 American Journal of Plant Sciences, 2013, 4, 1983-1989 http://dx.doi.org/10.4236/ajps.2013.410246 Published Online October 2013 (http://www.scirp.org/journal/ajps) 1983 Padina pavonica for the Removal of Dye from Polluted Water Eman M. Fakhry Department of Botany and Microbiology, Faculty of Science, Alexandria University, Alexandria, Egypt. Email: emfakhr@hotmail.com Received July 29th, 2013; revised August 29th, 2013; accepted September 15th, 2013 Copyright © 2013 Eman M. Fakhry. This is an open access article distributed under the Creative Commons Attribution License, which permits unrestricted use, distribution, and reproduction in any medium, provided the original work is properly cited. ABSTRACT The adsorption of fast yellow dye onto dried biomass Padina pavonica was studied in batch experiments. The amount of dye adsorbed (mg/g) was increased with the increase in initial dye concentration. An equilibrium time of about 90 min was achieved for dye concentrations ranging from 5 to 160 mg/L with maximum removal percentage of 73.2%. Pseudo-first and second order kinetic models have been used to analyze the adsorption data. The pseudo second-order kinetic model adequately described the adsorption data with correlation coefficient between 0.96 and 1.084. Fourier transform infra-red analysis demonstrated the chelating character of the dye molecule to different functionalities groups of the alga. Stirring speed higher than 50 rpm revealed no significant changes in dye adsorption. Temperature ranging from 15˚C to 65˚C showed stability followed by a decrease in adsorption. Scanning electron microscopy of adsorbent particles showed a high surface porosity allowing the free passage of dye molecules. Keywords: Adsorption; Dried Padina pavonica; Equilibrium; Fast Yellow Dye; Functional Groups 1. Introduction Pollution is an environmental problem of worldwide concern. Consuming of water by agricultural, industrial and domestic sectors resulted in the generation of large amounts of waste water containing a number of pollut- ants [1]. Organic dyes in water are one of the important classes of pollutants [2]. It is difficult to treat dyes as they have a complex molecular structure which makes them more stable and difficult to be biodegraded [3,4]. The first known use of organic colorant appeared nearly 4000 years ago, when the blue dye indigo was found in the wrapping of mummies in Egyptian tombs [5]. There are more than 100,000 commercial dyes with a roughly estimated production of millions of tons per year [6-8]. Azo-dyes are one of the important classes of dyes which are characterized by an azo group consisting of two ni- trogen atoms (–N═N–) as the chromophore in the mole- cule [9]. These dyes find application in many industries including textile, cosmetic, food colorants, printing, and pharmaceutical industries. The effluents of these indus- tries tend to contain dyes in sufficient quantities. They cause coloration of water bodies once released into the aquatic environment and subsequently interfere with the transmission of light affecting aquatic communities’ life [10]. Various treatment technologies such as precipita- tion, ion exchange, and adsorption have been employed to remove dye pollutants from aqueous solutions. Ad- sorption using low cost biological materials is generally regarded as an effective technique for the treatment of dye-containing wastewater [11-16]. One of the promising biological materials is the use of nonviable dried organ- isms. They have been proposed