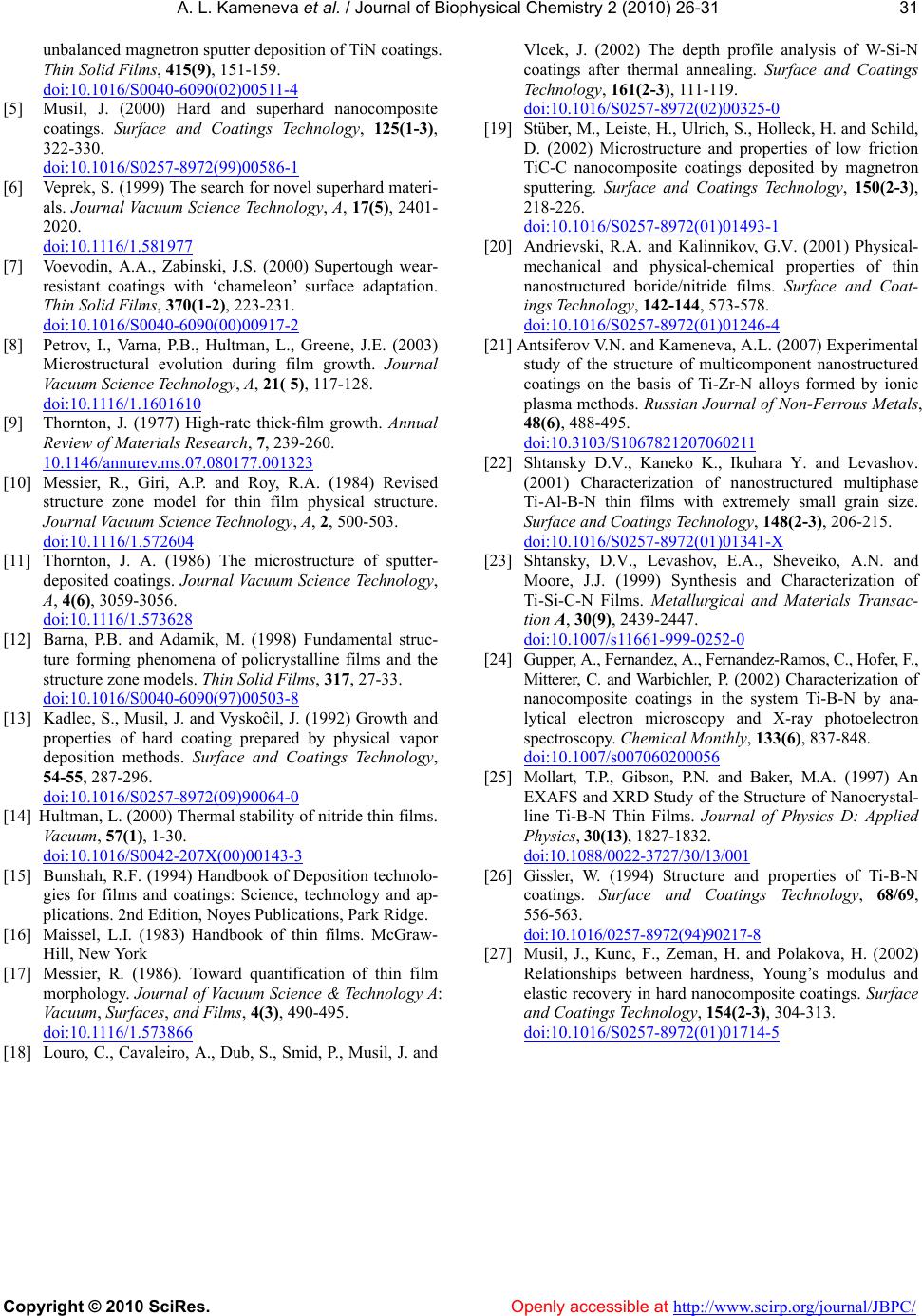
A. L. Kameneva et al. / Journal of Biophysical Chemistry 2 (2010) 26-31
Copyright © 2010 SciRes. Openly accessible at http://www.scirp.org/journal/JBPC/
31
unbalanced magnetron sputter deposition of TiN coatings.
Thin Solid Films, 415(9), 151-159.
doi:10.1016/S0040-6090(02)00511-4
[5] Musil, J. (2000) Hard and superhard nanocomposite
coatings. Surface and Coatings Technology, 125(1-3),
322-330.
doi:10.1016/S0257-8972(99)00586-1
[6] Veprek, S. (1999) The search for novel superhard materi-
als. Journal Vacuum Science Technology, A, 17(5), 2401-
2020.
doi:10.1116/1.581977
[7] Voevodin, A.A., Zabinski, J.S. (2000) Supertough wear-
resistant coatings with ‘chameleon’ surface adaptation.
