 Journal of Surface Engineered Materials and Advanced Technology, 2013, 3, 287-294 http://dx.doi.org/10.4236/jsemat.2013.34039 Published Online October 2013 (http://www.scirp.org/journal/jsemat) Effect of Monovacancy Defects on Adsorbing of CO, CO2, NO and NO2 on Carbon Nanotubes: First Principle Calculations Ahlam A. EL-Barbary1,2*, Gehan H. Ismail1,2, Afaf M. Babeer2 1Physics Department, Ain Shams University, Cairo, Egypt; 2Physics Department, Faculty of Science, Jazan University, Jazan, KSA. Email: *ahla_eg@yahoo.co.uk Received August 28th, 2013; revised September 25th, 2013; accepted October 20th, 2013 Copyright © 2013 Ahlam A. EL-Barbary et al. This is an open access article distributed under the Creative Commons Attribution License, which permits unrestricted use, distribution, and reproduction in any medium, provided the original work is properly cited. ABSTRACT We have applied density functional theory to investigate different types of carbon nanotubes (armchair (4,4)CNT and zig-zag (7,0)CNT) as sensors of some pollutant gas molecules, especially CO, CO2, NO and NO2. We show, for the first time, that the adsorption of pollutant gas molecules on carbon nanotubes are improved by introducing the monovacancy defects on the surfaces of (7,0)CNT. The adsorption energies, the optimal adsorption positions and the orientation of these gas molecules on the surfaces of carbon nanotubes are studied. It is found that the most adsorbed pollutant gas is NO molecule on (7,0)CNT. Keywords: Carbon Nanotubes; DFT; Sensor; Monovacancy Defects 1. Introduction More than a decade after the discovery of carbon nano- tubes (CNT) by Iijima in 1991 [1], they are still one of the hottest research areas in all of science and engineer- ing. The specific geometry and unique properties of car- bon nanotubes suggest important potential applications including transistors, hydrogen storage devices, quantum dots, gas sensors, and so many other applications [2-5]. Gas adsorption on carbon nanotubes is a great issue for both essential research and applied application of nano- tubes. The adsorptive characteristics of SWCNTs in the gas phase caused their use as gas sensors of pollutant gas- es, and storage of fuels [6,7]. The most widely used consideration of atomistic mod- eling of a CNT is by reference to rolling up graphene sheet to form a hollow cylinder with end caps. The cyl- inder is composed of hexagonal carbon rings, while the end caps of pentagonal rings. In terms of the roll-up vec- tor, the armchair nanotubes are defined by (n, n) and the zigzag nanotubes by (n, 0). Due to the highly regular ato- mic structure of CNTs and the large degree of the struc- ture, purity makes it accessible to accurate computer mo- deling using a variety of theoretical techniques. The in- teraction between sp2-bonded carbon materials and mo- lecular species has been extensively investigated for car- bon nanotubes and fullerenes for sensor applications and chemical functionalization. Furthermore, there are evidences indicating the great importance of molecular gaseous orientation on the en- ergy of adsorption [8,9]. However, the effect of monova- cancy defects is one of the major aspects of gas-nanotube interactions that has not been studied yet. Therefore, in this study we will investigate the effect of chiralities of CNTs on adsorption energy, as well as the effect of mo- novacancy defects. 