as potential sorbents; since they are dead materials having no need of nutrition to keep the biomass [17]. Algae have been found to be po- tential and suitable biosorbent because of their fast and easy growth as well as their wide availability. There were various researches on the usage of micro and macro algae as sorbent materials [2,18-21]. The sorption capability of algae has been attributed to their cell walls which are often porous and allow the passage of molecules and ions in aqueous solutions [22,23]. Essentially, the extracellu- lar biopolymers of Phaeophyta are predominately alginic acid or alginate with a smaller amount of fucoidan which seems easily permeable for small ions [24]. Whereas the Rhodophyta contain a number of sulfated galactans like agar, carregeenan and porphyran [25]. Thus, the adsorp- tion capacity along with the dye sorption process onto the surface is due to the different long chain extracellular bi- opolymers. Furthermore, algae functional groups found Copyright © 2013 SciRes. AJPS
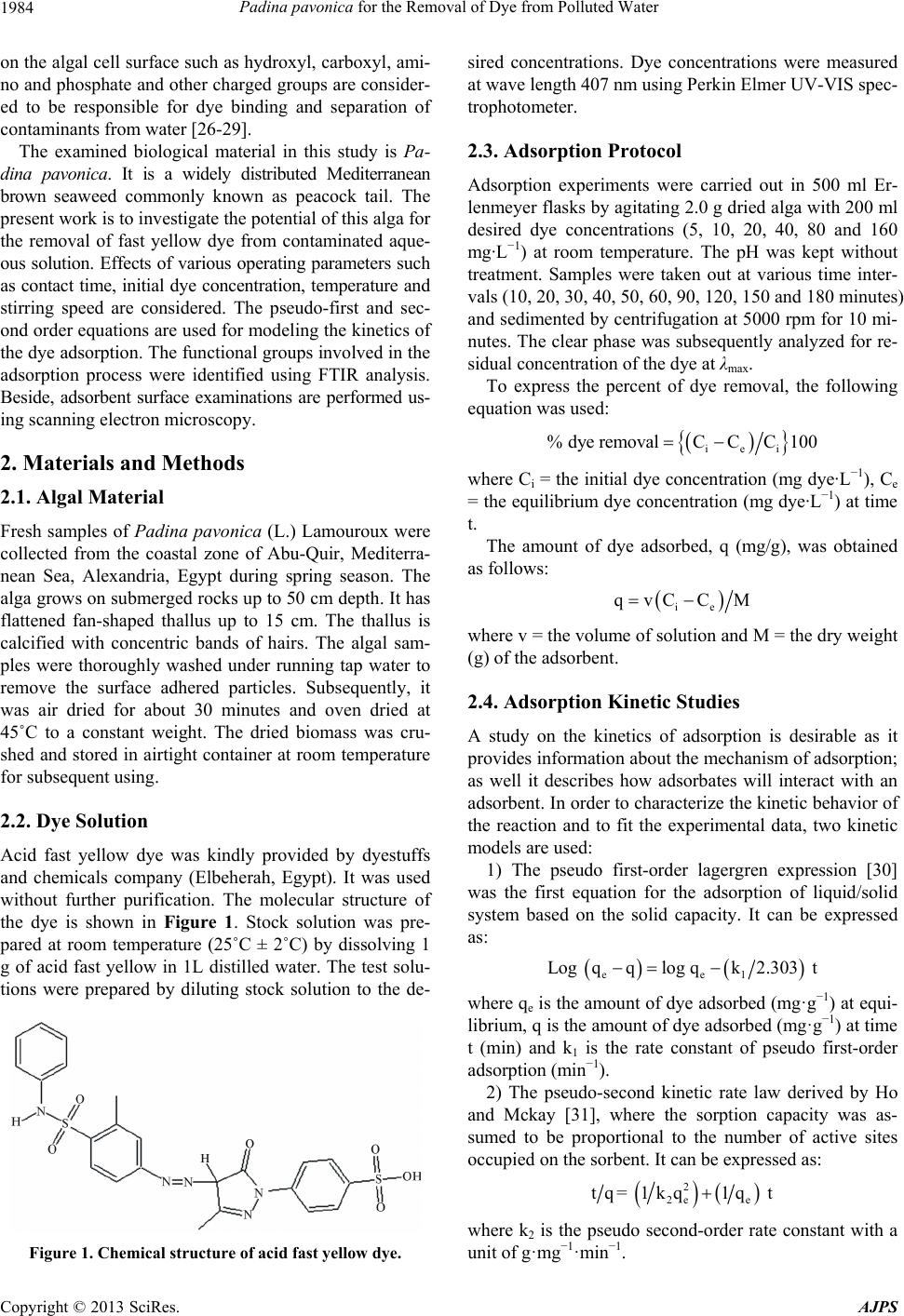 Padina pavonica for the Removal of Dye from Polluted Water 1984 on the algal cell surface such as hydroxyl, carboxyl, ami- no and phosphate and other charged groups are consider- ed to be responsible for dye binding and separation of contaminants from water [26-29]. The examined biological material in this study is Pa- dina pavonica. It is a widely distributed Mediterranean brown seaweed commonly known as peacock tail. The present work is to investigate the potential of this alga for the removal of fast yellow dye from contaminated aque- ous solution. Effects of various operating parameters such as contact time, initial dye concentration, temperature and stirring speed are considered. The pseudo-first and sec- ond order equations are used for modeling the kinetics of the dye adsorption. The functional groups involved in the adsorption process were identified using FTIR analysis. Beside, adsorbent surface examinations are performed us- ing scanning electron microscopy. 2. Materials and Methods 2.1. Algal Material Fresh samples of Padina pavonica (L.) Lamouroux were collected from the coastal zone of Abu-Quir, Mediterra- nean Sea, Alexandria, Egypt during spring season. The alga grows on submerged rocks up to 50 cm depth. It has flattened fan-shaped thallus up to 15 cm. The thallus is calcified with concentric bands of hairs. The algal sam- ples were thoroughly washed under running tap water to remove the surface adhered particles. Subsequently, it was air dried for about 30 minutes and oven dried at 45˚C to a constant weight. The dried biomass was cru- shed and stored in airtight container at room temperature for subsequent using. 2.2. Dye Solution Acid fast yellow dye was kindly provided by dyestuffs and chemicals company (Elbeherah, Egypt). It was used without further purification. The molecular structure of the dye is shown in Figure 1. Stock solution was pre- pared at room temperature (25˚C ± 2˚C) by dissolving 1 g of acid fast yellow in 1L distilled water. The test solu- tions were prepared by diluting stock solution to the de- Figure 1. Chemical structure of acid fast yellow dye. sired concentrations. Dye concentrations were measured at wave length 407 nm using Perkin Elmer UV-VIS spec- trophotometer. 