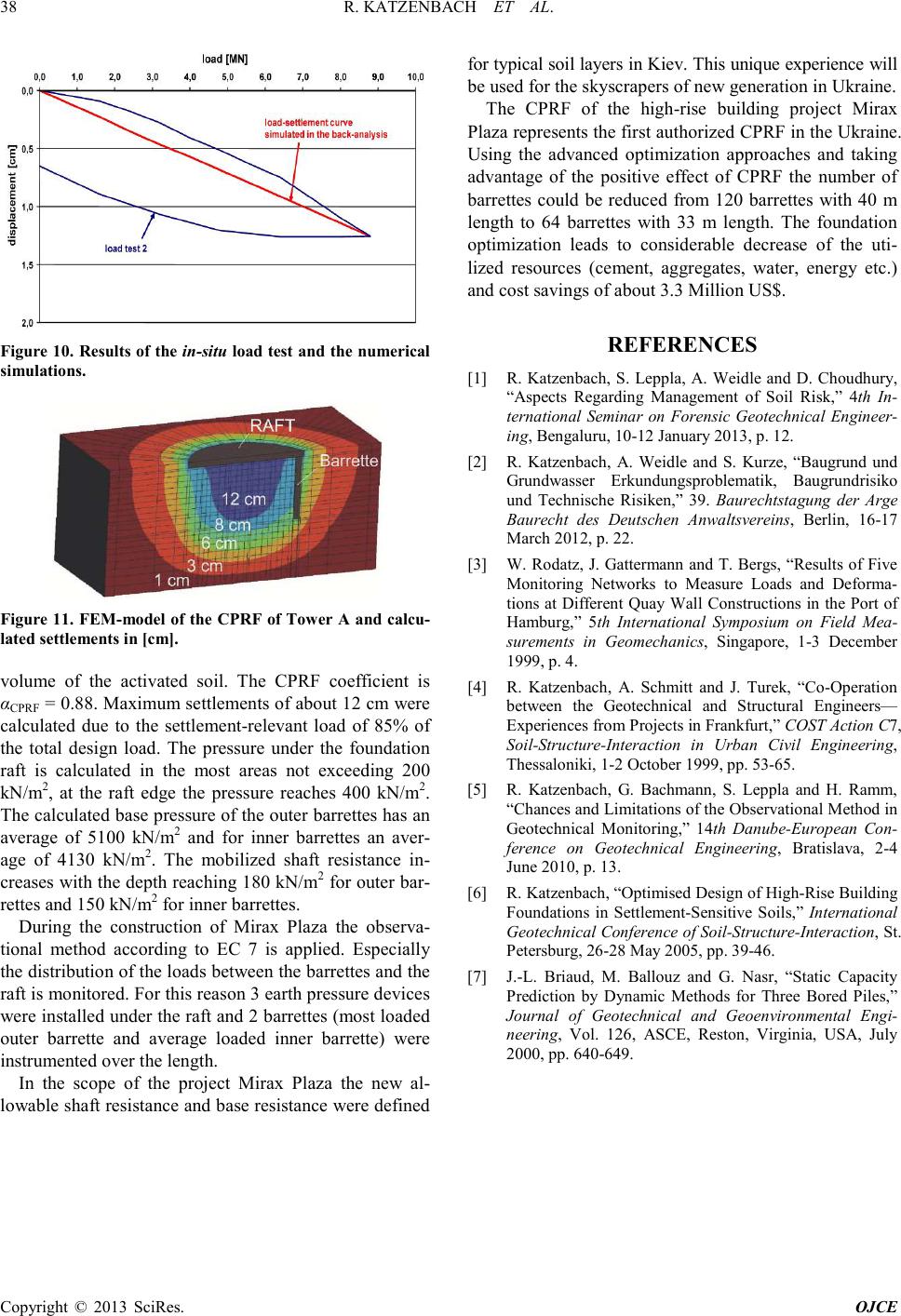
R. KATZENBACH ET AL.
Copyright © 2013 SciRes. OJCE
Figure 10. Results of the in-situ load test and the nu merical
si mulati ons.
