-Cks1 Binding Interface,” The Jour- nal of Biological Chemistry, Vol. 279, No. 49, 2004, pp. 51362-51369. http://dx.doi.org/10.1074/jbc.M405944200 [44] L. Brizuela, G. Draetta and D. Beach, “p13suc1 Acts in the Fission Yeast Cell Division Cycle as a Component of the p34cdc2 Protein Kinase,” The EMBO Journal, Vol. 6, No. 11, 1987, pp. 3507-3514. [45] D. Patra, et al., “The Xenopus Suc1/Cks Protein Pro- motes the Phosphorylation of G(2)/M Regulators,” The Journal of Biological Chemistry, Vol. 274, No. 52, 1999, pp. 36839-36842. http://dx.doi.org/10.1074/jbc.274.52.36839 [46] M. Koivomagi, et al., “Cascades of Multisite Phosphory- lation Control Sic1 Destruction at the Onset of S Phase,” Nature, Vol. 480, No. 7375, 2011, pp. 128-131. http://dx.doi.org/10.1038/nature10560 [47] S. Xu, et al., “Substrate Recognition and Ubiquitination of SCFSkp2/Cks1 Ubiquitin-Protein Isopeptide Ligase,” The Journal of Biological Chemistry, Vol. 282, No. 21, 2007, pp. 15462-15470. http://dx.doi.org/10.1074/jbc.M610758200 [48] A. Krishnan, S. A. Nair and M. R. Pillai, “Loss of Cks1 Homeostasis Deregulates Cell Division Cycle,” Journal of Cellular and Molecular Medicine, Vol. 14, No. 1-2, 2010, pp. 154-164. http://dx.doi.org/10.1111/j.1582-4934.2009.00698.x [49] T. Bashir and M. Pagano, “Don’t Skip the G1 Phase: How APC/CCdh1 Keeps SCFSKP2 in Check. Cell Cycle, Vol. 3, No. 7, 2004, pp. 850-852. http://dx.doi.org/10.4161/cc.3.7.977 [50] J. W. Harper, “Protein Destruction: Adapting Roles for Cks Proteins,” Current Biology, Vol. 11, No. 11, 2001, pp. R431-R435. http://dx.doi.org/10.1016/S0960-9822(01)00253-6 [51] A. Krishnan, S. A. Nair and M. R. Pillai, “Loss of Cks1 Homeostasis Deregulates Cell Division Cycle,” Journal of Cellular and Molecular Medicine, Vol. 14, No. 1-2, 2010, pp. 154-164. http://dx.doi.org/10.1111/j.1582-4934.2009.00698.x [52] J. Pines, “Cell Cycle: Reaching for a Role for the Cks Proteins,” Current Biology, Vol. 6, No. 11, 1996, pp. 1399-1402. http://dx.doi.org/10.1016/S0960-9822(96)00741-5 [53] J. Bartek and J. Lukas, “p27 Destruction: Cks1 Pulls the Trigger,” Nature Cell Biology, Vol. 3, No. 4, 2001, pp. E95-E98. http://dx.doi.org/10.1038/35070160 [54] Y. Tang and S. I. Reed, “The Cdk-Associated Protein Cks1 Functions Both in G1 and G2 in Saccharomyces cerevisiae,” Genes & Development, Vol. 7, No. 5, 1993, pp. 822-832. http://dx.doi.org/10.1101/gad.7.5.822 [55] B. A. Schulman, et al., “Insights into SCF Ubiquitin Li- gases from the Structure of the Skp1-Skp2 Complex,” Nature, Vol. 408, No. 6810, 2000, pp. 381-386. http://dx.doi.org/10.1038/35042620 [56] G. Mulligan and T. Jacks, “The Retinoblastoma Gene Fa- mily: Cousins with Overlapping Interests,” Trends in Ge- netics, Vol. 14, No. 6, 1998, pp. 223-229. http://dx.doi.org/10.1016/S0168-9525(98)01470-X [57] X. Mayol and X. Grana, “The p130 Pocket Protein: Keep- ing Order at Cell Cycle Exit/Re-Entrance Transitions,” Frontiers in Bioscience, Vol. 3, 1998, pp. d11-d24. [58] D. Tedesco, J. Lukas and S. I. Reed, “The pRb-Related Protein p130 is Regulated by Phosphorylation-Dependent Proteolysis via the Protein-Ubiquitin Ligase SCF(Skp2),” Genes & Development, Vol. 16, No. 22, 2002, pp. 2946- 2957. http://dx.doi.org/10.1101/gad.1011202 [59] R. Wasch and D. Engelbert, “Anaphase-Promoting Com- plex-Dependent Proteolysis of Cell Cycle Regulators and Genomic Instability of Cancer Cells,” Oncogene, Vol. 24, No. 1, 2005, pp. 1-10. http://dx.doi.org/10.1038/sj.onc.1208017 [60] L. Song and M. Rape, “Regulated Degradation