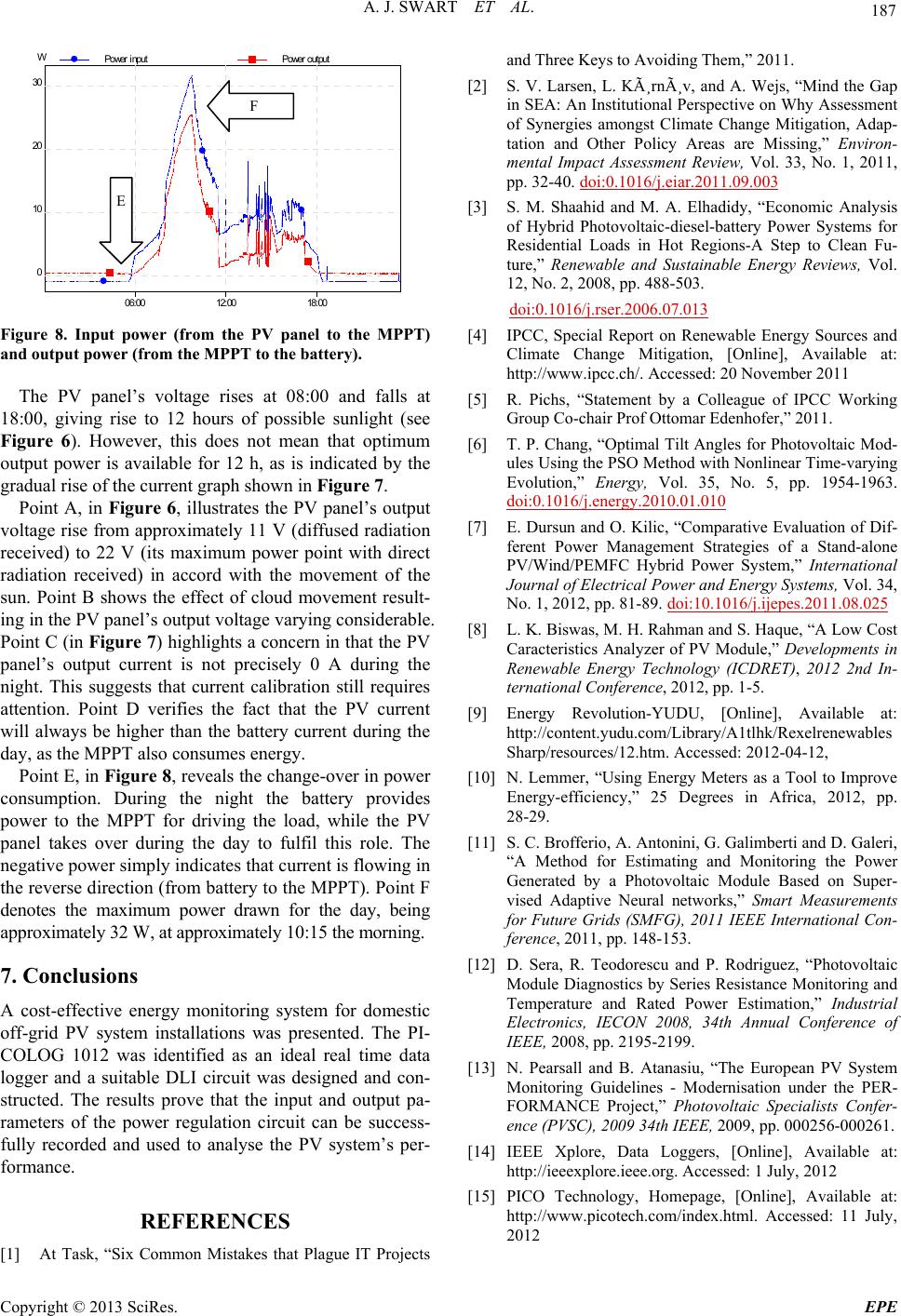
A. J. SWART ET AL. 187
W
0
10
20
30
Pow er inputPow er output
06:00 12:00 18:00
E
F
Figure 8. Input power (from the PV panel to the MPPT)
and output power (from the MPPT to the battery).
The PV panel’s voltage rises at 08:00 and falls at
18:00, giving rise to 12 hours of possible sunlight (see
Figure 6). However, this does not mean that optimum
output power is available for 12 h, as is indicated by the
gradual rise of the current graph shown in Figure 7.
Point A, in Figure 6, illustrates the PV panel’s output
voltage rise from approximately 11 V (diffused radiation
received) to 22 V (its maximum power point with direct
radiation received) in accord with the movement of the
sun. Point B shows the effect of cloud movement result-
ing in the PV panel’s output voltage varying considerable.
