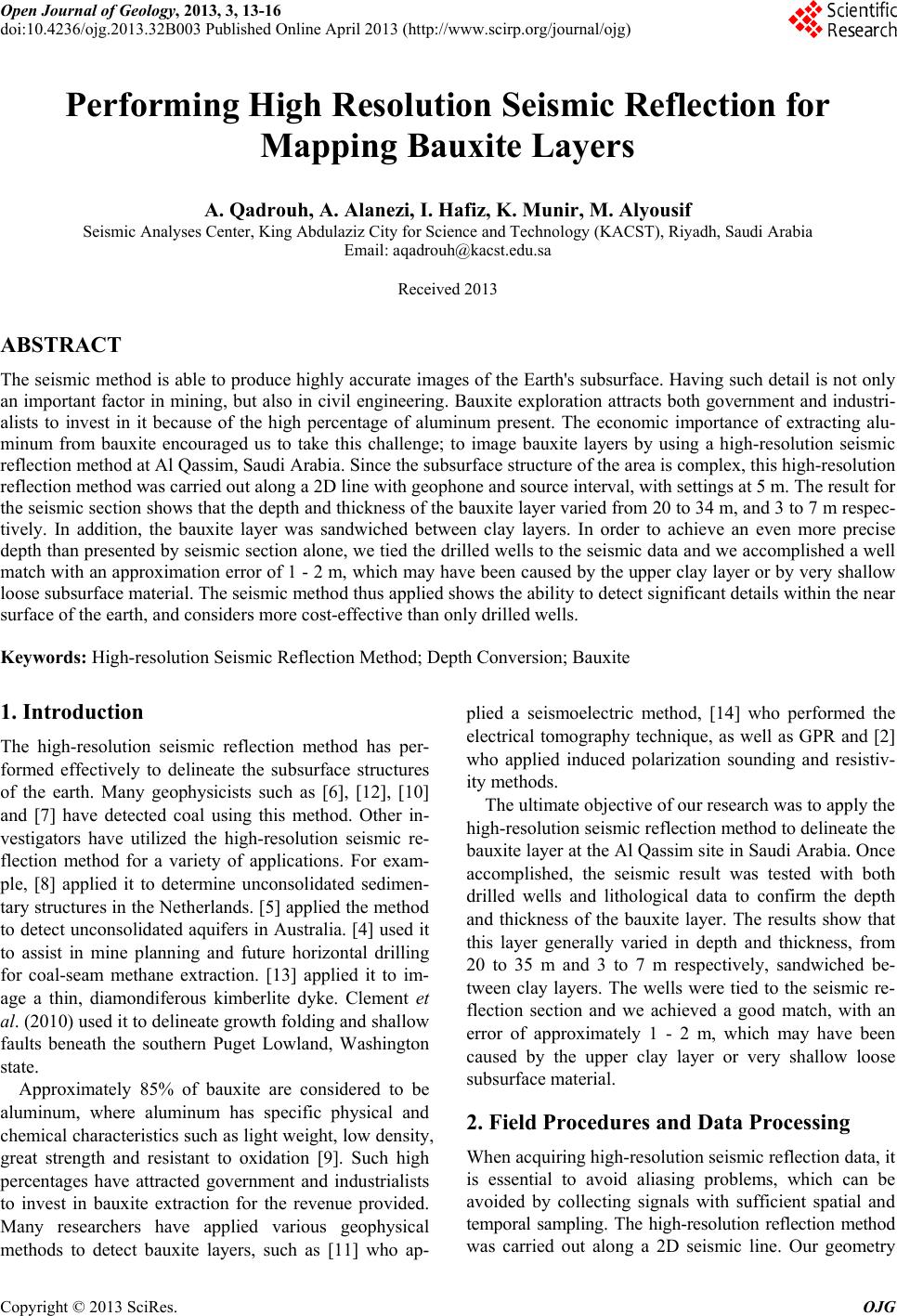
Open Journal of Geology, 2013, 3, 13-16
doi:10.4236/ojg.2013.32B003 Published Online April 2013 (http://www.scirp.org/journal/ojg)
Performing High Resolution Seismic Reflection for
Mapping Bauxite Layers
A. Qadrouh, A. Alanezi, I. Hafiz, K. Munir, M. Alyousif
Seismic Analyses Center, King Abdulaziz City for Science and Technology (KACST), Riyadh, Saudi Arabia
Email: aqadrouh@kacst.edu.sa
Received 2013
ABSTRACT
The seismic method is able to produce highly accurate images of the Earth's subsurface. Having such detail is not only
an important factor in mining, but also in civil engineering. Bauxite exploration attracts both government and industri-
alists to invest in it because of the high percentage of aluminum present. The economic importance of extracting alu-
minum from bauxite encouraged us to take this challenge; to image bauxite layers by using a high-resolution seismic
reflection method at Al Qassim, Saudi Arabia. Since the subsurface structure of the area is complex, this high-resolution
reflection method was carried out along a 2D line with geophone and source interv al, with se ttings at 5 m. The result for
the seismic section shows that the depth and thickness of the bauxite layer varied from 20 to 34 m, and 3 to 7 m respec-
tively. In addition, the bauxite layer was sandwiched between clay layers. In order to achieve an even more precise
depth than presen ted by seismic section alone, we tied the dr illed wells to the seismic data and we accomplished a well
match with an approximation error of 1 - 2 m, which may have been caused by the upper clay layer or by very shallow
loose subsurface material. The seismic method thus applied shows the ab ility to detect sign ificant details within th e n ear
surface of the earth, and considers more cost-effective than only drilled wells.
Keywords: High-resolution Seismic Reflection Method; Depth Conversion; Bauxite
1. Introduction
The high-resolution seismic reflection method has per-
formed effectively to delineate the subsurface structures
of the earth. Many geophysicists such as [6], [12], [10]
and [7] have detected coal using this method. Other in-
vestigators have utilized the high-resolution seismic re-