of Spin- dle Assembly Factors by the Anaphase-Promoting Com- plex,” Molecular Cell, Vol. 38, No. 3, 2010, pp. 369-382. http://dx.doi.org/10.1016/j.molcel.2010.02.038 [61] A. M. Fry and H. Yamano, “APC/C-Mediated Degrada- tion in Early Mitosis: How to Avoid Spindle Assembly Checkpoint Inhibition,” Cell Cycle, Vol. 5, No. 14, 2006, pp. 1487-1491. http://dx.doi.org/10.4161/cc.5.14.3003 [62] S. Kim and H. Yu, “Mutual Regulation between the Spindle Checkpoint and APC/C,” Seminars in Cell & De- velopmental Biology, Vol. 22, No. 6, 2011, pp. 551-558. http://dx.doi.org/10.1016/j.semcdb.2011.03.008 [63] R. H. Chen, “Dual Inhibition of Cdc20 by the Spindle Checkpoint,” Journal of Biomedical Science, Vol. 14(4): 2007, pp. 475-479. http://dx.doi.org/10.1007/s11373-007-9157-3 [64] J. Nilsson, et al., “The APC/C Maintains the Spindle Assembly Checkpoint by Targeting Cdc20 for Destruc- tion,” Nature Cell Biology, Vol. 10, No. 12, 2008, pp. 1411-1420. http://dx.doi.org/10.1038/ncb1799 [65] W. van Zon and R. M. Wolthuis, “Cyclin A and Nek2A: APC/C-Cdc20 Substrates Invisible to the Mitotic Spindle Checkpoint,” Biochemical Society Transactions, Vol. 38, 2010, pp. 72-77. http://dx.doi.org/10.1042/BST0380072 [66] B. Di Fiore and J. Pines, “How Cyclin A Destruction Es- capes the Spindle Assembly Checkpoint,” Nature Cell Biology, Vol. 190, No. 4, 2010, pp. 501-509. http://dx.doi.org/10.1083/jcb.201001083 [67] R. Wolthuis, et al., “Cdc20 and Cks Direct the Spindle Checkpoint-Independent Destruction of Cyclin A,” Mole- cular Cell, Vol. 30, No. 3, 2008, pp. 290-302. http://dx.doi.org/10.1016/j.molcel.2008.02.027 [68] D. Patra and W. G. Dunphy, “Xe-p9, a Xenopus Suc1/ Cks Protein, Is Essential for the Cdc2-Dependent Phos- phorylation of the Anaphase-Promoting Complex at Mi- tosis,” Genes & Development, Vol. 12, No. 16, 1998, pp. 2549-2559. http://dx.doi.org/10.1101/gad.12.16.2549 [69] D. Patra and W. G. Dunphy, “Xe-p9, a Xenopus Suc1/ Cks Homolog, Has Multiple Essential Roles in Cell Cycle Control,” Genes & Development, Vol. 10, No. 12, 1996, pp. 1503-1515. http://dx.doi.org/10.1101/gad.10.12.1503 Copyright © 2013 SciRes. JCT
 Cks1: Structure, Emerging Roles and Implications in Multiple Cancers 1351 [70] L. Westbrook, et al., “Cks1 Regulates Cdk1 Expression: A Novel Role during Mitotic Entry in Breast Cancer Cells,” Cancer Research, Vol. 67, No. 23, 2007, pp. 11393-11401. http://dx.doi.org/10.1158/0008-5472.CAN-06-4173 [71] H. S. Martinsson-Ahlzen, et al., “Cyclin-Dependent Kina- se-Associated Proteins Cks1 and Cks2 Are Essential dur- ing Early Embryogenesis and for Cell Cycle Progression in Somatic Cells,” Molecular and Cellular Biology, Vol. 28, No. 18, 2008, pp. 5698-5709. http://dx.doi.org/10.1128/MCB.01833-07 [72] Y. S. Tsai, et al., “RNA Silencing of Cks1 Induced G2/M Arrest and Apoptosis in Human Lung Cancer Cells,” IUBMB Life, Vol. 57, No. 8, 2005, pp. 583-589. http://dx.doi.org/10.1080/15216540500215531 [73] A. Hoellein, et al., “Cks1 Promotion of S Phase Entry and Proliferation Is Independent of p27Kip1 Suppression,” Molecular and Cellular Biology, Vol. 32, No. 13, 2012, pp. 2416-2427. http://dx.doi.org/10.1128/MCB.06771-11 [74] S. Chaves, et al., “Cks1, Cdk1, and the 19S Proteasome Collaborate to Regulate Gene Induction-Dependent Nu- cleosome Eviction in Yeast,” Molecular and Cellular Bi- ology, Vol. 30, No. 22, 2010, pp. 5284-5294. http://dx.doi.org/10.1128/MCB.00952-10 [75] R. Holic, et al., “Cks1 Activates Transcription by Bind- ing to the Ubiquitylated