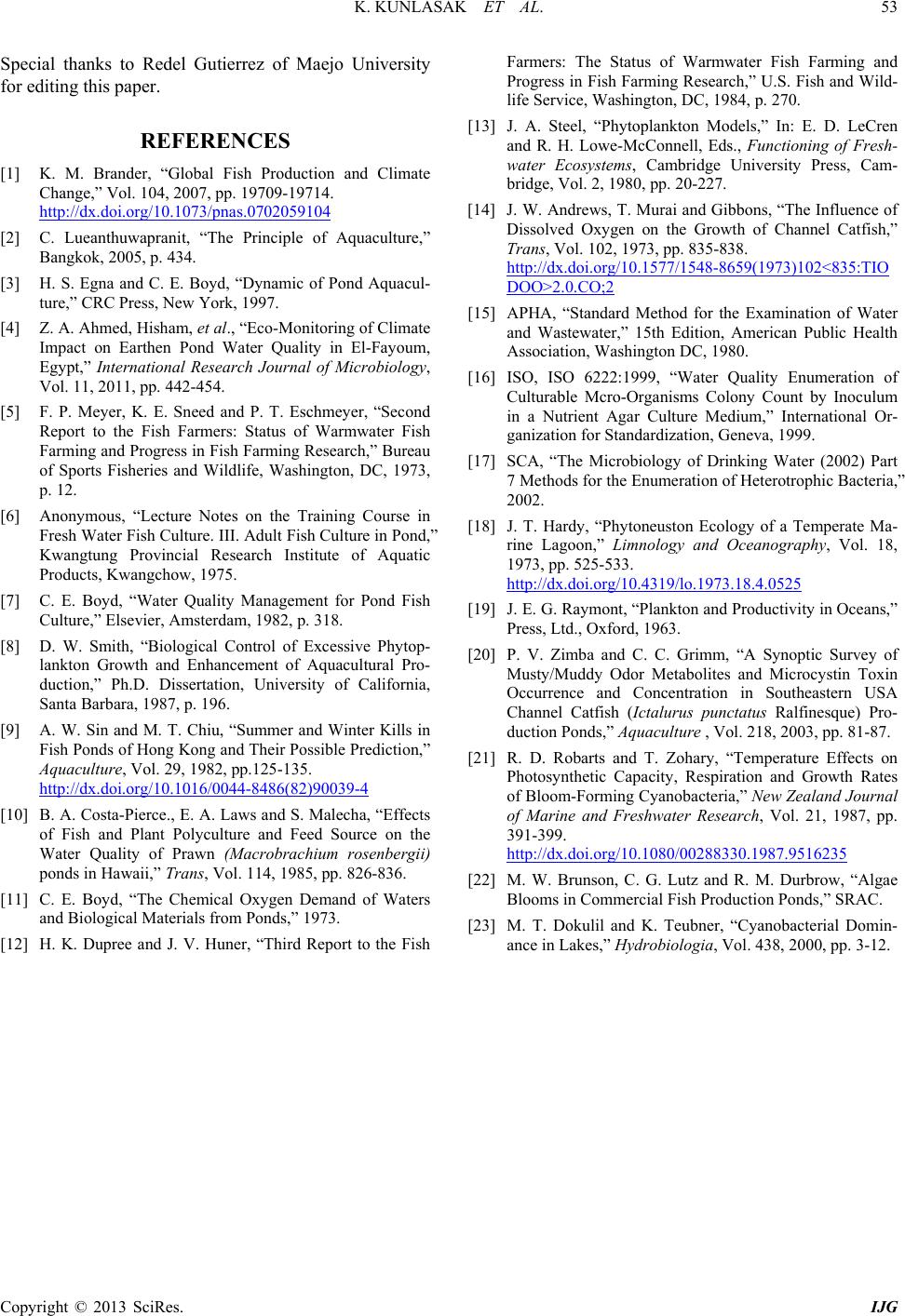
K. KUNLASAK ET AL.
Copyright © 2013 SciRes. IJG
Special thanks to Redel Gutierrez of Maejo University
for editing this paper.
REFERENCES
[1] K. M. Brander, “Global Fish Production and Climate
Change,” Vol. 104, 2007, pp. 19709-19714.
http://dx.doi.org/10.1073/pnas.0702059104
[2] C. Lueanthuwapranit, “The Principle of Aquaculture,”
Bangkok, 2005, p. 434.
[3] H. S. Egna and C. E. Boyd, “Dynamic of Pond Aquacul-
ture,” CRC Press, New York, 1997.