2. Computational Methods All calculations were performed with the DFT as imple- mented within G03W package [10-12], using B3LYP ex- change-functional and applying basis set 6 - 31 g(d,p). In the basis set 6 - 31 g(d,p), number 6 represents the num- ber of primitive Gaussians comprising each core atomic orbital basis function. Numbers 3 and 1 indicate that the valence orbitals are composed of two basis functions each, the first one composed of a linear combination of 3 primitive Gaussian functions, the other composed of a li- near combination of 1 primitive Gaussian functions, how- ever (d, p) are polarization (correlating) functions. This corresponds to the approximation method that makes use *Corresponding author. Copyright © 2013 SciRes. JSEMAT
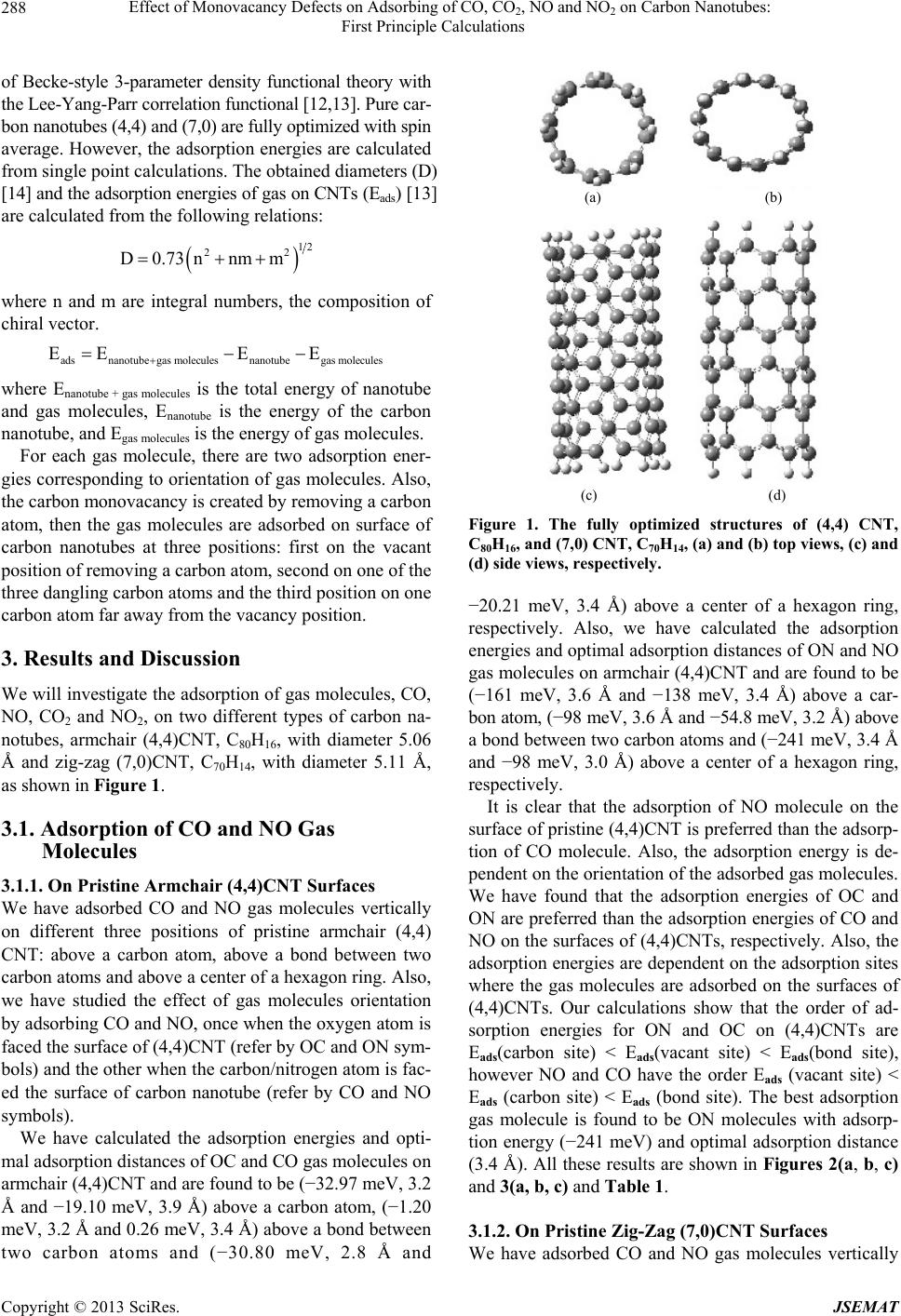 Effect of Monovacancy Defects on Adsorbing of CO, CO2, NO and NO2 on Carbon Nanotubes: First Principle Calculations 288 of Becke-style 3-parameter density functional theory with the Lee-Yang-Parr correlation functional [12,13]. Pure car- bon nanotubes (4,4) and (7,0) are fully optimized with spin average. However, the adsorption energies are calculated from single point calculations. The obtained diameters (D) [14] and the adsorption energies of gas on CNTs (Eads) [13] are calculated from the following relations: 12 22 D0.73n nmm where n and m are integral numbers, the composition of chiral vector. adsnanotubegas moleculesnanotubegas molecules EEE E where Enanotube + gas molecules is the total energy of nanotube and gas molecules, Enanotube is the energy of the carbon nanotube, and Egas molecules is the energy of gas molecules. For each gas molecule, there are two adsorption ener- gies corresponding to orientation of gas molecules. Also, the carbon monovacancy is created by removing a carbon atom, then the gas molecules are adsorbed on surface of carbon nanotubes at three positions: first on the vacant position of removing a carbon atom, second on one of the three dangling carbon atoms and the third position on one carbon atom far away from the vacancy position. 3. Results and Discussion We will investigate the adsorption of gas molecules, CO, NO, CO2 and NO2, on two different types of carbon na- notubes, armchair (4,4)CNT, C80H16, with diameter 5.06 Å and zig-zag (7,0)CNT, C70H14, with diameter 5.11 Å, as shown in Figure 1. 3.1. Adsorption of CO and NO Gas Molecules 3.1.1. On Pristine Armchair (4,4)CNT Surfaces We have adsorbed CO and NO gas molecules vertically on different three positions of pristine armchair (4,4) CNT: above a carbon atom, above a bond between two carbon atoms and above a center of a hexagon ring. Also, we have studied the effect of gas molecules orientation by adsorbing CO and NO, once when the oxygen atom is faced the surface of (4,4)CNT (refer by OC and ON sym- bols) and the other when the carbon/nitrogen atom is fac- ed the surface of carbon nanotube (refer by CO and NO symbols). We have calculated the adsorption energies and opti- mal adsorption distances of OC and CO gas molecules on armchair (4,4)CNT and are found to be (−32.97 meV, 3.2 Å and −19.10 meV, 3.9 Å) above a carbon atom, (−1.20 meV, 3.2 Å and 0.26 meV, 3.4 Å) above a bond between two carbon atoms and (−30.80 meV, 2.8 Å and (a) (b) (c) (d) Figure 