Proteasome,” Molecular and Cel- lular Biology, Vol. 30, No. 15, 2010, pp. 3894-3901. http://dx.doi.org/10.1128/MCB.00655-09 [76] M. C. Morris, et al., “Cks1-Dependent Proteasome Re- cruitment and Activation of CDC20 Transcription in Bud- ding Yeast,” Nature, Vol. 423, No. 6943, 2003, pp. 1009- 1013. http://dx.doi.org/10.1038/nature01720 [77] V. P. Yu, et al., “A Kinase-Independent Function of Cks1 and Cdk1 in Regulation of Transcription,” Molecular Cell, Vol. 17, No. 1, 2005, pp. 145-151. http://dx.doi.org/10.1016/j.molcel.2004.11.020 [78] D. J. Baker, et al., “Mitotic Regulation of the Anaphase- Promoting Complex,” Cellular and Molecular Life Sci- ences, Vol. 64, No. 5, 2007, pp. 589-600. http://dx.doi.org/10.1007/s00018-007-6443-1 [79] L. J. Appleman, et al., “CD28 Costimulation Mediates Transcription of SKP2 and CKS1, the substrate Recogni- tion Components of SCFSkp2 Ubiquitin Ligase That Leads p27kip1 to Degradation,” Cell Cycle, Vol. 5, No. 18, 2006, pp. 2123-2129. http://dx.doi.org/10.4161/cc.5.18.3139 [80] M. Dibb, et al., “The FOXM1-PLK1 Axis Is Commonly Upregulated in Oesophageal Adenocarcinoma,” British Journal of Cancer, Vol. 107, No. 10, 2012, pp. 1766- 1775. http://dx.doi.org/10.1038/bjc.2012.424 [81] U. B. Keller, et al., “Myc Targets Cks1 to Provoke the Suppression of p27Kip1, Proliferation and Lymphoma- genesis,” The EMBO Journal, Vol. 26, No. 10, 2007, pp. 2562-2574. http://dx.doi.org/10.1038/sj.emboj.7601691 [82] M. Mourot, et al., “The Influence of Follicle Size, FSH- Enriched Maturation Medium, and Early Cleavage on Bovine Oocyte Maternal mRNA Levels,” Molecular Re- production and Development, Vol. 73, No. 11, 2006, pp. 1367-1379. http://dx.doi.org/10.1002/mrd.20585 [83] V. Petrovic, et al., “FoxM1 Regulates Growth Factor- Induced Expression of Kinase-Interacting Stathmin (KIS) to Promote Cell Cycle Progression,” The Journal of Bio- logical Chemistry, Vol. 283, No. 1, 2008, pp. 453-460. http://dx.doi.org/10.1074/jbc.M705792200 [84] N. Uehara, K. Yoshizawa and A. Tsubura, “Vorinostat Enhances Protein Stability of p27 and p21 through Nega- tive Regulation of Skp2 and Cks1 in Human Breast Can- cer Cells,” Oncology Reports, Vol. 28, No. 1, 2012, pp. 105-110. [85] I. C. Wang, et al., “Forkhead Box M1 Regulates the Tran- scriptional Network of Genes Essential for Mitotic Pro- gression and Genes Encoding the SCF (Skp2-Cks1) Ubi- quitin Ligase,” Molecular and Cellular Biology, Vol. 25, No. 24, 2005, pp. 10875-10894. http://dx.doi.org/10.1128/MCB.25.24.10875-10894.2005 [86] W. Wang, et al., “Negative Regulation of SCFSkp2 Ubi- quitin Ligase by TGF-Beta Signaling,” Oncogene, Vol. Vol. 23, No. 5, 2004, pp. 1064-1075. http://dx.doi.org/10.1038/sj.onc.1207204 [87] K. Rother, et al., “Expression of Cyclin-Dependent Ki- nase Subunit 1 (Cks1) Is Regulated during the Cell Cycle by a CDE/CHR Tandem Element and is Downregulated by p53 but Not by p63 or p73,” Cell Cycle, Vol. 6, No. 7, 2007, pp. 853-862. http://dx.doi.org/10.4161/cc.6.7.4017 [88] T. Bashir, et al., “Control of the SCF(Skp2-Cks1) Ubi- quitin Ligase by the APC/C(Cdh1) Ubiquitin Ligase,” Nature, Vol. 428, No. 6979, 2004, pp. 190-193. http://dx.doi.org/10.1038/nature02330 [89] D. J. Lenschow, T. L. Walunas and J. A. Bluestone, “CD28/B7 System of T Cell Costimulation,” Annual Re- view of Immunology, Vol. 14, 1996, pp. 233-258. http://dx.doi.org/10.1146/annurev.immunol.14.1.233 [90] L. J. Appleman, et al., “CD28 Costimulation Mediates T Cell Expansion via IL-2-Independent and IL-2-Dependent