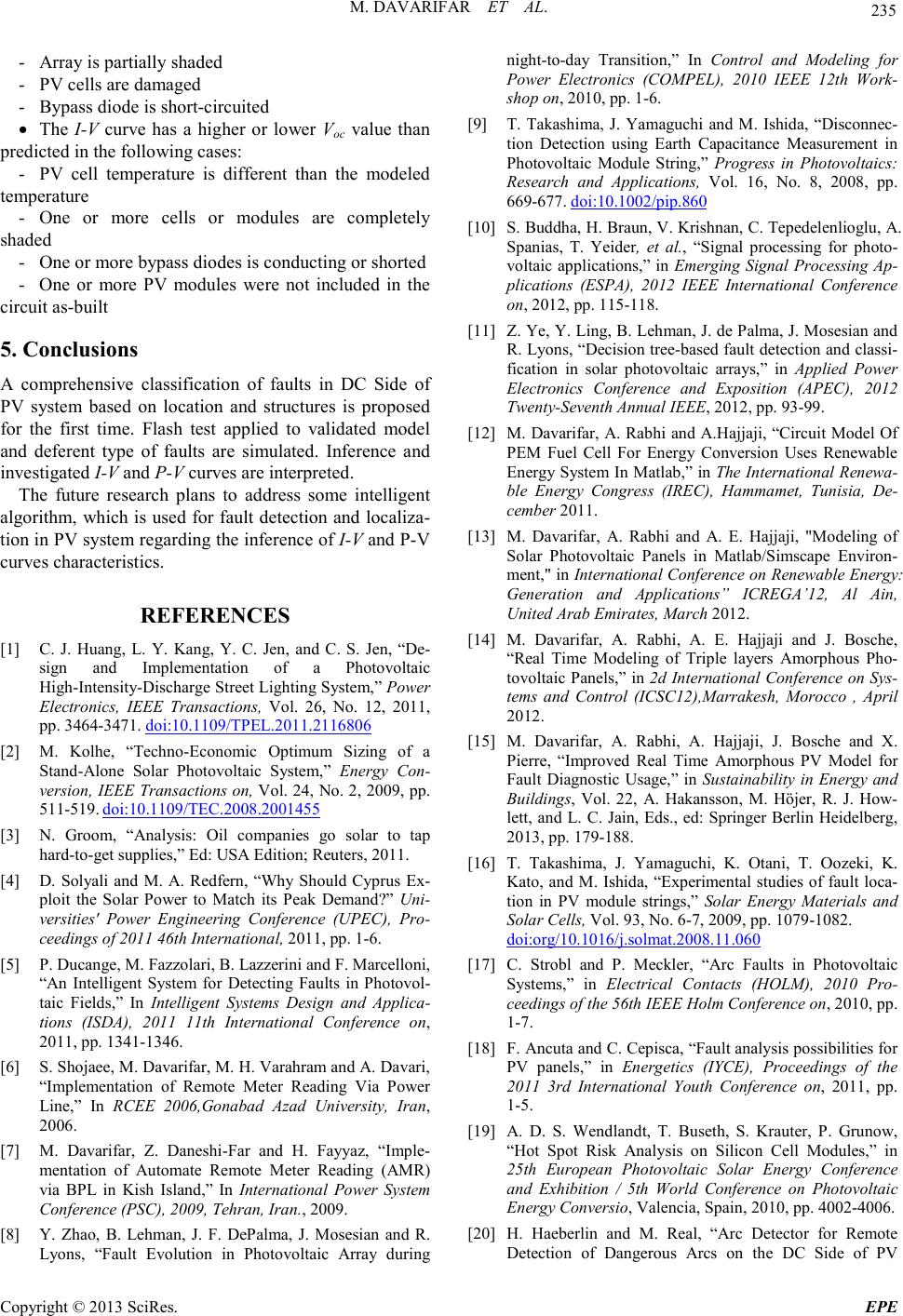
M. DAVARIF AR ET AL.
Copyright © 2013 SciRes. EPE
- Array is partially shaded
- PV cells are damaged
- Bypass diode is short-circuited
• The I-V curve has a higher or lower Voc value than
predicte d in the following cases:
- PV cell temperature is different than the modeled
temperature
- One or more cells or modules are completely
shaded
- One or more bypass diodes is conducting or s horte d
- One or more PV modules were not included in the
circuit as-built
5. Conclusions
A comprehensive classification of faults in DC Side of
PV system based on location and structures is proposed
for the first time. Flash test applied to validated model
and deferent type of faults are simulated. Inference and
investigated I-V and P-V curves are interpreted.
The future research plans to address some intelligent
algorithm, which is used for fault detection and localiza-
tion i n P V sys tem r egar din g the inference of I-V and P -V
curves characteristics.
REFERENCES
[1] C. J. Huang, L. Y. Kang, Y. C. Jen, and C. S. Jen, “De-
sign and Implementation of a Photovoltaic
High-Intensity-Discharge Street Lighting System,” Power
Electronics, IEEE Transactions, Vol. 26, No. 12, 2011,
pp. 3464 -3471. doi:10.1109/TPEL.2011.2116806
[2] M. Kolhe, “Techno-Economic Optimum Sizing of a
Stand-Alone Solar Photovoltaic System,” Energy Con-
version, IEEE Transactions on, Vol. 24, No. 2, 2009, pp.
