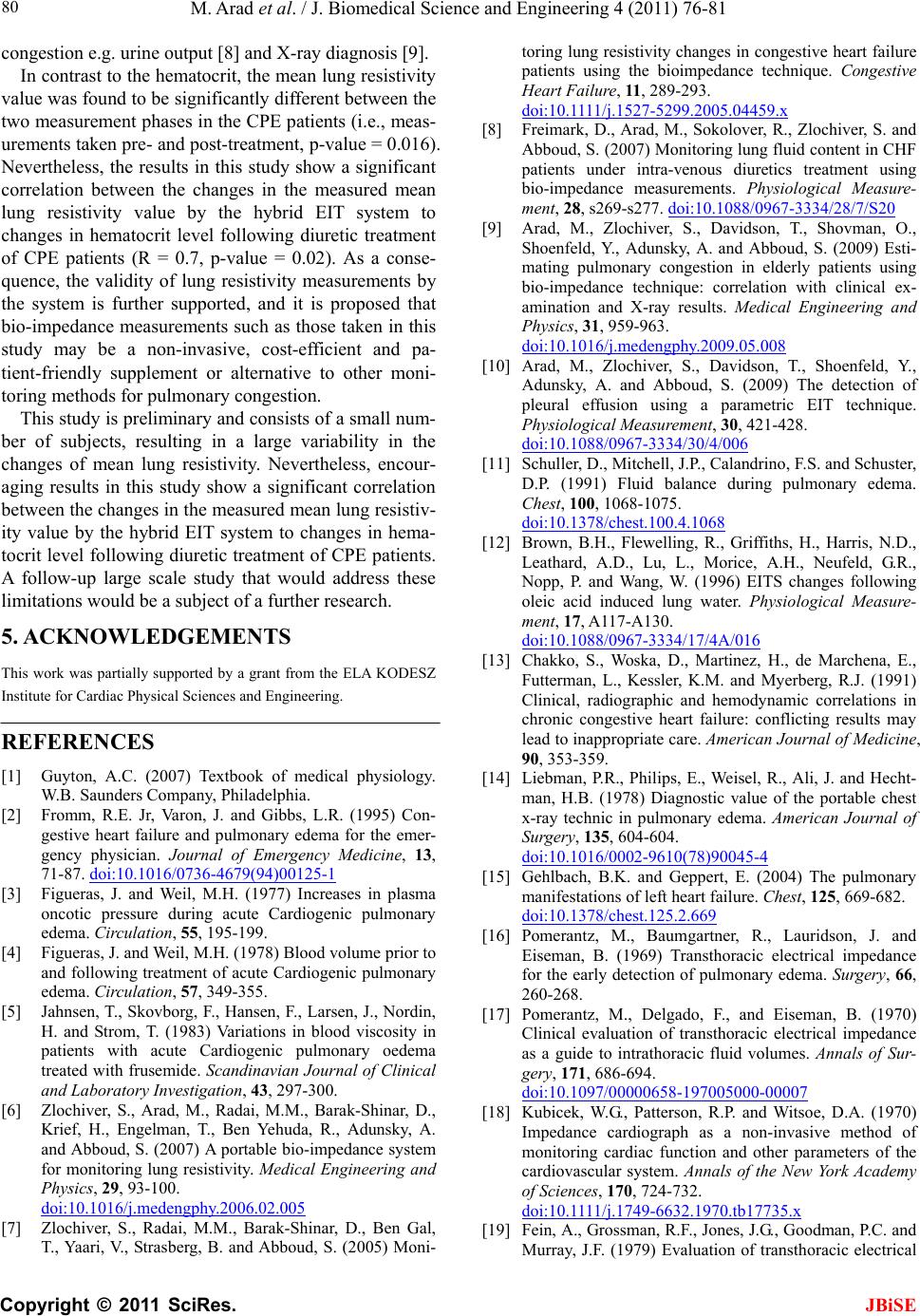
M. Arad et al. / J. Biomedical Science and Engineering 4 (2011) 76-81
Copyright © 2011 SciRes. JBiSE
80
congestion e.g. urine output [8] and X-ray diagnosis [9].
In contrast to the hematocrit, the mean lung resistivity
value was found to be significantly different between the
two measurement phases in the CPE patients (i.e., meas-
urements taken pre- and post-treatment, p-value = 0.016).
Nevertheless, the results in this study show a significant
correlation between the changes in the measured mean
lung resistivity value by the hybrid EIT system to
changes in hematocrit level following diuretic treatment
of CPE patients (R = 0.7, p-value = 0.02). As a conse-
quence, the validity of lung resistivity measurements by
the system is further supported, and it is proposed that
bio-impedance measurements such as those taken in this
study may be a non-invasive, cost-efficient and pa-
tient-friendly supplement or alternative to other moni-
toring methods for pulmonary congestion.
This study is preliminary and consists of a small num-
ber of subjects, resulting in a large variability in the
changes of mean lung resistivity. Nevertheless, encour-
aging results in this study show a significant correlation
between the changes in the measured mean lung resistiv-
ity value by the hybrid EIT system to changes in hema-
tocrit level following diuretic treatment of CPE patients.
A follow-up large scale study that would address these
limitations would be a subject of a further research.
5. ACKNOWLEDGEMENTS
This work was partially supported by a grant from the ELA KODESZ
Institute for Cardiac Physical Sciences and Engineering.