Regulation of Cell Cycle Progression,” The Journal of Immunology, Vol. 164, No. 1, 2000, pp. 144-151. [91] M. Okamura, et al., “The Possible Mechanism of En- hanced Carcinogenesis Induced by Genotoxic Carcino- gens in rasH2 Mice,” Cancer Letters, Vol. 245, No. 1-2, 2007, pp. 321-330. http://dx.doi.org/10.1016/j.canlet.2006.01.025 [92] E. S. Polinko and S. Strome, “Depletion of a Cks Homo- log in C. Elegans Embryos Uncovers a Post-Metaphase Role in Both Meiosis and Mitosis,” Current Biology, Vol. 10, No. 22, 2000, p. 1471-1474. http://dx.doi.org/10.1016/S0960-9822(00)00808-3 [93] M. Ghorbani, et al., “Cks85A and Skp2 Interact to Main- tain Diploidy and Promote Growth in Drosophila,” De- velopmental Biology, Vol. 358, No. 1, 2011, pp. 213-223. http://dx.doi.org/10.1016/j.ydbio.2011.07.031 [94] H. Yang, et al., “Identification and Expression of the Amphioxus Cks1 Gene,” Cell Biology International, Vol. 29, No. 7, 2005, pp. 593-597. http://dx.doi.org/10.1016/j.cellbi.2005.03.012 [95] J. C. Mottram and K. M. Grant, “Leishmania Mexicana p12cks1, a Homologue of Fission Yeast p13suc1, Asso- ciates with a Stage-Regulated Histone H1 Kinase,” Bio- chemical Journal, Vol. 316 , 1996, pp. 833-839. Copyright © 2013 SciRes. JCT
 Cks1: Structure, Emerging Roles and Implications in Multiple Cancers 1352 [96] P. Colas, F. Serras and A. E. Van Loon, “Microinjection of Suc1 Transcripts Delays the Cell Cycle Clock in Pa- tella Vulgata Embryos,” The International Journal of De- velopmental Biology, Vol. 37, No. 4, 1993, pp. 589-594. [97] V. Boudolf, et al., “Identification of Novel Cyclin-De- pendent Kinases Interacting with the Cks1 Protein of Ara- bidopsis,” Journal of Experimental Botany, Vol. 52, No. 359, 2001, pp. 1381-1382. http://dx.doi.org/10.1093/jexbot/52.359.1381 [98] L. De Veylder, et al., “The Arabidopsis Cks1At Protein Binds the Cyclin-Dependent Kinases Cdc2aAt and Cdc2bAt,” FEBS Letters, Vol. 412, No. 3, 1997, pp. 446- 452. http://dx.doi.org/10.1016/S0014-5793(97)00822-3 [99] V. L. De, et al., “CKS1At Overexpression in Arabidopsis thaliana Inhibits Growth by Reducing Meristem Size and Inhibiting Cell-Cycle Progression,” The Plant Journal, Vol. 25, No. 6, 2001, pp. 617-626. [100] K. M. Grant, et al., “The Crk3 Gene of Leishmania Mexi- cana Encodes a Stage-Regulated cdc2-Related Histone H1 Kinase That Associates with p12,” The Journal of Biological Chemistry, Vol. 273, No. 17, 1998, pp. 10153- 10159. http://dx.doi.org/10.1074/jbc.273.17.10153 [101] N. J. Pearson, et al., “A Pre-Anaphase Role for a Cks/ Suc1 in Acentrosomal Spindle Formation of Drosophila Female Meiosis,” EMBO Reports, Vol. 6, No. 11, 2005, pp. 1058-1063. http://dx.doi.org/10.1038/sj.embor.7400529 [102] A. Swan, G. Barcelo and T. Schupbach, “Drosophila Cks30A Interacts with Cdk1 to Target Cyclin A for De- struction in the Female Germline,” Development, Vol. 132, No. 16, 2005, pp. 3669-3678. http://dx.doi.org/10.1242/dev.01940 [103] A. Swan and T. Schupbach, “Drosophila Female Meiosis and Embryonic Syncytial Mitosis Use Specialized Cks and CDC20 Proteins for Cyclin Destruction,” Cell Cycle, Vol. 4, No. 10, 2005, pp. 1332-1334. http://dx.doi.org/10.4161/cc.4.10.2088 [104] C. A. Auld, K. M. Fernandes and R. F. Morrison, “Skp2- Mediated p27(Kip1) Degradation during S/G2 Phase Progression of Adipocyte Hyperplasia,” Journal of Cel- lular Physiology, Vol. 211, No. 1, 2007, pp. 101-111. http://dx.doi.org/10.1002/jcp.20915 [105] M. Radulovic, et al., “CKS Proteins Protect Mitochon- drial Genome