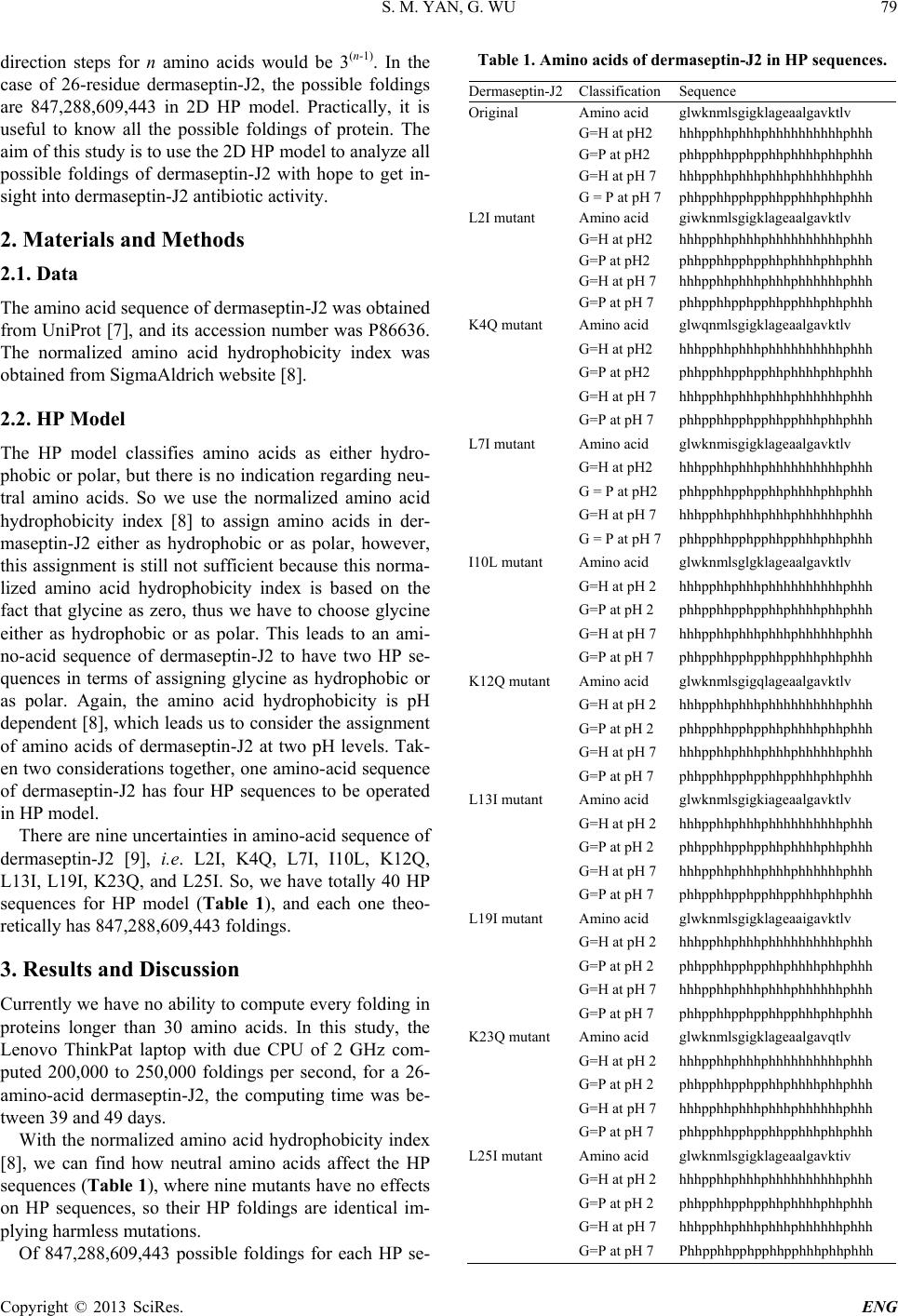
S. M. YAN, G. WU
Copyright © 2013 SciRes. ENG
direction steps for n amino acids would be 3(n-1). In the
case of 26-residue dermaseptin-J2, the possible foldings
are 847,288,609,443 in 2D HP model. Practically, it is
useful to know all the possible foldings of protein. The
aim of this study is to use the 2D HP model to analyze all
possible foldings of dermaseptin-J2 with hope to get in-
sight int o de rmasept in-J2 antibiotic activity.
2. Materials and Methods
2.1. Data
The amino acid sequence of dermaseptin-J2 was obtained
from UniProt [7], and its accession number w as P86636.
The normalized amino acid hydrophobicity index was
obtaine d from SigmaAldri c h we bs i te [8].
2.2. HP Model
The HP model classifies amino acids as either hydro-
phobic or polar, but there is n o indication regarding neu-
tral amino acids. So we use the normalized amino acid
hydrophobicity index [8] to assign amino acids in der-
maseptin-J2 either as hydrophobic or as polar, however,
this assignment is still not sufficient because this norma-
lized amino acid hydrophobicity index is based on the
fact that glycine as zero, thus we have to choose glycine
either as hydrophobic or as polar. This leads to an ami-
no-acid sequence of dermaseptin-J2 to have two HP se-
quences in terms of assigning glycine as hydrophobic or
as polar. Again, the amino acid hydrophobicity is pH
dependent [8], which leads us to consider the assignment
of amino acids of dermaseptin-J2 at two pH levels. Tak-
en two considerations together, one amino-acid s equence
of dermaseptin-J2 has four HP sequences to be operated
in HP model.