1. The fully optimized structures of (4,4) CNT, C80H16, and (7,0) CNT, C70H14, (a) and (b) top views, (c) and (d) side views, respectively. −20.21 meV, 3.4 Å) above a center of a hexagon ring, respectively. Also, we have calculated the adsorption energies and optimal adsorption distances of ON and NO gas molecules on armchair (4,4)CNT and are found to be (−161 meV, 3.6 Å and −138 meV, 3.4 Å) above a car- bon atom, (−98 meV, 3.6 Å and −54.8 meV, 3.2 Å) above a bond between two carbon atoms and (−241 meV, 3.4 Å and −98 meV, 3.0 Å) above a center of a hexagon ring, respectively. It is clear that the adsorption of NO molecule on the surface of pristine (4,4)CNT is preferred than the adsorp- tion of CO molecule. Also, the adsorption energy is de- pendent on the orientation of the adsorbed gas molecules. We have found that the adsorption energies of OC and ON are preferred than the adsorption energies of CO and NO on the surfaces of (4,4)CNTs, respectively. Also, the adsorption energies are dependent on the adsorption sites where the gas molecules are adsorbed on the surfaces of (4,4)CNTs. Our calculations show that the order of ad- sorption energies for ON and OC on (4,4)CNTs are Eads(carbon site) < Eads(vacant site) < Eads(bond site), however NO and CO have the order Eads (vacant site) < Eads (carbon site) < Eads (bond site). The best adsorption gas molecule is found to be ON molecules with adsorp- tion energy (−241 meV) and optimal adsorption distance (3.4 Å). All these results are shown in Figures 2(a, b, c) and 3(a, b, c) and Table 1. 3.1.2. On Pristine Zig-Zag (7,0)CNT Surfaces We have adsorbed CO and NO gas molecules vertically Copyright © 2013 SciRes. JSEMAT
 Effect of Monovacancy Defects on Adsorbing of CO, CO2, NO and NO2 on Carbon Nanotubes: First Principle Calculations Copyright © 2013 SciRes. JSEMAT 289 (a) (b) (d) (e)(f) (c) 12 10 8 6 4 2 0 -2 -2 3 8 13 12 10 8 6 4 2 0 -2 14 -2 3 8 13 18 15 10 5 0 -2 3 8 13 18 0 5 5 10 1 0 2 3 4 0 2 4 6 0 2 4 6 8 0 2 4 6 8 0 2 4 6 8 adsorption distance/A adsorption distance/A adsorption distance/A adsorption energy/eV adsorption energy/eV Figure 2. Adsorption energies of CO and OC gas molecules on pristine (4,4)CNT surfaces (a) above a carbon atom, (b) above a bond between two carbon atoms, (c) above a center of hexagon ring. Adsorption energies of CO and OC gas molecules on pristine (7,0)CNT (d)above a carbon atom, (e) above a bond between two carbon atoms (f) above a center of hexagon ring. The blue marks are corresponding to the CO molecules and the red marks referred to OC molecule. (a) 12 10 8 6 4 2 0 -2 0 2 4 6 adsorption energy/eV (b) (c) (d) (e) (f) 12 10 8 6 4 2 0 -2 12 10 8 6 4 2 0 -2 8 0 1 2 3 4 0 1 2 3 4 -2 3 8 13 18 0 2 4 6 8 adsorption energy/eV 12 10 8 6 4 2 0 -2 0 2 46 8 adsorption distance/A 10 8 6 4 2 0 -2 0 1 2 3 4 adsorption distance/A adsorption distance/A Figure 3. Adsorption energies of NO and ON gas molecules on pristine (4,4)CNT surfaces (a) above a carbon atom, (b) above a bond between two carbon atoms, (c) above a center of hexagon ring. Adsorption energies of NO and ON gas molecules on pristine (7,0)CNT (d)above a carbon atom, (e) above a bond between two carbon atoms (f) above a center of hexagon ring. The blue marks are corresponding to the NO molecule and the red marks are referred to ON molecule.