Integrity by Interacting with Mitochondrial Single-Stranded DNA-Binding Protein,” Molecular & Cellular Proteomics, Vol. 9, No. 1, 2010, pp. 145-152. http://dx.doi.org/10.1074/mcp.M900078-MCP200 [106] R. M. Nagler, et al., “The Expression and Prognostic Significance of Cks1 in Salivary Cancer,” Cancer Inves- tigation, Vol. 27, No. 5, 2009, pp. 512-520. http://dx.doi.org/10.1080/07357900802239116 [107] M. Shapira, et al., “Alterations in the Expression of the Cell Cycle Regulatory Protein Cyclin Kinase Subunit 1 in Colorectal Carcinoma,” Cancer, Vol. 100, No. 8, 2004, pp. 1615-1621. http://dx.doi.org/10.1002/cncr.20172 [108] D. D. Hershko and M. Shapira, “Prognostic Role of p27Kip1 Deregulation in Colorectal Cancer,” Cancer, Vol. 107, No. 4, 2006, pp. 668-675. http://dx.doi.org/10.1002/cncr.22073 [109] M. Slotky, et al., “The Expression of the Ubiquitin Ligase Subunit Cks1 in Human Breast Cancer,” Breast Cancer Research, Vol. 7, No. 5, 2005, pp. R737-R744. http://dx.doi.org/10.1186/bcr1278 [110] L. Westbrook, et al., “High Cks1 Expression in Trans- genic and Carcinogen-Initiated Mammary Tumors Is Not Always Accompanied by Reduction in p27Kip1,” Inter- national Journal of Oncology, Vol. 34, No. 5, 2009, pp. 1425-1431. [111] N. Inui, et al., “High Expression of Cks1 in Human Non- Small Cell Lung Carcinomas,” Biochemical and Biophy- sical Research Communications, Vol. 303, No. 3, 2003, p. 978-984. http://dx.doi.org/10.1016/S0006-291X(03)00469-8 [112] S. Fredersdorf, et al., “High Level Expression of p27 (kip1) and Cyclin D1 in Some Human Breast Cancer Cells: Inverse Correlation between the Expression of p27 (kip1) and Degree of malignancy in human breast and colorectal cancers,” Proceedings of the National Academy of Sciences of the United States of America, Vol. 94, No. 12, 1997, pp. 6380-6385. http://dx.doi.org/10.1073/pnas.94.12.6380 [113] T. H. Qiu, et al., “Global Expression Profiling Identifies Signatures of Tumor Virulence in MMTV-PyMT-Trans- genic Mice: Correlation to Human Disease,” Cancer Re- search, Vol. 64, No. 17, 2004, pp. 5973-5981. http://dx.doi.org/10.1158/0008-5472.CAN-04-0242 [114] Y. Hu, et al., “From Mice to Humans: Identification of Commonly Deregulated Genes in Mammary Cancer via Comparative SAGE Studies,” Cancer Research, Vol. 64, No. 21, 2004, pp. 7748-7755. http://dx.doi.org/10.1158/0008-5472.CAN-04-1827 [115] S. Signoretti, et al., “Oncogenic Role of the Ubiquitin Ligase Subunit Skp2 in Human Breast Cancer,” Journal of Clinical Investigation, Vol. 110, No. 5, 2002, pp. 633- 641. [116] A. Krishnan, et al., “Fluoxetine Mediates G0/G1 Arrest by Inducing Functional Inhibition of Cyclin Dependent Kinase Subunit (CKS)1,” Biochemical Pharmacology, Vol. 75, No. 10, 2008, pp. 1924-1934. http://dx.doi.org/10.1016/j.bcp.2008.02.013 [117] J. Jiang and D. Sliva, “Novel Medicinal Mushroom Blend Suppresses Growth and Invasiveness of Human Breast Cancer Cells,” International Journal of Oncology, Vol. 37, No. 6, 2010, pp. 1529-1536. [118] E. Rico-Bautista, et al., “Chemical Genetics Approach to Restoring p27Kip1 Reveals Novel Compounds with Anti- proliferative Activity in Prostate Cancer Cells,” BMC Bi- ology, Vol. 8, 2010, p. 153. http://dx.doi.org/10.1186/1741-7007-8-153 [119] E. Rico-Bautista and D. A. Wolf, “Skipping Cancer: Small Molecule Inhibitors of SKP2-Mediated p27 Deg- radation,” Chemistry & Biology, Vol. 19, No. 12, 2012, pp. 1497-1498. http://dx.doi.org/10.1016/j.chembiol.2012.12.001 [120] L. Wu, et al., “Specific Small Molecule Inhibitors of Skp2-Mediated p27 Degradation,” Chemistry & Biology, Vol. 19, No. 12, 2012, pp. 1515-1524. http://dx.doi.org/10.1016/j.chembiol.2012.09.015 Copyright © 2013 SciRes. JCT