flection method for a variety of applications. For exam-
ple, [8] applied it to determine unconsolidated sedimen-
tary structures in the Netherlands. [5] applied the method
to detect unconsolidated aquifers in Australia. [4] used it
to assist in mine planning and future horizontal drilling
for coal-seam methane extraction. [13] applied it to im-
age a thin, diamondiferous kimberlite dyke. Clement et
al. (2010) used it to d elin eate g row th fold ing and sh allow
faults beneath the southern Puget Lowland, Washington
state.
Approximately 85% of bauxite are considered to be
aluminum, where aluminum has specific physical and
chemical characteristics such as light weight, low density,
great strength and resistant to oxidation [9]. Such high
percentages have attracted government and industrialists
to invest in bauxite extraction for the revenue provided.
Many researchers have applied various geophysical
methods to detect bauxite layers, such as [11] who ap-
plied a seismoelectric method, [14] who performed the
electrical tomography technique, as well as GPR and [2]
who applied induced polarization sounding and resistiv-
ity methods.
The ultimate objective of our research was to apply the
high-resolution seismic reflection method to delineate the
bauxite layer at the Al Qassim site in Saudi Arabia. Once
accomplished, the seismic result was tested with both
drilled wells and lithological data to confirm the depth
and thickness of the bauxite layer. The results show that
this layer generally varied in depth and thickness, from
20 to 35 m and 3 to 7 m respectively, sandwiched be-
tween clay layers. The wells were tied to the seismic re-
flection section and we achieved a good match, with an
error of approximately 1 - 2 m, which may have been
caused by the upper clay layer or very shallow loose
subsurface material.
2. Field Procedures and Data Processing
When acquiring high-resolution seismic reflection data, it
is essential to avoid aliasing problems, which can be
avoided by collecting signals with sufficient spatial and
temporal sampling. The high-resolution reflection method
was carried out along a 2D seismic line. Our geometry
Copyright © 2013 SciRes. OJG