Figure 11. FEM-model of the CPRF of Tow er A and calcu-
lated settlements in [cm].
volume of the activated soil. The CPRF coefficient is
αCPRF = 0.88. Maximum settlements of about 12 cm were
calculated due to the settlement-relevant load of 85% of
the total design load. The pressure under the foundation
raft is calculated in the most areas not exceeding 200
kN/m2, at the raft edge the pressure reaches 400 kN/m2.
The calculated base pressure of the outer barrettes has an
average of 5100 kN/m2 and for inner barrettes an aver-
age of 4130 kN/m2. The mobilized shaft resistance in-
creases with the depth reaching 180 kN/m2 for outer bar-
rettes and 150 kN/m2 for inner barrettes.
During the construction of Mirax Plaza the observa-
tional method according to EC 7 is applied. Especially
the distributio n of the loads bet ween the barrettes and the
raft is monitored. For this reason 3 earth pressure devices
were installed under the raft and 2 barrettes (most loaded
outer barrette and average loaded inner barrette) were
instrume nted over the length.
In the scope of the project Mirax Plaza the new al-
lowable shaft resistance and base resistance were defined
for t ypic al soi l layer s in K iev. T his uni que e xper ienc e wil l
be used fo r the skyscra per s of new generation in Ukraine.
The CPRF of the high-rise building project Mirax
Plaza represents the first authorized CPRF in the Ukraine.
Using the advanced optimization approaches and taking
advantage of the positive effect of CPRF the number of
barrettes could be reduced from 120 barrettes with 40 m
length to 64 barrettes with 33 m length. The foundation
optimization leads to considerable decrease of the uti-
lized resources (cement, aggregates, water, energy etc.)
and cost savings of about 3.3 Million US$.
REFERENCES
[1] R. Katzenbach, S. Leppla, A. Weidle and D. Choudhury,
“Aspects Regarding Management of Soil Risk,” 4th In-
ternational Seminar on Forensic Geotechnical Engineer-
ing, Bengaluru, 10-12 Januar y 2013, p. 12 .
[2] R. Katzenbach, A. Weidle and S. Kurze, “Baugrund und
Grundwasser Erkundungsproblematik, Baugrundrisiko
und Technische Risiken,” 39. Baurechtstagung der Arge
Baurecht des Deutschen Anwaltsvereins, Berlin, 16-17
March 2012, p. 22.
[3] W. Rodatz, J. Gattermann and T. Bergs, “Results of Five
Monitoring Networks to Measure Loads and Deforma-
tions at Different Quay Wall Constructions in the Port of
Hamburg,” 5th International Symposium on Field Mea-
surements in Geomech anics, Singapore, 1-3 December
1999, p. 4.
[4] R. Katzenbach, A. Schmitt and J. Turek, “Co-Operation
between the Geotechnical and Structural Engineers—
Experien ces fro m P rojects in Frankfurt ,” COST Action C7,
Soil-Structure-Interaction in Urban Civil Engineering,
Thessaloniki, 1-2 Octobe r 19 99, pp. 53 -65.
[5] R. Katzenbach, G. Bachmann, S. Leppla and H. Ramm,
“Chances and Limitations of the Observational Method in
Geotechnical Monitoring,” 14th Danube-European Con-
ference on Geotechnical Engineering, Bratislava, 2-4
June 201 0, p. 13.
[6] R. K atzen bach , “Opt imised Desi gn of High-Rise Building
Foundations in Settlement-Sensitive Soils,” International
Geotechnical Conference of Soil-Structure-Interaction, St.
Petersburg, 26-28 May 2005, pp. 39-46.
[7] J.-L. Briaud, M. Ballouz and G. Nasr, “Static Capacity
Prediction by Dynamic Methods for Three Bored Piles,”
Journal of Geotechnical and Geoenvironmental Engi-
neerin g, Vol. 126, ASCE, Reston, Virginia, USA, July
2000, pp . 640-649.