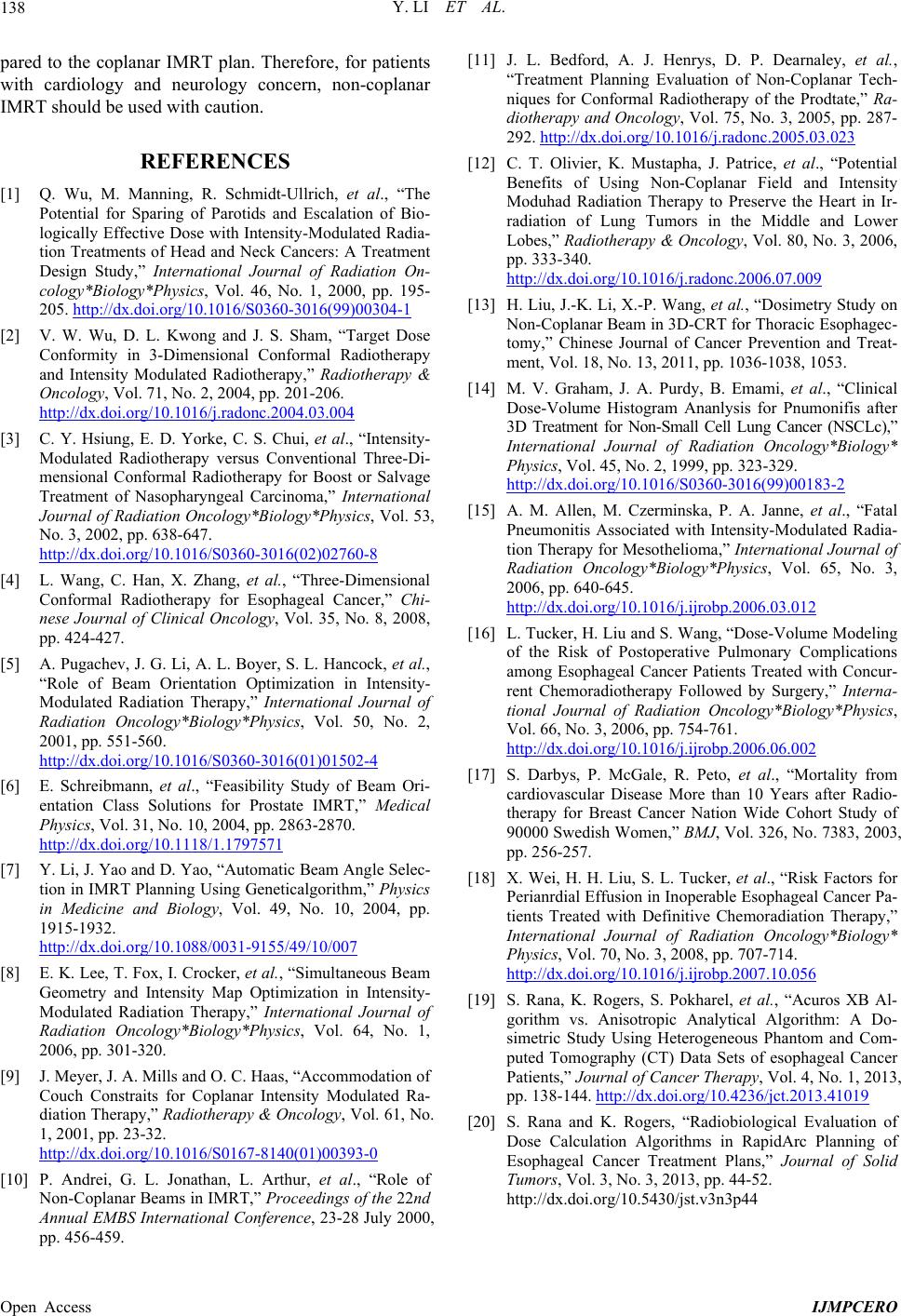
Y. LI ET AL.
Open Access IJMPCERO
138
pared to the coplanar IMRT plan. Therefore, for patients
with cardiology and neurology concern, non-coplanar
IMRT should be used with caution.
REFERENCES
[1] Q. Wu, M. Manning, R. Schmidt-Ullrich, et al., “The
Potential for Sparing of Parotids and Escalation of Bio-
logically Effective Dose with Intensity-Modulated Radia-
tion Treatments of Head and Neck Cancers: A Treatment
Design Study,” International Journal of Radiation On-
colo gy*Biol ogy*Physi cs, Vol. 46, No. 1, 2000, pp. 195-
205. http://dx.doi.org/10.1016/S0360-3016(99)00304-1
[2] V. W. Wu, D. L. Kwong and J. S. Sham, “Target Dose
Conformity in 3-Dimensional Conformal Radiotherapy
and Intensity Modulated Radiotherapy,” Radiotherapy &
Oncology, Vol. 71, No. 2, 2004, pp. 201-206.
http://dx.doi.org/10.1016/j.radonc.2004.03.004
[3] C. Y. Hsiung, E. D. Yorke, C. S. Chui, et al., “Intensity-
Modulated Radiotherapy versus Conventional Three-Di-
mensional Conformal Radiotherapy for Boost or Salvage
Treatment of Nasopharyngeal Carcinoma,” International
Journal of Radiation Oncology*Biology*Physics, Vol. 53,
No. 3, 2002, pp. 638-647.
http://dx.doi.org/10.1016/S0360-3016(02)02760-8
[4] L. Wang, C. Han, X. Zhang, et al., “Three-Dimensional
Conformal Radiotherapy for Esophageal Cancer,” Chi-
nese Journal of Clinical Oncology, Vol. 35, No. 8, 2008,
pp. 424-427.