[4] Z. A. Ahmed, Hisham, et al., “Eco-Monitoring of Climate
Impact on Earthen Pond Water Quality in El-Fayoum,
Egypt,” International Research Journal of Microbiology,
Vol. 11, 2011, pp. 442-454.
[5] F. P. Meyer, K. E. Sneed and P. T. Eschmeyer, “Second
Report to the Fish Farmers: Status of Warmwater Fish
Farming and Progress in Fish Farming Research,” Bureau
of Sports Fisheries and Wildlife, Washington, DC, 1973,
p. 12.
[6] Anonymous, “Lecture Notes on the Training Course in
Fresh Water Fish Culture. III. Adult Fish Culture in Pond,”
Kwangtung Provincial Research Institute of Aquatic
Products, Kwangchow, 1975.
[7] C. E. Boyd, “Water Quality Management for Pond Fish
Culture,” Elsevier, Amsterdam, 1982, p. 318.
[8] D. W. Smith, “Biological Control of Excessive Phytop-
lankton Growth and Enhancement of Aquacultural Pro-
duction,” Ph.D. Dissertation, University of California,
Santa Barbara, 1987, p. 196.
[9] A. W. Sin and M. T. Chiu, “Summer and Winter Kills in
Fish Ponds of Hong Kong and Their Possible Prediction,”
Aquaculture, Vol. 29, 1982, pp.125-135.
http://dx.doi.org/10.1016/0044-8486(82)90039-4
[10] B. A. Costa-Pierce., E. A. Laws and S. Malecha, “Effects
of Fish and Plant Polyculture and Feed Source on the
Water Quality of Prawn (Macrobrachium rosenbergii)
ponds in Hawaii,” Trans, Vol. 114, 1985, pp. 826-836.
[11] C. E. Boyd, “The Chemical Oxygen Demand of Waters
and Biological Materials from Ponds,” 1973.
[12] H. K. Dupree and J. V. Huner, “Third Report to the Fish
Farmers: The Status of Warmwater Fish Farming and
Progress in Fish Farming Research,” U.S. Fish and Wild-
life Service, Washington, DC, 1984, p. 270.
[13] J. A. Steel, “Phytoplankton Models,” In: E. D. LeCren
and R. H. Lowe-McConnell, Eds., Functioning of Fresh-
water Ecosystems, Cambridge University Press, Cam-
bridge, Vol. 2, 1980, pp. 20-227.
[14] J. W. Andrews, T. Murai and Gibbons, “The Influence of
Dissolved Oxygen on the Growth of Channel Catfish,”
Trans, Vol. 102, 1973, pp. 835-838.
http://dx.doi.org/10.1577/1548-8659(1973)102<835:TIO
DOO>2.0.CO;2
[15] APHA, “Standard Method for the Examination of Water
and Wastewater,” 15th Edition, American Public Health
Association, Washington DC, 1980.
[16] ISO, ISO 6222:1999, “Water Quality Enumeration of
Culturable Mcro-Organisms Colony Count by Inoculum
in a Nutrient Agar Culture Medium,” International Or-
ganization for Standardization, Geneva, 1999.
[17] SCA, “The Microbiology of Drinking Water (2002) Part
7 Methods for the Enumeration of Heterotrophic Bacteria,”
2002.
[18] J. T. Hardy, “Phytoneuston Ecology of a Temperate Ma-
rine Lagoon,” Limnology and Oceanography, Vol. 18,
1973, pp. 525-533.
http://dx.doi.org/10.4319/lo.1973.18.4.0525
[19] J. E. G. Raymont, “Plankton and Productivity in Oceans,”
Press, Ltd., Oxford, 1963.
[20] P. V. Zimba and C. C. Grimm, “A Synoptic Survey of
Musty/Muddy Odor Metabolites and Microcystin Toxin
Occurrence and Concentration in Southeastern USA
Channel Catfish (Ictalurus punctatus Ralfinesque) Pro-
duction Ponds,” Aquaculture , Vol. 218, 2003, pp. 81-87.
[21] R. D. Robarts and T. Zohary, “Temperature Effects on
Photosynthetic Capacity, Respiration and Growth Rates
of Bloom-Forming Cyanoba cteria,” New Zealand Journal
of Marine and Freshwater Research, Vol. 21, 1987, pp.
391-399.
http://dx.doi.org/10.1080/00288330.1987.9516235
[22] M. W. Brunson, C. G. Lutz and R. M. Durbrow, “Algae
Blooms in Commercial Fish Production Ponds,” SRAC.
[23] M. T. Dokulil and K. Teubner, “Cyanobacterial Domin-
ance in Lakes,” Hydrobiologia, Vol. 438, 2000, pp. 3-12.