 Effect of Monovacancy Defects on Adsorbing of CO, CO2, NO and NO2 on Carbon Nanotubes: First Principle Calculations 290 Table 1. The calculated adsorption energies (Eads) and optimal adsorption distances (R) of CO, and NO above a carbon site, bond site and vacant site of pristine (4,4)CNTs and (7,0)CNTs. All energies are given by meV and distance by Å. Carbon site Bond site Vacant site OC CO OC CO OC CO Eads/meV −32.97 −19.1 −1.2 0.26 −30.8 −20.21 R/Å 3.2 3.9 3.2 3.4 2.8 3.4 ON NO ON NO ON NO Eads/meV −161 −138 −98 −54.8 −241 −98 (4,4)CNT R/Å 3.6 3.4 3.6 3.2 3.4 3.0 OC CO OC CO OC CO Eads/meV −95 1.4 28.1 68.8 −73.9 39.1 R/Å 3.4 3.8 3.2 3.8 2.6 3.4 ON NO ON NO ON NO Eads/meV −1863.9 −1765.3 −1344.2 −1284.3 −1372.1 −1310.4 (7,0)CNT R/Å 2.8 2.6 3.3 3.3 3.0 3.6 on the same three positions of pristine zig-zag (7,0) CNT: above a carbon atom, above a bond between two carbon atoms and above a center of a hexagon ring. Also, we have studied the effect of gas molecules orientation by adsorbing CO and NO, once when the oxygen atom is faced the surface of (7,0)CNT (OC and ON) and the other when the carbon/nitrogen atom is faced the surface (CO and NO). We have calculated the adsorption energies and opti- mal adsorption distances of OC and CO gas molecules on armchair (7,0)CNT and are found to be (−95 meV, 3.4Å and 1.4 meV, 3.8 Å) above a carbon atom, (28.1 meV, 3.2 Å and 68.8 meV, 3.8 Å) above a bond between two carbon atoms and (−73.9 meV, 2.6 Å and 39.1 meV,3.4 Å) above a center of a hexagon ring, respectively. Also, we have calculated the adsorption energies and optimal adsorption distances of ON and NO gas molecules on zig-zag (7,0)CNT and are found to be (−1863.9 meV, 2.8 Å and −1765.3 meV, 2.6 Å) above a carbon atom, (−1344.2 meV, 3.3 Å and −1284.3 meV, 3.3 Å) above a bond between two carbon atoms and (−1372.1 meV, 3.6 Å and −1310.4 meV, 3.0 Å) above a center of a hexagon ring, respectively. It is clear that the adsorption of NO on the surface of (7,0)CNT is preferred than the adsorption of CO. Also, the adsorption energy is dependent on the orientation of the adsorbed gas molecules. We have found that the ad- sorption energies of OC and ON are preferred than the adsorption energies of CO and NO on the surfaces of (7,0)CNTs, respectively. Also, the adsorption energies are dependent on the adsorption sites where the gas mo- lecules are adsorbed on the surfaces of (7,0)CNTs. Our calculations show that the order of adsorption energies for adsorbing CO, OC, NO and ON are Eads(carbon site) < Eads(vacant site) < Eads(bond site), see Figures 2 and 3(d, e, f) and Table 1. 3.2. Adsorption of CO2 and NO2 on (4,4)CNT and (7,0)CNT Surfaces We have calculated the adsorption energies and the op- timal distances of CO2 and NO2 above a carbon atom site, a bond site, and a vacant site of pristine (4,4)CNT. They are found to be (−33 meV, 3.7 Å), (−80 meV, 3.4 Å) and (−38 meV, 2.2 Å) for CO2, respectively, and (−37 meV, 3.1 Å), (−146.6 meV, 2.4 Å) and (−81 meV, 2.8 Å) for NO2, respectively. The calculated adsorption energies and the optimal distances of CO2 and NO2 above a car- bon atom site, a bond site, and a vacant site of pristine (7,0)CNT are found to be (−169.3 meV, 3.4 Å), (−23.9 meV, 3.8 Å), (−77.7 meV, 2.8 Å) for