2.3. Adsorption Protocol Adsorption experiments were carried out in 500 ml Er- lenmeyer flasks by agitating 2.0 g dried alga with 200 ml desired dye concentrations (5, 10, 20, 40, 80 and 160 mg· L −1) at room temperature. The pH was kept without treatment. Samples were taken out at various time inter- vals (10, 20, 30, 40, 50, 60, 90, 120, 150 and 180 minutes) and sedimented by centrifugation at 5000 rpm for 10 mi- nutes. The clear phase was subsequently analyzed for re- sidual concentration of the dye at λmax. To express the percent of dye removal, the following equation was used: iei % dye removalCCC100 where Ci = the initial dye concentration (mg dye·L−1), Ce = the equilibrium dye concentration (mg dye·L−1) at time t. The amount of dye adsorbed, q (mg/g), was obtained as follows: ie qvCCM where v = the volume of solution and M = the dry weight (g) of the adsorbent. 2.4. Adsorption Kinetic Studies A study on the kinetics of adsorption is desirable as it provides information about the mechanism of adsorption; as well it describes how adsorbates will interact with an adsorbent. In order to characterize the kinetic behavior of the reaction and to fit the experimental data, two kinetic models are used: 1) The pseudo first-order lagergren expression [30] was the first equation for the adsorption of liquid/solid system based on the solid capacity. It can be expressed as: ee1 Log qqlog qk2.3t 30 where qe is the amount of dye adsorbed (mg·g−1) at equi- librium, q is the amount of dye adsorbed (mg·g−1) at time t (min) and k1 is the rate constant of pseudo first-order adsorption (min−1). 2) The pseudo-second kinetic rate law derived by Ho and Mckay [31], where the sorption capacity was as- sumed to be proportional to the number of active sites occupied on the sorbent. It can be expressed as: 2 e2e tq= 1k1qtq where k2 is the pseudo second-order rate constant with a unit of g·mg−1·min−1. Copyright © 2013 SciRes. AJPS
 Padina pavonica for the Removal of Dye from Polluted Water 1985 The best-fit equilibrium model was selected based on the linear squared regression correlation coefficient, R2, values. 2.5. Sorbent Characterization The functional groups of Padina pavonica were inter- preted using the fourier transform infrared (FTIR) tech- nique. A sample of adsorbent was mixed with approxi- mately 0.5 g potassium bromide in the sample disk short- ly before recording the spectra. The spectra were collec- ted by Perkin-Elmer spectrum RXIFT-IR System within the range 500 - 4000 cm−1. 2.6. Scanning Electron Microscopy To study the surface texture, porous properties and mor- phology of the algal particles, scanning electron micro- scope examination was chosen. Samples were coated with a thin electric conductive gold film prior to use. Exami- nations of surface texture were performed using Jeol JSM-5300 scanning electron microscope. 3. Results and Discussion 3.1. Dye Concentrations and Sorbent Contact The relationship between the time profiles and the dye removal percentage at various initial dye concentrations is shown in Figure 2. The adsorption rate of dye in- creased from 18.8% to 73.23% as the dye concentration increased from 5 to 160 mg/L. This can be attributed to an increase in surface area of the biosorbent, which in turn increases the binding sites. Generally, the adsorption rate is strongly influenced by several parameters includ- ing the state of the solid, availability of solute, and inter- ference between reactive binding sites [32]. Figure 2. Effect of contact time on the adsorption rate of fast yellow by Padina pavonica. 