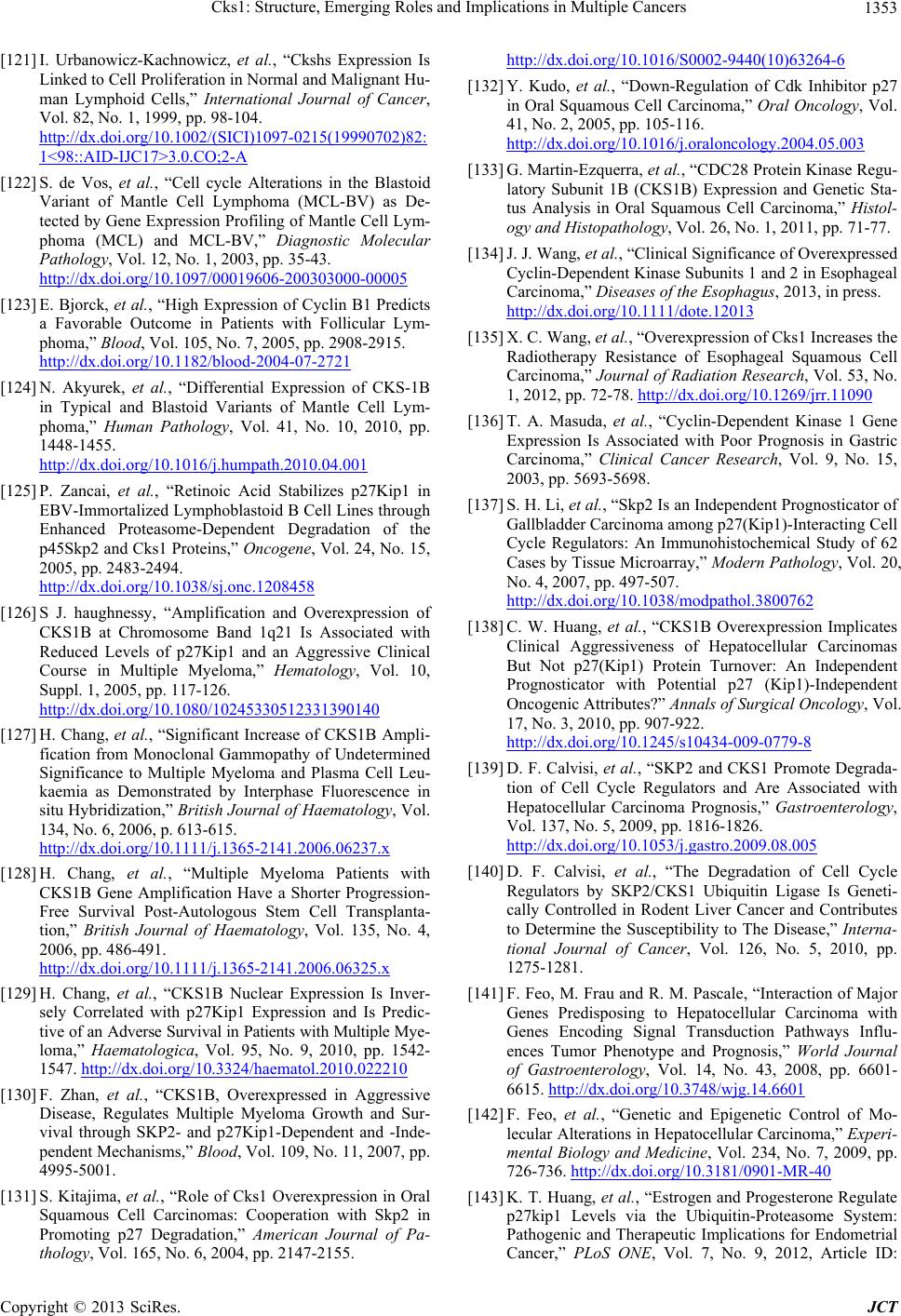 Cks1: Structure, Emerging Roles and Implications in Multiple Cancers 1353 [121] I. Urbanowicz-Kachnowicz, et al., “Ckshs Expression Is Linked to Cell Proliferation in Normal and Malignant Hu- man Lymphoid Cells,” International Journal of Cancer, Vol. 82, No. 1, 1999, pp. 98-104. http://dx.doi.org/10.1002/(SICI)1097-0215(19990702)82: 1<98::AID-IJC17>3.0.CO;2-A [122] S. de Vos, et al., “Cell cycle Alterations in the Blastoid Variant of Mantle Cell Lymphoma (MCL-BV) as De- tected by Gene Expression Profiling of Mantle Cell Lym- phoma (MCL) and MCL-BV,” Diagnostic Molecular Pathology, Vol. 12, No. 1, 2003, pp. 35-43. http://dx.doi.org/10.1097/00019606-200303000-00005 [123] E. Bjorck, et al., “High Expression of Cyclin B1 Predicts a Favorable Outcome in Patients with Follicular Lym- phoma,” Blood, Vol. 105, No. 7, 2005, pp. 2908-2915. http://dx.doi.org/10.1182/blood-2004-07-2721 [124] N. Akyurek, et al., “Differential Expression of CKS-1B in Typical and Blastoid Variants of Mantle Cell Lym- phoma,” Human Pathology, Vol. 41, No. 10, 2010, pp. 1448-1455. http://dx.doi.org/10.1016/j.humpath.2010.04.001 [125] P. Zancai, et al., “Retinoic Acid Stabilizes p27Kip1 in EBV-Immortalized Lymphoblastoid B Cell Lines through Enhanced Proteasome-Dependent Degradation of the p45Skp2 and Cks1 Proteins,” Oncogene, Vol. 24, No. 15, 2005, pp. 2483-2494. http://dx.doi.org/10.1038/sj.onc.1208458 [126] S J. haughnessy, “Amplification and Overexpression of CKS1B at Chromosome Band 1q21 Is Associated with Reduced Levels of p27Kip1 and an Aggressive Clinical Course in Multiple Myeloma,” Hematology, Vol. 10, Suppl. 1, 2005, pp. 117-126. http://dx.doi.org/10.1080/10245330512331390140 [127] H. Chang, et al., “Significant Increase of CKS1B Ampli- fication from Monoclonal Gammopathy of Undetermined Significance to Multiple Myeloma and Plasma Cell Leu- kaemia as Demonstrated by Interphase Fluorescence in situ Hybridization,” British Journal of Haematology, Vol. 134, No. 6, 2006, p. 613-615. http://dx.doi.org/10.1111/j.1365-2141.2006.06237.x [128] H. Chang, et al., “Multiple Myeloma Patients with CKS1B Gene Amplification Have a Shorter Progression- Free Survival Post-Autologous Stem Cell Transplanta- tion,” British Journal of Haematology, Vol. 135, No. 4, 2006, pp. 486-491. http://dx.doi.org/10.1111/j.1365-2141.2006.06325.x [129] H. Chang, et al., “CKS1B Nuclear Expression Is Inver- sely Correlated with p27Kip1 Expression and Is Predic- tive of an Adverse Survival in Patients with Multiple Mye- loma,” Haematologica, Vol. 95, No. 9, 2010, pp. 1542- 1547. http://dx.doi.org/10.3324/haematol.2010.022210 [130] F. Zhan, et al., “CKS1B, Overexpressed in Aggressive Disease, Regulates Multiple Myeloma Growth and Sur- vival through SKP2- and p27Kip1-Dependent and -Inde- pendent Mechanisms,” Blood, Vol. 109, No. 11, 2007, pp. 4995-5001. [131] S. Kitajima, et al., “Role of Cks1 Overexpression in Oral Squamous Cell Carcinomas: Cooperation with Skp2 in Promoting p27 Degradation,” American Journal of Pa- thology, Vol. 165, No. 6, 2004, pp. 2147-2155. http://dx.doi.org/10.1016/S0002-9440(10)63264-6 [132] Y. Kudo, et al., “Down-Regulation of Cdk Inhibitor p27 in Oral Squamous Cell Carcinoma,” Oral Oncology, Vol. 41, No. 2, 2005, pp. 105-116. http://dx.doi.org/10.1016/j.oraloncology.2004.05.003 [133] G. Martin-Ezquerra, et al., “CDC28 Protein Kinase Regu- latory Subunit 1B (CKS1B) Expression and Genetic Sta- tus Analysis in Oral Squamous Cell Carcinoma,” Histol- ogy and Histopathology, Vol. 26, No. 1, 2011, pp. 71-77. [134] J. J. Wang, et al., “Clinical Significance of Overexpressed Cyclin-Dependent Kinase Subunits 1 and 2 in Esophageal Carcinoma,” Diseases of the Esophagus, 2013, in press. http://dx.doi.org/10.1111/dote.12013 [135] X. C. Wang, et al., “Overexpression of Cks1 Increases the Radiotherapy Resistance of Esophageal Squamous Cell Carcinoma,” Journal of Radiation Research, Vol. 53, No. 1, 2012, pp. 72-78. http://dx.doi.org/10.1269/jrr.11090 [136] T. A. Masuda, et al., “Cyclin-Dependent Kinase 1 Gene Expression Is Associated with Poor Prognosis in Gastric Carcinoma,” Clinical Cancer Research, Vol. 9, No. 15, 2003, pp. 5693-5698. [137] S. H. Li, et al., “Skp2 Is an Independent Prognosticator of Gallbladder Carcinoma among p27(Kip1)-Interacting Cell Cycle Regulators: An Immunohistochemical Study of 62 Cases by Tissue Microarray,” Modern Pathology, Vol. 20, No. 4, 2007, pp. 497-507. http://dx.doi.org/10.1038/modpathol.3800762 [138] C. W. Huang, et al., “CKS1B Overexpression Implicates Clinical Aggressiveness of Hepatocellular Carcinomas But Not p27(Kip1) Protein Turnover: An Independent Prognosticator with Potential p27 (Kip1)-Independent Oncogenic Attributes?” Annals of Surgical Oncology, Vol. 17, No. 3, 2010, pp. 907-922. http://dx.doi.org/10.1245/s10434-009-0779-8 [139] D. F. Calvisi, et al., “SKP2 and CKS1 Promote Degrada- tion of Cell Cycle Regulators and Are Associated with Hepatocellular Carcinoma Prognosis,” Gastroenterology, Vol. 137, No. 5, 2009, pp. 1816-1826. http://dx.doi.org/10.1053/j.gastro.2009.08.005 [140] D. F. Calvisi, et al., “The Degradation of Cell Cycle Regulators by SKP2/CKS1 Ubiquitin Ligase Is Geneti- cally Controlled in Rodent Liver Cancer and Contributes to Determine the Susceptibility to The Disease,” Interna- tional Journal of Cancer, Vol. 126, No. 5, 2010, pp. 1275-1281. [141] F. Feo, M. Frau and R. M. Pascale, “Interaction of Major Genes Predisposing to Hepatocellular Carcinoma with Genes Encoding Signal Transduction Pathways Influ- ences Tumor Phenotype and Prognosis,” World Journal of Gastroenterology, Vol. 14, No. 43, 2008, pp. 6601- 6615. http://dx.doi.org/10.3748/wjg.14.6601 [142] F. Feo, et al., “Genetic and Epigenetic Control of Mo- lecular Alterations in Hepatocellular Carcinoma,” Experi- mental Biology and Medicine, Vol. 234, No. 7, 2009, pp. 726-736. http://dx.doi.org/10.3181/0901-MR-40 [143] K. T. Huang, et al., “Estrogen and Progesterone Regulate p27kip1 Levels via the Ubiquitin-Proteasome System: Pathogenic and Therapeutic Implications for Endometrial Cancer,” PLoS ONE, Vol. 7, No. 9, 2012, Article ID: Copyright © 2013 SciRes. JCT
 Cks1: Structure, Emerging Roles and Implications in Multiple Cancers Copyright © 2013 SciRes. JCT 1354 e46072. http://dx.doi.org/10.1371/journal.pone.0046072 [144] V. Ouellet, et al., “Discrimination between Serous Low Malignant Potential and Invasive Epithelial Ovarian Tu- mors Using Molecular Profiling,” Oncogene, Vol. 24, No. 29, 2005, pp. 4672-4687. http://dx.doi.org/10.1038/sj.onc.1208214 [145] V. Ouellet, et al., “Tissue Array Analysis of Expression Microarray Candidates Identifies Markers Associated with Tumor Grade and Outcome in Serous Epithelial Ova- rian Cancer,” International Journal of Cancer, Vol. 119, No. 3, 2006, pp. 