CO2, respectively, and (−1602.7 meV, 2.6 Å), (−972.8 meV, 2.2 Å) and (−1218.9 meV, 2.8 Å) for NO2, respectively. One can see that the adsorption of NO2 on the surface of (4,4)CNT is preferred than the adsorption of CO2. Also, the adsorption energy is dependent on the type of CNTs. We have found that the adsorption energies of NO2 on (7,0)CNT are preferred than the adsorption ener- gies NO2 on (4,4)CNT. Also, the adsorption energies are dependent on the adsorption sites where the gas mole- cules are adsorbed on the surfaces of (4,4)CNTs and (7,0)CNTs. Our calculations show that the order of ad- sorption energies for adsorbing CO2 and NO2 on (4,4) Copyright © 2013 SciRes. JSEMAT
 Effect of Monovacancy Defects on Adsorbing of CO, CO2, NO and NO2 on Carbon Nanotubes: First Principle Calculations 291 CNTs are Eads(bond site) < Eads(vacant site) < Eads(carbon site), however for adsorbing CO2 and NO2 on (7,0)CNTs have the order Eads(carbon site) < Eads(vacant site) < Eads(bond site). All these results are shown in Figure 4 and Table 2. 3.3. Adsorption Energies of CO, NO and CO2 on Monovacancy Defected (4,4)CNTs We have studied the adsorption energies and optimal ad- sorption energies of CO, NO and CO2 of the monova- cancy defected (4,4) CNT on three different sites. The calculated adsorption energies and optimal adsorption energies for CO molecules are found to be (39 meV, 3.0 Å), (−88 meV, 3.9 Å) and (40 meV, 3.2 Å) on a vacant site, on one dangling carbon atom site, and on a carbon atom far away from the position of monovacancy defects, respectively. The optimal adsorption energies for NO molecules are (−770 meV, 3.4 Å), (−560 meV, 3.0 Å) and (−280 meV, 3.9 Å) on a vacant site, on one dangling carbon atom site, and on a carbon atom far away from the position of monovacancy defects, respectively. The calculated adsorption energies and optimal ad- sorption distances for CO2 molecules are (−1 meV, 2.7 Å), (−10 meV, 3.0 Å) and (47 meV, 3.2 Å) on a vacant site, on one dangling carbon atom site, and on a carbon atom far away from the position of monovacancy defects, respectively, as shown in Figure 5 and Table 3. One can see that the adsorption of CO on the monovacancy de- fected surface of (4,4)CNT is enhancement only on the position of dangling carbon atom ( the adsorption energy is changed from −32.97 meV to −88 meV). For NO ad- sorption, the enhancement is achieved on the three posi- tions from −138 meV to −770 meV above the vacant site, to −560 meV above the dangling carbon atom site and to −280 meV above the carbon atom site. However, for the CO2 there is no enhancement on any adsorption site. 3.4. Adsorption Energies of CO, NO and CO2 on Monovacancy Defected (7,0)CNTs We have calculated the adsorption energies and the op- timal adsorption distances of CO, NO and CO2 of the monovacancy defected (7,0) CNT on the same three sites, above a vacant site due to a removing carbon atom site, above one dangling carbon atom site, and a carbon atom (a) -1 0 1 0 2 4 6 adsorption energy/eV adsorption distance/A (b) (c)(d) adsorption distance/A adsorption distance/A adsorption distance/A 2 3 4 8 5 6 7 0 2 4 6 8 10 12 14 16 18 20 -2 0 2 4 6 8 10 12 14 -2 0 2 4 6 8 10 12 14 16 18 01 2340 1 2 0 2 46 -2 3 4 Figure 4. The