3.2. Sorption Equilibrium Studies In Figure 3, adsorption capacity (q) is correlated with time (min) at different initial dye concentrations (mg/L) keeping biosorbent weight as constant. It shows that most of the dye is adsorbed to achieve adsorption equilibrium in about 90 min except for 20 mg/L dye concentration; it was 120 min. At this point, the amount of dye being ad- sorbed onto the alga was in equilibrium state and no in- crease in loading capacity on the external surface of the adsorbent can take place. The overall adsorption is seen to consist of higher adsorption rate at the early period which may be due to the availability of more adsorption sites on the adsorbent surface [33]. As time passes, the adsorption rate is slow down due to the accumulation of the dye molecules in the vacant sites. This observation is consistent with the concept of non-homogeneity of the algal surface, which contains a variety of active sites. It serves as adsorption sites and may differ both with re- spect to the strength of the dye sorptive bond and the rate of adsorption onto the active sites [34,35]. Also it is more likely to note that the external diffusion is one of the rate-controlling steps of the initial fast adsorption of the dye onto the biosorbent [36]. In general, adsorption may be assumed to involve migration of dye from the bulk of the solution to the surface of adsorbent followed by dif- fusion of the adsorbate through the boundary layer and into the interior pore structure of the surface of adsorbent species. 3.3. Adsorption Kinetics The principle behind the adsorption kinetics involves the search for a best model that well represents the interpre- tation of adsorption data. The pseudo-first and second order kinetic models, which are widely used to describe the adsorption kinetics, were applied. The best-fit model was determined based on the linear regression correlation coefficient values. Since the linear dependency was not Figure 3. Adsorption capacity of fast yellow onto Padina pa- vonica. Copyright © 2013 SciRes. AJPS
 Padina pavonica for the Removal of Dye from Polluted Water 1986 obtained between log (qe − q) and t (Figure 4), it can be said that the first-order equation of Lagergren does not fit well to the whole range of contact time. This suggests that the adsorption of dye onto the algal biomass is not a first-order reaction. The second order rate constant k2 and qe where determined by plotting tq versus t (Figure 5). Correlation coefficient (R2) together with the adsorption rate k2 show that the pseudo second-order model is well in line with the tested experimental data. The linear plot between log (qe − q) and t was detected and the correla- tion coefficients are nearly equal to 1 (Table 1). In the view of these results, the pseudo-second order kinetic model provided a good correlation for the adsorption of fast yellow onto the biosorbent in contrast to the pseudo- first order model. 3.4. Sorbent Characterization FTIR spectroscopy involves collecting absorption infor- mation in the form of spectra. These spectra specify the absorption signals and the corresponding functional groups on the pure biomass surface to be compared with Figure 4. Pseudo-fist-order kinetic sorption of fast yellow onto Padina pavonica. Figure 5. Plot of the pseudo-second-order model at different initial dye concentrations. Table 1. Pseudo second order kinetic constants for the ad- sorption of fast yellow onto algal biomass. Dye concentrationqe k 2 (mg/L) (mg/g) (g/mg·min) R2 5 0.325 0.0344 0.971 10 0.65 0.017 1.05 20 1.319 0.0063 1.084 40 2.95 0.0037 0.988 80 6.12 0.0018 0.96 160 11.72 0.00094 1.009 the referenced data of IR absorption. The FTIR