599-607. http://dx.doi.org/10.1002/ijc.21902 [146] S. Yamamoto, et al., “Cumulative Alterations of p27-Re- lated Cell-Cycle Regulators in the Development of En- dometriosis-Associated Ovarian Clear Cell Adenocarci- noma,” Histopathology, Vol. 56, No. 6, 2010, pp. 740- 749. http://dx.doi.org/10.1111/j.1365-2559.2010.03551.x [147] S. Yamamoto, et al., “Aberrant Expression of p27(Kip1)- Interacting Cell-Cycle Regulatory Proteins in Ovarian Clear Cell Carcinomas and Their Precursors with Special Consideration of Two Distinct Multistage Clear Cell Car- cinogenetic Pathways,” Virchows Arch, Vol. 455, No. 5, 2009, pp. 413-422. http://dx.doi.org/10.1007/s00428-009-0844-5 [148] Y. Lan, et al., “Aberrant Expression of Cks1 and Cks2 Contributes to Prostate Tumorigenesis by Promoting Pro- liferation and Inhibiting Programmed Cell Death,” Inter- national Journal of Cancer, Vol. 123, No. 3, 2008, pp. 543-551. http://dx.doi.org/10.1002/ijc.23548 [149] K. Miyai, et al., “Altered Expression of p27(Kip1)-Inter- Acting Cell-Cycle Regulators in the Adult Testicular Germ Cell Tumors: Potential Role in Tumor Develop- ment and Histological Progression,” APMIS, Vol. 120, No. 11, 2012, pp. 890-900. http://dx.doi.org/10.1111/j.1600-0463.2012.02919.x [150] V. G. Zolota, et al., “Histologic-Type Specific Role of Cell Cycle Regulators in Non-Small Cell Lung Carci- noma,” Journal of Surgical Research, 164, No. 2, 2010, pp. 256-265. http://dx.doi.org/10.1016/j.jss.2009.03.035 [151] R. Salgado, et al., “CKS1B Amplification Is a Frequent Event in Cutaneous Squamous Cell Carcinoma with Ag- gressive Clinical Behaviour,” Genes, Chromosomes and Cancer, Vol. 49, No. 11, 2010, pp. 1054-1061. http://dx.doi.org/10.1002/gcc.20814 [152] K. Kawakami, et al., “Increased SKP2 and CKS1 Gene Expression Contributes to the Progression of Human urothelial Carcinoma,” Journal of Urology, Vol. 178, No. 1, 2007, pp. 301-307. http://dx.doi.org/10.1016/j.juro.2007.03.002 [153] Z. Liu, et al., “Prognostic Implication of p27Kip1, Skp2 and Cks1 Expression in Renal Cell Carcinoma: A Tissue Microarray Study,” Journal of Experimental & Clinical Cancer Research, Vol. 27, 2008, p. 51. http://dx.doi.org/10.1186/1756-9966-27-51 [154] D. M. Kokkinakis, et al., “Modulation of Gene Expres- sion in Human Central Nervous System Tumors under Methionine Deprivation-Induced Stress,” Cancer Re- search, Vol. 64, No. 20, 2004, pp. 7513-7525. http://dx.doi.org/10.1158/0008-5472.CAN-04-0592 [155] H. Halfter, et al., “Oncostatin M Induces Growth Arrest by Inhibition of Skp2, Cks1, and Cyclin A Expression and Induced p21 Expression,” Cancer Research, Vol. 66, No. 13, 2006, pp. 6530-6539. http://dx.doi.org/10.1158/0008-5472.CAN-04-3734 [156] R. Lin, et al., “Inhibition of F-Box Protein p45(SKP2) Expression and Stabilization of Cyclin-Dependent Kinase Inhibitor p27(KIP1) in Vitamin D Analog-Treated Cancer Cells,” Endocrinology, Vol. 144, No. 3, 2003, pp. 749- 753. http://dx.doi.org/10.1210/en.2002-0026 [157] D. M. Gascoyne, et al., “Loss of Mitotic Spindle Check- point Activity Predisposes to Chromosomal Instability at Early Stages of Fibrosarcoma Development,” Cell Cycle, Vol. 2, No. 3, 2003, pp. 238-245. http://dx.doi.org/10.4161/cc.2.3.355 [158] H. Y. Huang, et al., “Skp2 Overexpression Is Highly Representative of Intrinsic Biological Aggressiveness and Independently Associated with Poor Prognosis in Primary Localized Myxofibrosarcomas,” Clinical Cancer Re- search, Vol. 12, No. 2, 2006, pp. 487-498. http://dx.doi.org/10.1158/1078-0432.CCR-05-1497
|