calculated adsorption energies above carbon atom site (blue marks), above bond site (red marks) and above vacant site (green marks) of (a) CO2 on (4,4)CNT, (b) NO2 on (4,4)CNT (c) CO2 on (4,4)CNT and (d)NO2 on (7,0)CNT. Table 2. The calculated adsorption energies and optimal adsorption distances of CO2 and NO2, above a carbon site, bond site and vacant site of pristine (4,4)CNTs and (7,0)CNTs. All energies are given by meV and distance by Å. Carbon site Bond site Vacant site CO2 NO2 CO2 NO2 CO2 NO2 Eads/meV −33 −37 −80 −146.6 −38 −81 (4,4)CNT R/Å 3.7 3.11 3.4 2.4 2.2 2.8 CO2 NO2 CO2 NO2 CO2 NO2 Eads/meV −169.3 −1602.7 −23.9 −972.8 −77.7 −1218.9 (7,0)CNT R/Å 3.4 2.6 3.8 2.2 2.8 2.8 Copyright © 2013 SciRes. JSEMAT
 Effect of Monovacancy Defects on Adsorbing of CO, CO2, NO and NO2 on Carbon Nanotubes: First Principle Calculations 292 (a) 0 5 0 2 4 6 adsorption energy adsorption distance/A adsorption distance/A adsorption distance/A 02 46 10 0 2 4 6 0 1 23 4 (b) (c) (f) (e) (d) (g) (h) (i) 0 5 10 -5 0 2 4 6 0 5 10 -5 0 5 10 -5 15 3 8 -2 0 0.5 1 1.5 2 0 2 -2 4 6 0 4 1 2 3 0 2 4 6 0 1 2 3 4 5 012 345012 3 4 5 01 2 3 4 0 12 012 34 adsorption energy adsorption energy Figure 5. Adsorption energies of CO, NO and CO2 gas molecules on the monovacancy defects of (4,4)CNTs. (a, d, g), above the vacancy site for CO, NO and CO2, respectively. (b, e, h) above one of three dangling carbon atoms for CO, NO and CO2, respectively, and (c, f, i) above a carbon atom far away from the vacancy defect for CO, NO and CO2, respectively. Table 3. The calculated adsorption energies and optimal adsorption distances of CO, NO and NO2, on monovacancy defected (4,4)CNTs and (7,0)CNTs. All energies are given by meV and distance by Å. Vacant site Dangling carbon atom site Carbon atom site CO NO CO2 CO NO CO2 CO NO CO2 Eads/meV 39 −770 −1.0 −88 −560 10 40 −280 47 (4,4)CNT R/Å 3.0 3.4 2.7 3.9 3.0 3.0 3.2 3.9 3.2 Eads/meV 90 −2410 7 −220 −2410 −99 −240 −2420 −12 (7,0)CNT R/Å 2.6 2.6 2.8 3.8 3.8 3.8 2.6 3.8 3.0 site far away from the position of monovacancy defects. The calculated adsorption energies and optimal adsorp- tion energies for CO molecules are (90 meV, 2.6 Å), (−220 meV, 3.8 Å) and (−240 meV, 2.6 Å) on a vacant site, on one dangling carbon atom site, and on a carbon atom site, respectively. The calculated adsorption energies and optimal ad- sorption distances for NO molecules are (−2410 meV, 2.6 Å), (−2410 meV, 3.8 Å) and (−2420 meV, 3.8 Å), respectively. The calculated adsorption energies and the optimal adsorption distances for CO2 molecules are (7 meV, 2.8 Å), (−99 meV, 3.8 Å) and (−12 meV, 3.0 Å) on a vacant site, on one dangling carbon atom site, and on a carbon atom site, respectively, as shown in Figure 6 and Table 3. One can see that there is no enhancement of CO2 adsorption on any adsorption site. However, the adsorption of CO and NO is improved by applying the monovacancy defects on (7,0)CNTs. 3.5. Mulliken Atomic Charges To understand more about the surface reactivity, the Mulliken atomic charges are calculated for adsorbing some selected gas molecules on pristine (4,4)CNT and (7,0)CNT. As shown in Figure 7, the carbon atom or ni- trogen atom of CO and NO possess always positive Copyright © 2013 SciRes. JSEMAT