spectra are in the range of 500 - 4000 cm−1. It exhibits absorption bands at 3250, 2550, 3400, 1200, 3000, 1670 and 1128 cm−1 indicating the presence of OH, COOH, NH2, S=O, C-H, C=O and C-O groups, respectively (Figure 6). This assumes that the adsorbent consists of a heterogeneous surface composed of different classes of adsorption sites. These sites provide information on the nature of cell wall and dye interaction. As well, they are involved in almost all potential binding mechanisms. It was found that car- boxyl and hydroxyl groups are mainly responsible for dye sorption on Padina. However, other group function- alities showed less contribution in binding with dye. This result agreed with Murphy et al. [37] which reported that metal binding to brown seaweeds showed significant participation of carboxyl group accompanied by interac- tion of other groups. In fact, the relative importance of these functional groups depend on factors such as the quantity of sites, their accessibility, chemical state and affinity between site and dye [37,38]. 3.5. Effect of Stirring Speed The adsorption of dye as a function of stirring speed was investigated at room temperature and pH solution. Seven stirring speeds from 0 (without agitation) to 250 rpm were studied to determine the significant speed required for the optimal dye adsorption on dried alga. Figure 7 shows that maximum dye removal percentage was ob- tained at 50 rpm stirring speed. Exceeding this speed, there is no significant effect on the adsorption and the dye removal percentage held almost with no variety. These results indicate that the increase in stirring speed im- proves the diffusion of dye molecules toward the surface of the seaweed. However, stirring speed up to 50 rpm is sufficient to assure that all the cell wall binding sites are accessible for dye uptake. Afterward, the effect of exter- nal film diffusion on adsorption rate is not significant [34,39]. 3.6. Effect of Temperature The temperature dependence of dye adsorption onto the dried biomass was studied at 15˚C, 25˚C, 35˚C, 45˚C, Copyright © 2013 SciRes. AJPS
 Padina pavonica for the Removal of Dye from Polluted Water 1987 Figure 6. Peaks for nonliving biomass of Padina pavonica obtained from FTIR analysis. Figure 7. Effect of stirring speed on adsorption rate of dye onto non-living biomass. 55˚C and 65˚C keeping other parameters as constant. The temperature effect shown in Figure 8 recorded relatively slight differences in dye adsorption from 68.7% to 69.5% as the temperature increase from 15˚C to 35˚C. This may be due to an increase in the mobility of the adsorbate molecules and the existence of the pores on the surface of the adsorbent particles. Hussain et al., Meena et al. and Seki et al. [38,40,41] noted similar observations and they suggested that the increase in temperature increase the rate of diffusion of the adsorbate molecules across the external boundary layer and in the internal pores of the adsorbent particle. This is due to the total volume and the possibility of the adsorbent pores, an increase of number of active sites for the adsorption as well as an increase in the mobility of the adsorbate molecules. However, the dye uptake was found to decrease from 69.5% to 54.1% with temperature increase from 35˚C to 65˚C suggesting that adsorption between the alga and the dye was mainly physical adsorption, dominant at lower temperature. Various authors [42,43] reported that the dye adsorption decreases with the increase of solution temperature. This can be explained by the weakening of bonds between dye molecules and active sites of adsorbents for high tem- peratures. Moreover the rise in temperature may damage the active binding sites in the biomass. 