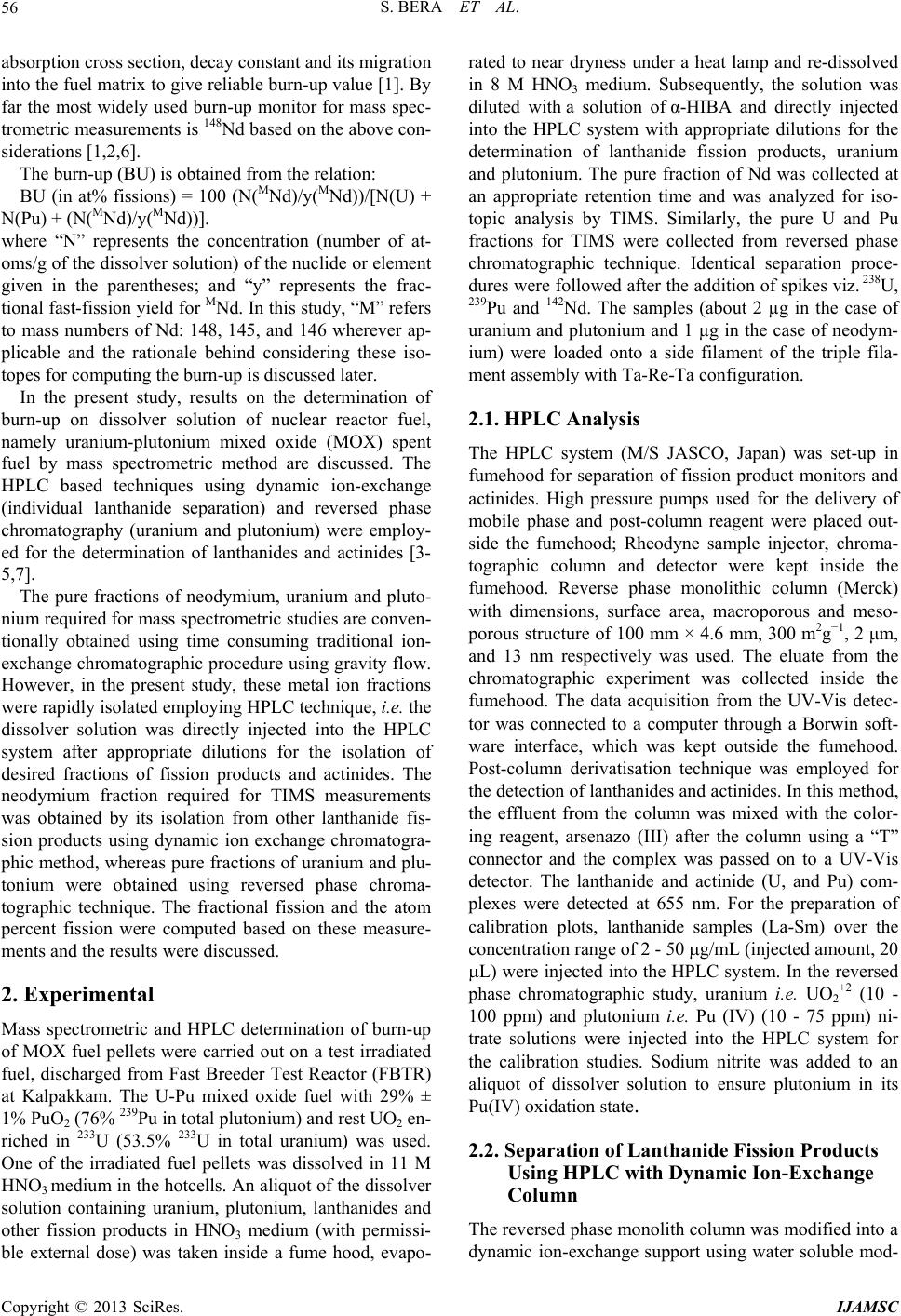
S. BERA ET AL.
56
absorption cross section, decay constant and its migration
into the fuel matrix to give reliable burn-up value [1]. By
far the most widely used burn-up monitor for mass spec-
trometric measurements is 148Nd based on the above con-
siderations [1,2,6].
The burn-up (BU) is obtained from the relation:
BU (in at% fissions) = 100 (N(MNd)/y(MNd))/[N(U) +
N(Pu) + (N(MNd)/y(MNd))].
where “N” represents the concentration (number of at-
oms/g of the dissolver solution) of the nuclide or element
given in the parentheses; and “y” represents the frac-
tional fast-fission yield for MNd. In this study, “M” refers
to mass numbers of Nd: 148, 145, and 146 wherever ap-
plicable and the rationale behind considering these iso-
topes for computing the burn-up is discussed later.