Thin Solid Films, 370(1-2), 223-231.
doi:10.1016/S0040-6090(00)00917-2
[8] Petrov, I., Varna, P.B., Hultman, L., Greene, J.E. (2003)
Microstructural evolution during film growth. Journal
Vacuum Science Technology, A, 21( 5), 117-128.
doi:10.1116/1.1601610
[9] Thornton, J. (1977) High-rate thick-film growth. Annual
Review of Materials Research, 7, 239-260.
10.1146/annurev.ms.07.080177.001323
[10] Messier, R., Giri, A.P. and Roy, R.A. (1984) Revised
structure zone model for thin film physical structure.
Journal Vacuum Science Technolog y, A, 2, 500-503.
doi:10.1116/1.572604
[11] Thornton, J. A. (1986) The microstructure of sputter-
deposited coatings. Journal Vacuum Science Technology,
A, 4(6), 3059-3056.
doi:10.1116/1.573628
[12] Barna, P.B. and Adamik, M. (1998) Fundamental struc-
ture forming phenomena of policrystalline films and the
structure zone models. Thin Solid Films, 317, 27-33.
doi:10.1016/S0040-6090(97)00503-8
[13] Kadlec, S., Musil, J. and Vyskoĉil, J. (1992) Growth and
properties of hard coating prepared by physical vapor
deposition methods. Surface and Coatings Technology,
54-55, 287-296.
doi:10.1016/S0257-8972(09)90064-0
[14] Hultman, L. (2000) Thermal stability of nitride thin films.
Vacuum, 57(1), 1-30.
doi:10.1016/S0042-207X(00)00143-3
[15] Bunshah, R.F. (1994) Handbook of Deposition technolo-
gies for films and coatings: Science, technology and ap-
plications. 2nd Edition, Noyes Publications, Park Ridge.
[16] Maissel, L.I. (1983) Handbook of thin films. McGraw-
Hill, New York
[17] Messier, R. (1986). Toward quantification of thin film
morphology. Journal of Vacuum Science & Technology A:
Vacuum, Surfaces, and Films, 4(3), 490-495.
doi:10.1116/1.573866
[18] Louro, C., Cavaleiro, A., Dub, S., Smid, P., Musil, J. and
Vlcek, J. (2002) The depth profile analysis of W-Si-N
coatings after thermal annealing. Surface and Coatings
Technology, 161(2-3), 111-119.
doi:10.1016/S0257-8972(02)00325-0
[19] Stüber, M., Leiste, H., Ulrich, S., Holleck, H. and Schild,
D. (2002) Microstructure and properties of low friction
TiC-C nanocomposite coatings deposited by magnetron
sputtering. Surface and Coatings Technology, 150(2-3),
218-226.
doi:10.1016/S0257-8972(01)01493-1
[20] Andrievski, R.A. and Kalinnikov, G.V. (2001) Physical-
mechanical and physical-chemical properties of thin
nanostructured boride/nitride films. Surface and Coat-
ings Tech nology, 142-144, 573-578.
doi:10.1016/S0257-8972(01)01246-4
[21] Antsiferov V.N. and Kameneva, A.L. (2007) Experimental
study of the structure of multicomponent nanostructured
coatings on the basis of Ti-Zr-N alloys formed by ionic
plasma methods. Russian Journal of Non-Ferrous Metals,
48(6), 488-495.
doi:10.3103/S1067821207060211
[22] Shtansky D.V., Kaneko K., Ikuhara Y. and Levashov.
(2001) Characterization of nanostructured multiphase
Ti-Al-B-N thin films with extremely small grain size.
Surface and Coatings Technology, 148(2-3), 206-215.
doi:10.1016/S0257-8972(01)01341-X
[23] Shtansky, D.V., Levashov, E.A., Sheveiko, A.N. and
Moore, J.J. (1999) Synthesis and Characterization of
Ti-Si-C-N Films. Metallurgical and Materials Transac-
tion A, 30(9), 2439-2447.
doi:10.1007/s11661-999-0252-0
[24] Gupper, A., Fernandez, A., Fernandez-Ramos, С., Hofer, F.,
Mitterer, С. and Warbichler, P. (2002) Characterization of
nanocomposite coatings in the system Ti-B-N by ana-
lytical electron microscopy and X-ray photoelectron
spectroscopy. Chemical Monthly, 133(6), 837-848.
doi:10.1007/s007060200056
[25] Mollart, T.P., Gibson, P.N. and Baker, M.A. (1997) An
EXAFS and XRD Study of the Structure of Nanocrystal-
line Ti-B-N Thin Films. Journal of Physics D: Applied
Physics, 30(13), 1827-1832.
doi:10.1088/0022-3727/30/13/001
[26] Gissler, W. (1994) Structure and properties of Ti-B-N
coatings. Surface and Coatings Technology, 68 /69,
556-563.
doi:10.1016/0257-8972(94)90217-8
[27] Musil, J., Kunc, F., Zeman, H. and Polakova, H. (2002)
Relationships between hardness, Young’s modulus and
elastic recovery in hard nanocomposite coatings. Surface
and Coatings Technology, 154(2-3), 304-313.
doi:10.1016/S0257-8972(01)01714-5