3.7. Scanning Electron Microscopy Scanning electron microscopy of biosorbent particles is represented in Figure 9. It shows a high surface porosity with numerous macropores and mesopores. These pores exhibit hole-like with rough surfaces suggesting that the examined biosorbent can tolerate superior dye adsorption Figure 8. Dye removal percentage by dried algal biomass at different temperature. (a) (b) Figure 9. Scanning electron micrographs of non-living algal particles. Copyright © 2013 SciRes. AJPS
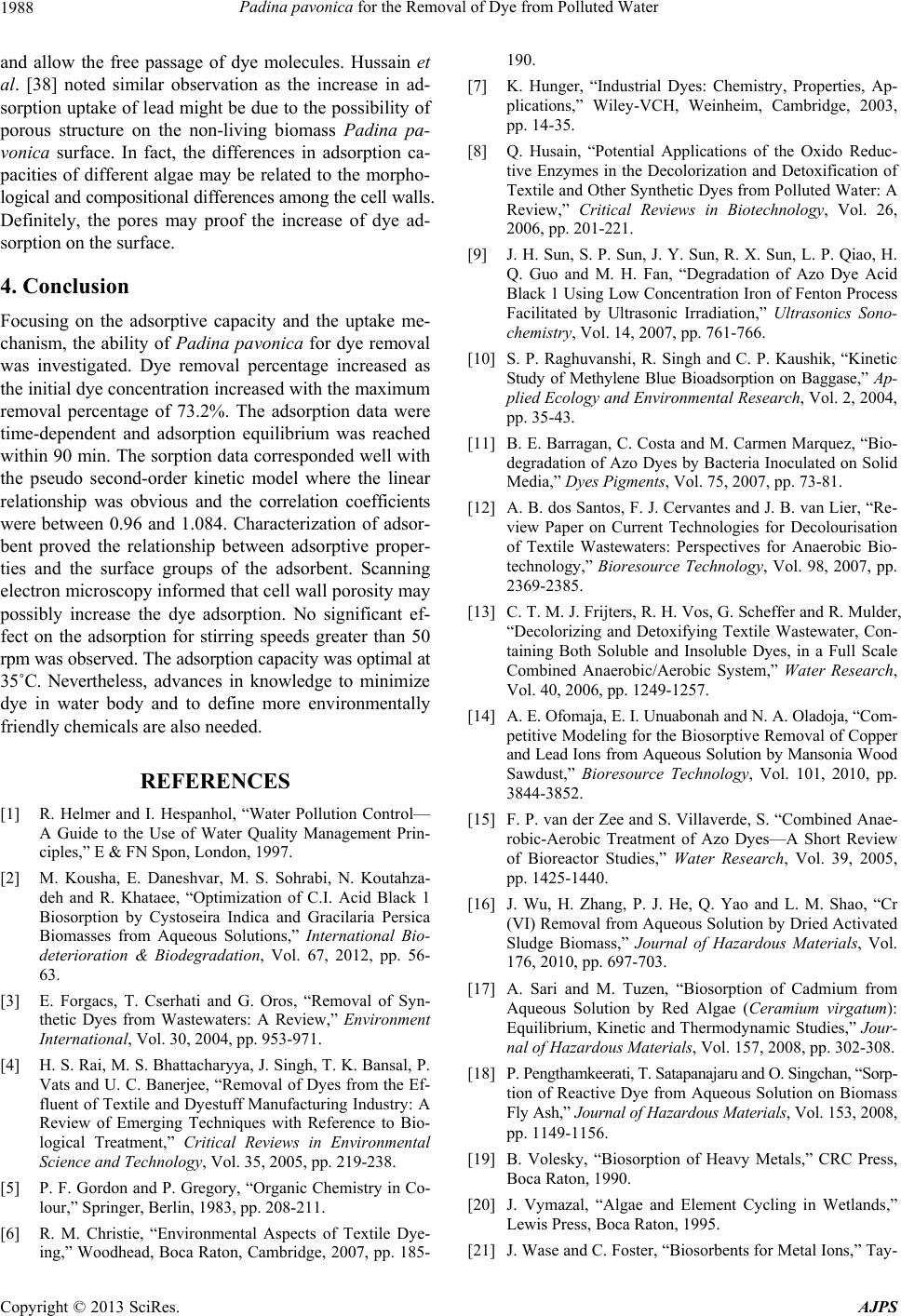 Padina pavonica for the Removal of Dye from Polluted Water 1988 and allow the free passage of dye molecules. Hussain et al. [38] noted similar observation as the increase in ad- sorption uptake of lead might be due to the possibility of porous structure on the non-living biomass Padina pa- vonica surface. In fact, the differences in adsorption ca- pacities of different algae may be related to the morpho- logical and compositional differences among the cell walls. Definitely, the pores may proof the increase of dye ad- sorption on the surface. 4. Conclusion Focusing on the adsorptive capacity and the uptake me- chanism, the ability of Padina pavonica for dye removal was investigated. Dye removal percentage increased as the initial dye concentration increased with the maximum removal percentage of 73.2%. The adsorption data were time-dependent and adsorption equilibrium was reached within 90 min. The sorption data corresponded well with the pseudo second-order kinetic model where the linear relationship was obvious and the correlation coefficients were between 0.96 and 1.084. Characterization of adsor- bent proved the relationship between adsorptive proper- ties and the surface groups of the adsorbent. Scanning electron microscopy informed that cell wall porosity may possibly increase the dye adsorption. No significant ef- fect on the adsorption for stirring speeds greater than 50 rpm was observed. The adsorption capacity was optimal at 35˚C. Nevertheless, advances in knowledge to minimize dye in water body and to define more environmentally friendly chemicals are also needed. REFERENCES [1] R. Helmer and I. Hespanhol, “Water Pollution Control— A Guide to the Use of Water Quality Management Prin- ciples,” E & FN Spon, London, 1997. [2] M. Kousha, E. Daneshvar, M. S. Sohrabi, N. Koutahza- deh and R. Khataee, “Optimization of C.I. Acid Black 1 Biosorption by Cystoseira Indica and Gracilaria Persica Biomasses from Aqueous Solutions,” International Bio- deterioration & Biodegradation, Vol. 67, 2012, pp. 56- 63. [3] E. Forgacs, T. Cserhati and G. Oros, “Removal of Syn- thetic Dyes from Wastewaters: A Review,” Environment International, Vol. 30, 2004, pp. 953-971. [4] H. S. Rai, M. S. Bhattacharyya, J. Singh, T. K. Bansal, P. Vats and U. C. Banerjee, “Removal of Dyes from the Ef- fluent of Textile and Dyestuff Manufacturing Industry: A Review of Emerging Techniques with Reference to Bio- logical Treatment,” Critical Reviews in Environmental Science and Technology, Vol. 35, 2005, pp. 219-238. [5] P. F. Gordon and P. Gregory, “Organic Chemistry in Co- lour,” Springer, Berlin, 1983, pp. 208-211. [6] R. M. Christie, “Environmental Aspects of Textile Dye- ing,” Woodhead, Boca Raton, Cambridge, 2007, pp. 185- 190. [7] K. Hunger, “Industrial