511-519. doi:10.1109/TEC.2008.2001455
[3] N. Groom, “Analysis: Oil companies go solar to tap
hard-to -get supplies,” Ed: USA Edition; Reuters, 2011.
[4] D. Solyali and M. A. Redfern, “Why Should Cyprus Ex-
ploit the Solar Power to Match its Peak Demand?” Uni-
versities' Power Engineering Conference (UPEC), Pro-
ceedings of 2011 46th International, 2011, pp. 1-6.
[5] P. D ucange, M. Fazzolari , B. Lazzerini and F. Marcelloni,
“An Intelligent System for Detecting Faults in Photovol-
taic Fields,” In Intelligent Systems Design and Applica-
tions (ISDA), 2011 11th International Conference on,
2011, pp . 1341-1346.
[6] S. Sho jaee, M . Davari far, M. H. Varahram and A. Davari,
“Implementation of Remote Meter Reading Via Power
Line,” In RCEE 2006,Gonabad Azad University, Iran,
2006.
[7] M. Davarifar, Z. Daneshi-Far and H. Fayyaz, “I mpl e-
mentation of Automate Remote Meter Reading (AMR)
via BPL in Kish Island,” In International Power System
Conference (PSC), 2009, Tehran, Iran., 2009.
[8] Y. Zhao, B. Lehman, J. F. DePalma, J. Mosesian and R.
Lyons, “Fault Evolution in Photovoltaic Array during
night-to-day Transition,” In Control and Modeling for
Power Electronics (COMPEL), 2010 IEEE 12th Work-
shop on, 2010, pp. 1-6.
[9] T. Takashima, J. Yamaguchi and M. Ishida, “Disconnec-
tion Detection using Earth Capacitance Measurement in
Photovoltaic Module String,” Progress in Photovoltaics:
Research and Applications, Vol. 16, No. 8, 2008, pp.
669-677. doi:10.1002/pip.860
[10] S. Buddha, H. Braun, V. Krishnan, C. Tepedelenlioglu, A.
Spanias, T. Yeider, et al., “Signal processing for photo-
voltaic applications,” in Emerging Signal Processing Ap-
plications (ESPA), 2012 IEEE International Conference
on, 2012, pp. 115-118.
[11] Z. Ye, Y. Ling, B. Lehman, J. de Palma, J. Mosesian and
R. Lyons, “Decisi on tree-b ased fault d etection an d classi-
fication in solar photovoltaic arrays,” in Applied Power
Electronics Conference and Exposition (APEC), 2012
Twent y-Seventh An nu al IEE E , 2012, pp. 93-99.
[12] M. Davarifar, A. Rab hi and A.Hajjaji, “Circuit Model Of
PEM Fuel Cell For Energy Conversion Uses Renewable
Energy System In Matlab,” in The Internat ional Renewa-
ble Energy Congress (IREC), Hammamet, Tunisia, De-
cember 2011.
[13] M. Davarifar, A. Rabhi and A. E. Hajjaji, "Modeling of
Solar Photovoltaic Panels in Matlab/Simscape Environ-
ment," in International Conference on Renewable Energy:
Generation and Applications” ICREGA’12, Al Ain,
Unite d Ar ab E m ir at e s , March 2012.
[14] M. Davarifar, A. Rabhi, A. E. Hajjaji and J. Bosche,
“Real Time Modeling of Triple layers Amorphous Pho-
tovoltaic Panels,” in 2d International Conference on Sys-
tems and Control (ICSC12),Marrakesh, Morocco , April
2012.
[15] M. Davarifar, A. Rabhi, A. Hajjaji, J. Bosche and X.
Pierre, “Improved Real Time Amorphous PV Model for
Fault Diagnostic Usage,” in Sustainability in Energy and
Buildings, Vol. 22, A. Hakansson, M. Höjer, R. J. How-
lett, and L. C. Jain, Eds., ed: Springer Berlin Heidelberg,
2013, pp . 179-188.
[16] T. Takashima, J. Yamaguchi, K. Otani, T. Oozeki, K.
Kato, and M. Ishida, “Experimental studies of fault loca-
tion in PV module strings,” Solar Energy Materials and
Solar Cells, Vol. 93, No. 6-7, 2009, pp. 1079-1082.
doi:org/10.1016/j.solmat.2008.11.060
[17] C. Strobl and P. Meckler, “Arc Faults in Photovoltaic
Systems,” in Electrical Contacts (HOLM), 2010 Pro-
ceedings of the 56th IEEE Holm Conference on, 20 10, pp.
1-7.
[18] F. Ancu ta and C. Cep isca, “Fault analysis possibilities for
PV panels,” in Energetics (IYCE), Proceedings of the
2011 3rd International Youth Conference on, 2011, pp.
1-5.
[19] A. D. S. Wendlandt, T. Buseth, S. Krauter, P. Grunow,
“Hot Spot Risk Analysis on Silicon Cell Modules,” in
25th European Photovoltaic Solar Energy Conference
and Exhibition / 5th World Conference on Photovoltaic
Energ y Conversio, Valencia, Spain, 2010, pp. 4002-4006.
[20] H. Haeberlin and M. Real, “Arc Detector for Remote
Detection of Dangerous Arcs on the DC Side of PV