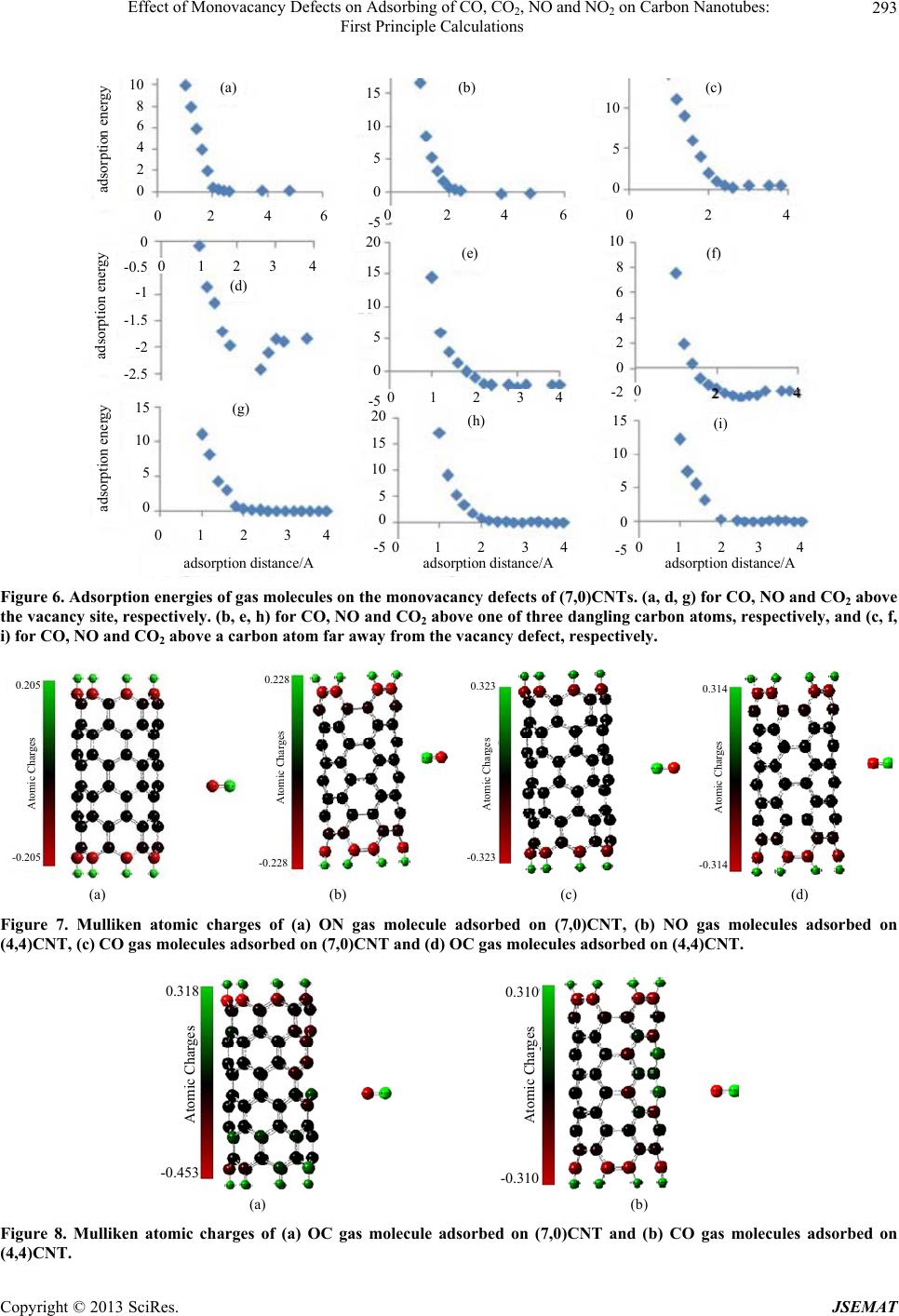 Effect of Monovacancy Defects on Adsorbing of CO, CO2, NO and NO2 on Carbon Nanotubes: First Principle Calculations 293 (a) 0 0 1 2 3 adsorption energy adsorption distance/A 2 4 10 6 4 0123 401 2 3 4 adsorption distance/A adsorption distance/A (b)(c) (f) (e) (d) (g) (h) (i) 8 0 2 4 10 6 8 -2 0 5 10 15 0 5 10 -5 0 -0.5 -1 -1.5 -2 -2.5 0 5 10 15 -5 20 0 5 10 15 0 5 10 15 -5 20 0 5 10 15 -5 0123 4 0 1 2 3 4 0 2 4 6 024602 4 02 4 adsorption energy adsorption energy Figure 6. Adsorption energies of gas molecules on the monovacancy defects of (7,0)CNTs. (a, d, g) for CO, NO and CO2 above the vacancy site, respectively. (b, e, h) for CO, NO and CO2 above one of three dangling carbon atoms, respectively, and (c, f, i) for CO, NO and CO2 above a carbon atom far away from the vacancy defect, respectively. Atomic Charges -0.205 0.205 Atomic Charges -0.228 0.228 Atomic Charges -0.323 0.323 Atomic Charges -0.314 0.314 (a) (b) (c) (d) Figure 7. Mulliken atomic charges of (a) ON gas molecule adsorbed on (7,0)CNT, (b) NO gas molecules adsorbed on (4,4)CNT, (c) CO gas molecules adsorbed on (7,0)CNT and (d) OC gas molecules adsorbed on (4,4)CNT. Atomic Charges -0.453 0.318 Atomic Charges -0.310 0.310 (a) (b) Figure 8. Mulliken atomic charges of (a) OC gas molecule adsorbed on (7,0)CNT and (b) CO gas molecules adsorbed on (4,4)CNT. Copyright © 2013 SciRes. JSEMAT
 Effect of Monovacancy Defects on Adsorbing of CO, CO2, NO and NO2 on Carbon Nanotubes: First Principle Calculations 294 charge, however, oxygen atom possesses always negative charge. This can be explained in terms the electron infin- ity of the oxygen is more than the electron infinities of carbon and nitrogen. Therefore, the adsorption energies of OC and ON are preferred than the adsorption energies of CO and NO on the surfaces of carbon nanotubes. Also, the Mulliken atomic charges are calculated for adsorbing some selected gas molecules on monovacancy defects of (4,4)CNT and (7,0)CNT. As shown in Figure 8, introducing the monovacancy defects leads to change the zero charge of carbon atom in case of pristine nano- tubes into partially negative and positive charges, espe- cially three dangling carbon atoms. Therefore, introduce- ing the monovacancy defects on carbon nanotubes sur- faces is caused the enhancement of adsorption energies. 4. Conclusion We have studied the adsorption energies of some pollut- ant gas molecules as CO, NO, CO2 and NO2 on (4,4) CNT and (7,0)CNT. We have investigated the effects of different adsorbed sites and the orientation of adsorbed gas molecules, in addition to introducing the monova- cancy defects on (4,4) CNT and (7,0) CNT surfaces. One can conclude that the zig-zag (7,0) CNT is better than the armchair (4,4)CNT for adsorbing pollutant gas mole- cules, especially, NO gas molecules. Also, we have found that introducing the monovacancy defects is improved the adsorption only on the (7,0)CNT of CO and NO gas molecules. REFERENCES [1] S. Iijima, “Helical Microtubules of Graphitic Carbon,” Nature, Vol. 354, No. 7, 1991, p. 56. http://dx.doi.org/10.1038/354056a0 [2] F. Schedin, A. K. Geim, S. V. Morozov, E. W. Hill, P. Blake, M. I. Katsnelson and K. S. Novoselov, “Detection of Individual Gas