Dyes: Chemistry, Properties, Ap- plications,” Wiley-VCH, Weinheim, Cambridge, 2003, pp. 14-35. [8] Q. Husain, “Potential Applications of the Oxido Reduc- tive Enzymes in the Decolorization and Detoxification of Textile and Other Synthetic Dyes from Polluted Water: A Review,” Critical Reviews in Biotechnology, Vol. 26, 2006, pp. 201-221. [9] J. H. Sun, S. P. Sun, J. Y. Sun, R. X. Sun, L. P. Qiao, H. Q. Guo and M. H. Fan, “Degradation of Azo Dye Acid Black 1 Using Low Concentration Iron of Fenton Process Facilitated by Ultrasonic Irradiation,” Ultrasonics Sono- chemistry, Vol. 14, 2007, pp. 761-766. [10] S. P. Raghuvanshi, R. Singh and C. P. Kaushik, “Kinetic Study of Methylene Blue Bioadsorption on Baggase,” Ap- plied Ecology and Environmental Research, Vol. 2, 2004, pp. 35-43. [11] B. E. Barragan, C. Costa and M. Carmen Marquez, “Bio- degradation of Azo Dyes by Bacteria Inoculated on Solid Media,” Dyes Pigments, Vol. 75, 2007, pp. 73-81. [12] A. B. dos Santos, F. J. Cervantes and J. B. van Lier, “Re- view Paper on Current Technologies for Decolourisation of Textile Wastewaters: Perspectives for Anaerobic Bio- technology,” Bioresource Technology, Vol. 98, 2007, pp. 2369-2385. [13] C. T. M. J. Frijters, R. H. Vos, G. Scheffer and R. Mulder, “Decolorizing and Detoxifying Textile Wastewater, Con- taining Both Soluble and Insoluble Dyes, in a Full Scale Combined Anaerobic/Aerobic System,” Water Research, Vol. 40, 2006, pp. 1249-1257. [14] A. E. Ofomaja, E. I. Unuabonah and N. A. Oladoja, “Com- petitive Modeling for the Biosorptive Removal of Copper and Lead Ions from Aqueous Solution by Mansonia Wood Sawdust,” Bioresource Technology, Vol. 101, 2010, pp. 3844-3852. [15] F. P. van der Zee and S. Villaverde, S. “Combined Anae- robic-Aerobic Treatment of Azo Dyes—A Short Review of Bioreactor Studies,” Water Research, Vol. 39, 2005, pp. 1425-1440. [16] J. Wu, H. Zhang, P. J. He, Q. Yao and L. M. Shao, “Cr (VI) Removal from Aqueous Solution by Dried Activated Sludge Biomass,” Journal of Hazardous Materials, Vol. 176, 2010, pp. 697-703. [17] A. Sari and M. Tuzen, “Biosorption of Cadmium from Aqueous Solution by Red Algae (Ceramium virgatum): Equilibrium, Kinetic and Thermodynamic Studies,” Jour- nal of Hazardous Materials, Vol. 157, 2008, pp. 302-308. [18] P. Pengthamkeerati, T. Satapanajaru and O. Singchan, “Sorp- tion of Reactive Dye from Aqueous Solution on Biomass Fly Ash,” Journal of Hazardous Materials, Vol. 153, 2008, pp. 1149-1156. [19] B. Volesky, “Biosorption of Heavy Metals,” CRC Press, Boca Raton, 1990. [20] J. Vymazal, “Algae and Element Cycling in Wetlands,” Lewis Press, Boca Raton, 1995. [21] J. Wase and C. Foster, “Biosorbents for Metal Ions,” Tay- Copyright © 2013 SciRes. AJPS
 Padina pavonica for the Removal of Dye from Polluted Water Copyright © 2013 SciRes. AJPS 1989 lor & Francis, 1996. [22] P. X. Sheng, Y. P. Ting, J. P. Chen and L. Hong, “Sorp- tion of Lead, Copper, Cadmium, Zinc, and Nickel by Ma- rine Algal Biomass: Characterization of Biosorptive Ca- pacity and Investigation of Mechanisms,” Journal of Col- loid and Interface Science, Vol. 275, 2004, pp. 131-141. [23] J. Wang and C. Chen, “Biosorbents for Heavy Metals Re- moval and Their Future,” Biotechnology Advances, Vol. 27, 2009, pp. 195-226. [24] R. H. S. Vieira and B. Volesky, “Biosorption: A Solution to Pollution,” International Microbiology, Vol. 3, 2000, pp. 17-24. [25] T. A. Davis, B. Volesky and A. Mucci, “A Review of the Biochemistry of Heavy Metal Biosorption by Brown Al- gae,” Water Research, Vol. 