REFERENCES
[1] Guyton, A.C. (2007) Textbook of medical physiology.
W.B. Saunders Company, Philadelphia.
[2] Fromm, R.E. Jr, Varon, J. and Gibbs, L.R. (1995) Con-
gestive heart failure and pulmonary edema for the emer-
gency physician. Journal of Emergency Medicine, 13,
71-87. doi:10.1016/0736-4679(94)00125-1
[3] Figueras, J. and Weil, M.H. (1977) Increases in plasma
oncotic pressure during acute Cardiogenic pulmonary
edema. Circulation, 55, 195-199.
[4] Figueras, J. and Weil, M.H. (1978) Blood volume prior to
and following treatment of acute Cardiogenic pulmonary
edema. Circulation, 57, 349-355.
[5] Jahnsen, T., Skovborg, F., Hansen, F., Larsen, J., Nordin,
H. and Strom, T. (1983) Variations in blood viscosity in
patients with acute Cardiogenic pulmonary oedema
treated with frusemide. Scandinavian Journal of Clinical
and Laboratory Investigation, 43, 297-300.
[6] Zlochiver, S., Arad, M., Radai, M.M., Barak-Shinar, D.,
Krief, H., Engelman, T., Ben Yehuda, R., Adunsky, A.
and Abboud, S. (2007) A portable bio-impedance system
for monitoring lung resistivity. Medical Engineering and
Physics, 29, 93-100.
doi:10.1016/j.medengphy.2006.02.005
[7] Zlochiver, S., Radai, M.M., Barak-Shinar, D., Ben Gal,
T., Yaari, V., Strasberg, B. and Abboud, S. (2005) Moni-
toring lung resistivity changes in congestive heart failure
patients using the bioimpedance technique. Congestive
Heart Failure, 11 , 289-293.
doi:10.1111/j.1527-5299.2005.04459.x
[8] Freimark, D., Arad, M., Sokolover, R., Zlochiver, S. and
Abboud, S. (2007) Monitoring lung fluid content in CHF
patients under intra-venous diuretics treatment using
bio-impedance measurements. Physiological Measure-
ment, 28, s269-s277. doi:10.1088/0967-3334/28/7/S20
[9] Arad, M., Zlochiver, S., Davidson, T., Shovman, O.,
Shoenfeld, Y., Adunsky, A. and Abboud, S. (2009) Esti-
mating pulmonary congestion in elderly patients using
bio-impedance technique: correlation with clinical ex-
amination and X-ray results. Medical Engineering and
Physics, 31, 959-963.
doi:10.1016/j.medengphy.2009.05.008
[10] Arad, M., Zlochiver, S., Davidson, T., Shoenfeld, Y.,
Adunsky, A. and Abboud, S. (2009) The detection of
pleural effusion using a parametric EIT technique.
Physiological Measurement, 30, 421-428.
doi:10.1088/0967-3334/30/4/006
[11] Schuller, D., Mitchell, J.P., Calandrino, F.S. and Schuster,
D.P. (1991) Fluid balance during pulmonary edema.
Chest, 100, 1068-1075.
doi:10.1378/chest.100.4.1068
[12] Brown, B.H., Flewelling, R., Griffiths, H., Harris, N.D.,
Leathard, A.D., Lu, L., Morice, A.H., Neufeld, G.R.,
Nopp, P. and Wang, W. (1996) EITS changes following
oleic acid induced lung water. Physiological Measure-
ment, 17, A117-A130.
doi:10.1088/0967-3334/17/4A/016
[13] Chakko, S., Woska, D., Martinez, H., de Marchena, E.,
Futterman, L., Kessler, K.M. and Myerberg, R.J. (1991)
Clinical, radiographic and hemodynamic correlations in
chronic congestive heart failure: conflicting results may
lead to inappropriate care. American Journal of Medicine,
90, 353-359.
[14] Liebman, P.R., Philips, E., Weisel, R., Ali, J. and Hecht-
man, H.B. (1978) Diagnostic value of the portable chest
x-ray technic in pulmonary edema. American Journal of
Surgery, 135, 604-604.
doi:10.1016/0002-9610(78)90045-4
[15] Gehlbach, B.K. and Geppert, E. (2004) The pulmonary
manifestations of left heart failure. Chest, 125, 669-682.
doi:10.1378/chest.125.2.669
[16] Pomerantz, M., Baumgartner, R., Lauridson, J. and
Eiseman, B. (1969) Transthoracic electrical impedance
for the early detection of pulmonary edema. Surgery, 66,
260-268.
[17] Pomerantz, M., Delgado, F., and Eiseman, B. (1970)
Clinical evaluation of transthoracic electrical impedance
as a guide to intrathoracic fluid volumes. Annals of Sur-
gery, 171, 686-694.
doi:10.1097/00000658-197005000-00007
[18] Kubicek, W.G., Patterson, R.P. and Witsoe, D.A. (1970)
Impedance cardiograph as a non-invasive method of
monitoring cardiac function and other parameters of the
cardiovascular system. Annals of the New York Academy
of Sciences, 170, 724-732.
doi:10.1111/j.1749-6632.1970.tb17735.x
[19] Fein, A., Grossman, R.F., Jones, J.G., Goodman, P.C. and
Murray, J.F. (1979) Evaluation of transthoracic electrical