Molecules Adsorbed on Graphene,” Nature Mater, Vol. 6, No. 9, 2007, p. 652. http://dx.doi.org/10.1038/nmat1967 [3] S. Peng, K. Cho, P. Qi and H. Dai, “Ab Initio Study of CNT NO2 Gas Sensor,” Chemical Physics Letters, Vol. 387, No. 4-6, 2004, p. 271. http://dx.doi.org/10.1016/j.cplett.2004.02.026 [4] J. Kong, N. Franklin, C. Zhou, M. G. Chapline, S. Peng, K. Cho and H. Dai, “Nanotube Molecular Wires as Che- mical Sensors,” Science, Vol. 287, No. 5453, 2000, p. 622. http://dx.doi.org/10.1126/science.287.5453.622 [5] S. Peng and K. Cho, “Chemical Control of Nanotube Elec- tronics,” Nanotechnology, Vol. 11, No. 4, 2000, p. 57. http://dx.doi.org/10.1088/0957-4484/11/2/303 [6] J. E. Fischer, “Carbon Nanotubes: A Nanostructured Ma- terial for Energy Storage,” Chemical Innovation, Vol. 30, No. 9, 2000, p. 21. [7] V. Meunier, J. Kephart, C. Roland and J. Bernholc, “Ab Initio Investigations of Lithium Diffusion in Carbon Na- notube Systems,” Physical Review Letters, Vol. 88, No. 7, 2002, p. 075506. http://dx.doi.org/10.1103/PhysRevLett.88.075506 [8] M. Keshavarz and M. Beheshti, “Investigation of Chemi- cal Adsorption of CO, CO2, H2 and NO Molecules on In- side and Outside of Single-Wall Nanotube Using HF and DFT Calculations,” Journal of Physical and Theoretical Chemistry, IAU Iran, Vol. 7, No. 2, 2010, p. 111. [9] K. Azizi, S. M. Hashemianzadeh and Sh. Bahramifar, “The Effect of Source Temperature on Morphological and Op- tical Properties of ZnO Nanowires Grown Using a Modi- fied Thermal Evaporation Set-Up,” Current Applied Phy- sics, Vol. 11, No. 3, 2011, pp. 776-782. http://dx.doi.org/10.1016/j.cap.2010.11.071 [10] M. J. Frisch, G. W. Trucks, H. B. Schlegel, G. E. Scuseria, M. A. Robb, J. R. Cheeseman, V. G. Zakrzewski, J. A. Montgomery, R. E. Stratmann, J. C. Burant, S. Dapprich, J. M. Millam, A. D. Daniels, K. N. Kudin, M. C. Strain, O. Farkas, J. Tomasi, V. Barone, M. Cossi, R. Cammi, B. Mennucci, C. Pomelli, C. Adamo, S. Clifford, J. Ochter- ski, G. A. Petersson, P. Y. Ayala, Q. Cui, K. Morokuma, D. K. Malick, A. D. Rabuck, K. Raghavachari, J. B. Fo- resman, J. Cioslowski, J. V. Ortiz, B. B. Stefanov, G. Liu, A. Liashenko, P. Piskorz, I. Komaromi, R. Gomperts, R. L. Martin, D. J. Fox, T. Keith, M. A. Al-Lamham, C. Y. Peng, A. Nanayakkara, C. Gonzalez, M. Challacombe, P. M. W. Gill, B. G. Johnson, W. Chen, M. W. Wong, J. L. Andres, M. Head-Gordon, E. S. Replogle and J. A. Pople, Gaussian, Inc., Wallingford CT, 2004. [11] A. A. EL-Barbary, H. I. Lebda and M. A. Kamel, “The High Conductivity of Defect Fullerene C40 Cage,” Com- putational Materials Science, Vol. 46, 2009, p. 128. http://dx.doi.org/10.1016/j.commatsci.2009.02.034 [12] A. A. El-Barbary, Kh. M. Eid, M. A. Kamel and M. M. Has- san, “Band Gap Engineering in Short Heteronanotube Seg- ments via Monovacancy Defects,” Computational Mate- rials Science, Vol. 69, 2013, p. 87. http://dx.doi.org/10.1016/j.commatsci.2012.10.035 [13] H. Chang, J. D. Lee, S. M. Lee and Y. H. Lee, “Adsorp- tion of NH3 and NO2 Molecules on Carbon Nanotubes,” Applied Physics Letters, Vol. 79, No. 23, 2001, p. 3863. http://dx.doi.org/10.1063/1.1424069 [14] H. Nalwa, “Nanostructured Materials and Nanotechnolo- gy,” Academic Press, San Diego, 2002. Copyright © 2013 SciRes. JSEMAT
|