In the present study, results on the determination of
burn-up on dissolver solution of nuclear reactor fuel,
namely uranium-plutonium mixed oxide (MOX) spent
fuel by mass spectrometric method are discussed. The
HPLC based techniques using dynamic ion-exchange
(individual lanthanide separation) and reversed phase
chromatography (uranium and plutonium) were employ-
ed for the determination of lanthanides and actinides [3-
5,7].
The pure fractions of neodymium, uranium and pluto-
nium required for mass spectrometric studies are conven-
tionally obtained using time consuming traditional ion-
exchange chromatographic procedure using gravity flow.
However, in the present study, these metal ion fractions
were rapidly isolated employing HPLC technique, i.e. the
dissolver solution was directly injected into the HPLC
system after appropriate dilutions for the isolation of
desired fractions of fission products and actinides. The
neodymium fraction required for TIMS measurements
was obtained by its isolation from other lanthanide fis-
sion products using dynamic ion exchange chromatogra-
phic method, whereas pure fractions of uranium and plu-
tonium were obtained using reversed phase chroma-
tographic technique. The fractional fission and the atom
percent fission were computed based on these measure-
ments and the results were discussed.
2. Experimental
Mass spectrometric and HPLC determination of burn-up
of MOX fuel pellets were carried out on a test irradiated
fuel, discharged from Fast Breeder Test Reactor (FBTR)
at Kalpakkam. The U-Pu mixed oxide fuel with 29% ±
1% PuO2 (76% 239Pu in total plutonium) and rest UO2 en-
riched in 233U (53.5% 233U in total uranium) was used.
One of the irradiated fuel pellets was dissolved in 11 M
HNO3 medi u m in the hotcells. An aliquot of the dissolver
solution containing uranium, plutonium, lanthanides and
other fission products in HNO3 medium (with permissi-
ble external dose) was taken inside a fume hood, evapo-
rated to near dryness under a heat lamp and re-dissolved
in 8 M HNO3 medium. Subsequently, the solution was
diluted with a solution of α-HIBA and directly injected
into the HPLC system with appropriate dilutions for the
determination of lanthanide fission products, uranium
and plutonium. The pure fraction of Nd was collected at
an appropriate retention time and was analyzed for iso-
topic analysis by TIMS. Similarly, the pure U and Pu
fractions for TIMS were collected from reversed phase
chromatographic technique. Identical separation proce-
dures were followed after the addition of spikes viz. 238U,
239Pu and 142Nd. The samples (about 2 µg in the case of
uranium and plutonium and 1 µg in the case of neodym-
ium) were loaded onto a side filament of the triple fila-
ment assembly with Ta-Re-Ta configuration.
2.1. HPLC Analysis
The HPLC system (M/S JASCO, Japan) was set-up in
fumehood for separation of fission product monitors and
actinides. High pressure pumps used for the delivery of
mobile phase and post-column reagent were placed out-
side the fumehood; Rheodyne sample injector, chroma-
tographic column and detector were kept inside the
fumehood. Reverse phase monolithic column (Merck)
with dimensions, surface area, macroporous and meso-
porous structure of 100 mm × 4.6 mm, 300 m2g−1, 2 μm,
and 13 nm respectively was used. The eluate from the
chromatographic experiment was collected inside the
fumehood. The data acquisition from the UV-Vis detec-
tor was connected to a computer through a Borwin soft-
ware interface, which was kept outside the fumehood.
Post-column derivatisation technique was employed for
the detection of lanthanides and actinides. In this method,
the effluent from the column was mixed with the color-
ing reagent, arsenazo (III) after the column using a “T”
connector and the complex was passed on to a UV-Vis
detector. The lanthanide and actinide (U, and Pu) com-
plexes were detected at 655 nm. For the preparation of
calibration plots, lanthanide samples (La-Sm) over the
concentration range of 2 - 50 g/mL (injected amount, 20
L) were injected into the HPLC system. In the reversed
phase chromatographic study, uranium i.e. UO2+2 (10 -
100 ppm) and plutonium i.e. Pu (IV) (10 - 75 ppm) ni-
trate solutions were injected into the HPLC system for
the calibration studies. Sodium nitrite was added to an
aliquot of dissolver solution to ensure plutonium in its
Pu(IV) oxidation state.
2.2. Separation of Lanthanide Fission Products
Using HPLC with Dynamic Ion-Exchange
Column
The reversed phase monolith column was modified into a
dynamic ion-exchange support using water soluble mod-
Copyright © 2013 SciRes. IJAMSC