37, 2003, pp. 4311-4330. [26] A. Çelekli and H. Bozkurt, “Bio-Sorption of Cadmium and Nickel Ions Using Spirulina Platensis: Kinetic and Equilibrium Studies,” Desalination, Vol. 275, 2011, pp. 141-147. [27] A. Çelekli and F. Geyik, “Artificial Neural Networks (ANN) Approach for Modeling of Removal of Lanaset Red G on Chara contraria,” Bioresource Technology, Vol. 102, 2011, pp. 5634-5638. [28] L. Fang, C. Zhou, P. Cai, W. Chen, X. Rong, K. Dai, W. Liang, J. Gu and Q. Huang, “Binding Characteristics of Copper and Cadmium by Cyanobacterium Spirulina Pla- tensis,” Journal of Hazardous Materials, Vol. 190, 2011, pp. 810-815. [29] A. Srinivasan and T. Viraraghavan, “Decolorization of Dye Wastewaters by Biosorbents: A Review,” Journal of En- vironmental Management, Vol. 91, 2010, pp. 1915-1929. [30] S. Lagergren, “Zur Theorie der Sogenannten Adsorption Gelöster Stoffe.Kungliga Svenska Vetenskapsakademiens,” Handlingar Band, 1898. [31] Y. S. Ho and G. McKay, “Pseudo-Second Order Model for Sorption Processes,” Process Biochemistry, Vol. 34, No. 5, 1999, pp. 451-465. http://dx.doi.org/10.1016/S0032-9592(98)00112-5 [32] S. Cengiz and L. Cavas, “Removal of Methylene Blue by Invasive Marine Seaweed: Caulerpa Racemosa var. Cyl- indracea,” Bioresource Technology, Vol. 99, 2008, pp. 2357-2363. [33] M. T. Uddin, M. S. Islam and M. Z. Abedin, “Adsorption of Phenol from Aqueous Solution by Water Hyacinth Ash,” Journal of Engineering and Applied Sciences, Vol. 2, No. 2, 2007, pp. 11-17. [34] M. Dŏgan, H. Abak and M. Alkan, “Adsorption of Me- thylene Blue onto Hazelnut Shell: Kinetics, Mechanism and Activation Parameters,” Journal of Hazardous Mate- rials, Vol. 164, 2009, pp. 172-181. [35] P. Kaewsarn and O. Yu, “Cadmium (II) Removal from Aqueous Solutions by Pre-Treated Biomass of Marine Al- ga Padina sp.,” Environmental Pollution, Vol. 112, 2001, pp. 209-213. [36] O. Hamdaoui, “Batch Study of Liquid-Phase Adsorption of Methylene Blue Using Cedar Sawdust and Crushed Brick,” Journal of Hazardous Materials B, Vol. 135, 2006, pp. 264-273. [37] V. Murphy, H. Hughes and P. McLoughlin, “Cu (II) Bin- ding by Dried Biomass of Red, Green and Brown Macro- algae,” Water Research, Vol. 41, 2007, pp. 731-740. [38] M. A. Hussain, A. Salleh and P. Milow, “Characterization of the Adsorption of the Lead (II) by the Nonliving Bio- mass Spirogyra neglecta (Hasall) Kützing,” American Journal of Biochemistry and Biotechnology, Vol. 5, No. 2, 2009, pp. 75-83. http://dx.doi.org/10.3844/ajbbsp.2009.75.83 [39] W. M. Antunes, A. S. Luna, C. A. Henriques and A. C. A. Da Costa, “An Evaluation of Copper Biosorption by a Brown Seaweed under Optimized Conditions,” Electronic Journal of Biotechnology, Vol. 6 No. 3, 2003, pp. 174- 184. [40] A. K. Meena, G. K. Mishra, P. K. Rai, C. Rajgopal and P. N. Nagar, “Removal of Heavy Metal Ions from Aqueous Solutions Using Carbon Aerogel as an Adsorbent,” Jour- nal of Hazardous Materials, Vol. 122, 2005, pp. 161-170. [41] H. Seki, A. Suzuki and S. I. Mitsueda, “Adsorption of Heavy Metal Ions on Rhodobacter Sphaeroides and Alca- ligenes Eutrophus H16,” Journal of Colloid and Interface Science, Vol. 197, 1998, pp. 185-190. [42] J. M. Chern and C. Y. Wu, “Desorption of Dye from Ac- tivated Carbon Beds: Effects of Temperature, pH, and Alcohol,” Water Research, Vol. 35, 2001, pp. 4159-4165. [43] M. S. Chiou and H. Y. Li, “Equilibrium and Kinetic Mod- eling of Adsorption of Reactive Dye on Cross-Linked Chitosan Beads,” Journal of Hazardous Materials B, Vol. 93, 2002, pp. 233-248.
|