There are nine uncertainties in amino-acid sequence of
dermaseptin-J2 [9], i.e. L2I, K4Q, L7I, I10L, K12Q,
L13I, L19I, K23Q, and L25I. So, we have totally 40 HP
sequences for HP model (Table 1), and each one theo-
retically has 847,288,609,443 foldings.
3. Results and Discussion
Currently we have no ability to compute every folding in
proteins longer than 30 amino acids. In this study, the
Lenovo ThinkPat laptop with due CPU of 2 GHz com-
puted 200,000 to 250,000 foldings per second, for a 26-
amino-acid dermaseptin-J2, the computing time was be-
tween 39 a nd 4 9 da y s .
With the normalized amino acid hydrophobicity index
[8], we can find how neutral amino acids affect the HP
sequences (Tab le 1), wh ere nine mutants have no effects
on HP sequences, so their HP foldings are identical im-
plying harmless mutations.
Of 847,288,609,443 possible foldings for each HP se-
Table 1. Amino acids of dermaseptin-J2 in HP sequence s.
Dermaseptin-J2 Classification Sequence
Original Amino acid glwknmlsgigklageaalgavktlv
G=H at pH2 hhhpphhphhhphhhhhhhhhhphhh
G=P at pH2 phhpphhpphpphhphhhhphhphhh
G=H at pH 7 hhhpphhphhhphhhphhhhhhphhh
G = P at pH 7 phhpphhpphpphhpphhhphhphhh
L2I mutant Amino acid giwknmlsgigklageaalgavktlv
G=H at pH2 hhhpphhphhhphhhhhhhhhhphhh
G=P at pH2 phhpphhpphpphhphhhhphhphhh
G=H at pH 7 hhhpphhphhhphhhphhhhhhphhh
G=P at pH 7 phhpphhpphpphhpphhhphhphhh
K4Q mutant Amino acid glwqnmlsgigklageaalgavktlv
G=H at pH2 hhhpphhphhhphhhhhhhhhhphhh
G=P at pH2 phhpphhpphpphhphhhhphhphhh
G=H at pH 7 hhhpphhphhhphhhphhhhhhphhh
G=P at pH 7 phhpphhpphpphhpphhhphhphhh
L7I mutant Amino acid glwknmisgigklageaalgavktlv
G=H at pH2 hhhpphhphhhphhhhhhhhhhphhh
G = P at pH2 phhpphhpphpphhphhhhphhphhh
G=H at pH 7 hhhpphhphhhphhhphhhhhhphhh
G = P at pH 7 phhpphhpphpphhpphhhphhphhh
I10L mutant Amino acid glwknmlsglgklageaalgavktlv
G=H at pH 2 hhhpphhphhhphhhhhhhhhhphhh
G=P at pH 2 phhpphhpphpphhphhhhphhphhh
G=H at pH 7 hhhpphhphhhphhhphhhhhhphhh
G=P at pH 7 phhpphhpphpphhpphhhphhphhh
K12Q mutant Amino acid glwknmlsgigqlageaalgavktlv
G=H at pH 2 hhhpphhphhhphhhhhhhhhhphhh
G=P at pH 2 phhpphhpphpphhphhhhphhphhh
G=H at pH 7 hhhpphhphhhphhhphhhhhhphhh
G=P at pH 7 phhpphhpphpphhpphhhphhphhh
L13I mutant Amino acid glwknmlsgigkiageaalgavktlv
G=H at pH 2 hhhpphhphhhphhhhhhhhhhphhh
G=P at pH 2 phhpphhpphpphhphhhhphhphhh
G=H at pH 7 hhhpphhphhhphhhphhhhhhphhh
G=P at pH 7 phhpphhpphpphhpphhhphhphhh
L19I mutant Amino acid glwknmlsgigklageaaigavktlv
G=H at pH 2 hhhpphhphhhphhhhhhhhhhphhh
G=P at pH 2 phhpphhpphpphhphhhhphhphhh
G=H at pH 7 hhhpphhphhhphhhphhhhhhphhh
G=P at pH 7 phhpphhpphpphhpphhhphhphhh
K23Q mutant Amino acid glwknmlsgigklageaalgavqtlv
G=H at pH 2 hhhpphhphhhphhhhhhhhhhphhh
G=P at pH 2 phhpphhpphpphhphhhhphhphhh
G=H at pH 7 hhhpphhphhhphhhphhhhhhphhh
G=P at pH 7 phhpphhpphpphhpphhhphhphhh
L25I mutant Amino acid glwknmlsgigklageaalgavktiv
G=H at pH 2 hhhpphhphhhphhhhhhhhhhphhh
G=P at pH 2 phhpphhpphpphhphhhhphhphhh
G=H at pH 7 hhhpphhphhhphhhphhhhhhphhh
G=P at pH 7 Phhpphhpphpphhpphhhphhphhh