[5] A. Pugachev, J. G. Li, A. L. Boyer, S. L. Hancock, et al.,
“Role of Beam Orientation Optimization in Intensity-
Modulated Radiation Therapy,” International Journal of
Radiation Oncology*Biology*Physics, Vol. 50, No. 2,
2001, pp. 551-560.
http://dx.doi.org/10.1016/S0360-3016(01)01502-4
[6] E. Schreibmann, et al., “Feasibility Study of Beam Ori-
entation Class Solutions for Prostate IMRT,” Medical
Physics, Vol. 31, No. 10, 2004, pp. 2863-2870.
http://dx.doi.org/10.1118/1.1797571
[7] Y. Li, J. Yao and D. Yao, “Automatic Beam Angle Selec-
tion in IMRT Planning Using Geneticalgorithm,” Physics
in Medicine and Biology, Vol. 49, No. 10, 2004, pp.
1915-1932.
http://dx.doi.org/10.1088/0031-9155/49/10/007
[8] E. K. Lee, T. Fox, I. Crocker, et al., “Simultaneous Beam
Geometry and Intensity Map Optimization in Intensity-
Modulated Radiation Therapy,” International Journal of
Radiation Oncology*Biology*Physics, Vol. 64, No. 1,
2006, pp. 301-320.
[9] J. Meyer, J. A. Mills and O. C. Haas, “Accommodation of
Couch Constraits for Coplanar Intensity Modulated Ra-
diation Therapy,” Radiotherapy & Oncology, Vol. 61, No.
1, 2001, pp. 23-32.
http://dx.doi.org/10.1016/S0167-8140(01)00393-0
[10] P. Andrei, G. L. Jonathan, L. Arthur, et al., “Role of
Non-Coplanar Beams in IMRT,” Proceedings of the 22nd
Annual EMBS International Conference, 23-28 July 2000,
pp. 456-459.
[11] J. L. Bedford, A. J. Henrys, D. P. Dearnaley, et al.,
“Treatment Planning Evaluation of Non-Coplanar Tech-
niques for Conformal Radiotherapy of the Prodtate,” Ra-
diotherapy and Oncology, Vol. 75, No. 3, 2005, pp. 287-
292. http://dx.doi.org/10.1016/j.radonc.2005.03.023
[12] C. T. Olivier, K. Mustapha, J. Patrice, et al., “Potential
Benefits of Using Non-Coplanar Field and Intensity
Moduhad Radiation Therapy to Preserve the Heart in Ir-
radiation of Lung Tumors in the Middle and Lower
Lobes,” Radiotherapy & Oncology, Vol. 80, No. 3, 2006,
pp. 333-340.
http://dx.doi.org/10.1016/j.radonc.2006.07.009
[13] H. Liu, J.-K. Li, X.-P. Wang, et al., “Dosimetry Study on
Non-Coplanar Beam in 3D-CRT for Thoracic Esophagec-
tomy,” Chinese Journal of Cancer Prevention and Treat-
ment, Vol. 18, No. 13, 2011, pp. 1036-1038, 1053.
[14] M. V. Graham, J. A. Purdy, B. Emami, et al., “Clinical
Dose-Volume Histogram Ananlysis for Pnumonifis after
3D Treatment for Non-Small Cell Lung Cancer (NSCLc),”
International Journal of Radiation Oncology*Biology*
Physics, Vol. 45, No. 2, 1999, pp. 323-329.
http://dx.doi.org/10.1016/S0360-3016(99)00183-2
[15] A. M. Allen, M. Czerminska, P. A. Janne, et al., “Fatal
Pneumonitis Associated with Intensity-Modulated Radia-
tion Therapy for Mesothelioma,” International Journal of
Radiation Oncology*Biology*Physics, Vol. 65, No. 3,
2006, pp. 640-645.
http://dx.doi.org/10.1016/j.ijrobp.2006.03.012
[16] L. Tucker, H. Liu and S. Wang, “Dose-Volume Modeling
of the Risk of Postoperative Pulmonary Complications
among Esophageal Cancer Patients Treated with Concur-
rent Chemoradiotherapy Followed by Surgery,” Interna-
tional Journal of Radiation Oncology*Biology*Physics,
Vol. 66, No. 3, 2006, pp. 754-761.
http://dx.doi.org/10.1016/j.ijrobp.2006.06.002
[17] S. Darbys, P. McGale, R. Peto, et al., “Mortality from
cardiovascular Disease More than 10 Years after Radio-
therapy for Breast Cancer Nation Wide Cohort Study of
90000 Swedish Women,” BMJ, Vol. 326, No. 7383, 2003,
pp. 256-257.
[18] X. Wei, H. H. Liu, S. L. Tucker, et al., “Risk Factors for
Perianrdial Effusion in Inoperable Esophageal Cancer Pa-
tients Treated with Definitive Chemoradiation Therapy,”
International Journal of Radiation Oncology*Biology*
Physics, Vol. 70, No. 3, 2008, pp. 707-714.
http://dx.doi.org/10.1016/j.ijrobp.2007.10.056
[19] S. Rana, K. Rogers, S. Pokharel, et al., “Acuros XB Al-
gorithm vs. Anisotropic Analytical Algorithm: A Do-
simetric Study Using Heterogeneous Phantom and Com-
puted Tomography (CT) Data Sets of esophageal Cancer
Patients,” Journal of Cancer Therapy, Vol. 4, No. 1, 2013,
pp. 138-144. http://dx.doi.org/10.4236/jct.2013.41019
[20] S. Rana and K. Rogers, “Radiobiological Evaluation of
Dose Calculation Algorithms in RapidArc Planning of
Esophageal Cancer Treatment Plans,” Journal of Solid
Tumors, Vol. 3, No. 3, 2013, pp. 44-52.
http://dx.doi.org/10.5430/jst.v3n3p44