Point C (in Figure 7) highlights a concern in that the PV
panel’s output current is not precisely 0 A during the
night. This suggests that current calibration still requires
attention. Point D verifies the fact that the PV current
will always be higher than the battery current during the
day, as the MPPT also consumes energy.
Point E, in Figure 8, reveals the change-over in power
consumption. During the night the battery provides
power to the MPPT for driving the load, while the PV
panel takes over during the day to fulfil this role. The
negative power simply indicates that current is flowing in
the reverse direction (from battery to the MPPT). Point F
denotes the maximum power drawn for the day, being
approximately 32 W, at approximately 10:15 the morning.
7. Conclusions
A cost-effective energy monitoring system for domestic
off-grid PV system installations was presented. The PI-
COLOG 1012 was identified as an ideal real time data
logger and a suitable DLI circuit was designed and con-
structed. The results prove that the input and output pa-
rameters of the power regulation circuit can be success-
fully recorded and used to analyse the PV system’s per-
formance.
REFERENCES
[1] At Task, “Six Common Mistakes that Plague IT Projects
and Three Keys to Avoiding Them,” 2011.
[2] S. V. Larsen, L. Kørnøv, and A. Wejs, “Mind the Gap
in SEA: An Institutional Perspective on Why Assessment
of Synergies amongst Climate Change Mitigation, Adap-
tation and Other Policy Areas are Missing,” Environ-
mental Impact Assessment Review, Vol. 33, No. 1, 2011,
pp. 32-40. doi:0.1016/j.eiar.2011.09.003
[3] S. M. Shaahid and M. A. Elhadidy, “Economic Analysis
of Hybrid Photovoltaic-diesel-battery Power Systems for
Residential Loads in Hot Regions-A Step to Clean Fu-
ture,” Renewable and Sustainable Energy Reviews, Vol.
12, No. 2, 2008, pp. 488-503.
doi:0.1016/j.rser.2006.07.013
[4] IPCC, Special Report on Renewable Energy Sources and
Climate Change Mitigation, [Online], Available at:
http://www.ipcc.ch/. Accessed: 20 November 2011
[5] R. Pichs, “Statement by a Colleague of IPCC Working
Group Co-chair Prof Ottomar Edenhofer,” 2011.
[6] T. P. Chang, “Optimal Tilt Angles for Photovoltaic Mod-
ules Using the PSO Method with Nonlinear Time-varying
Evolution,” Energy, Vol. 35, No. 5, pp. 1954-1963.
doi:0.1016/j.energy.2010.01.010
[7] E. Dursun and O. Kilic, “Comparative Evaluation of Dif-
ferent Power Management Strategies of a Stand-alone
PV/Wind/PEMFC Hybrid Power System,” International
Journal of Electrical Power and Energy Systems, Vol. 34,
No. 1, 2012, pp. 81-89. doi:10.1016/j.ijepes.2011.08.025
[8] L. K. Biswas, M. H. Rahman and S. Haque, “A Low Cost
Caracteristics Analyzer of PV Module,” Developments in
Renewable Energy Technology (ICDRET), 2012 2nd In-
ternational Conference, 2012, pp. 1-5.
[9] Energy Revolution-YUDU, [Online], Available at:
http://content.yudu.com/Library/A1tlhk/Rexelrenewables
Sharp/resources/12.htm. Accessed: 2012-04-12,
[10] N. Lemmer, “Using Energy Meters as a Tool to Improve
Energy-efficiency,” 25 Degrees in Africa, 2012, pp.
28-29.
[11] S. C. Brofferio, A. Antonini, G. Galimberti and D. Galeri,
“A Method for Estimating and Monitoring the Power
Generated by a Photovoltaic Module Based on Super-
vised Adaptive Neural networks,” Smart Measurements
for Future Grids (SMFG), 2011 IEEE International Con-
ference, 2011, pp. 148-153.
[12] D. Sera, R. Teodorescu and P. Rodriguez, “Photovoltaic
Module Diagnostics by Series Resistance Monitoring and
Temperature and Rated Power Estimation,” Industrial
Electronics, IECON 2008, 34th Annual Conference of
IEEE, 2008, pp. 2195-2199.
[13] N. Pearsall and B. Atanasiu, “The European PV System
Monitoring Guidelines - Modernisation under the PER-
FORMANCE Project,” Photovoltaic Specialists Confer-
ence (PVSC), 2009 34th IEEE, 2009, pp. 000256-000261.
[14] IEEE Xplore, Data Loggers, [Online], Available at:
http://ieeexplore.ieee.org. Accessed: 1 July, 2012
[15] PICO Technology, Homepage, [Online], Available at:
http://www.picotech.com/index.html. Accessed: 11 July,
2012
Copyright © 2013 SciRes. EPE