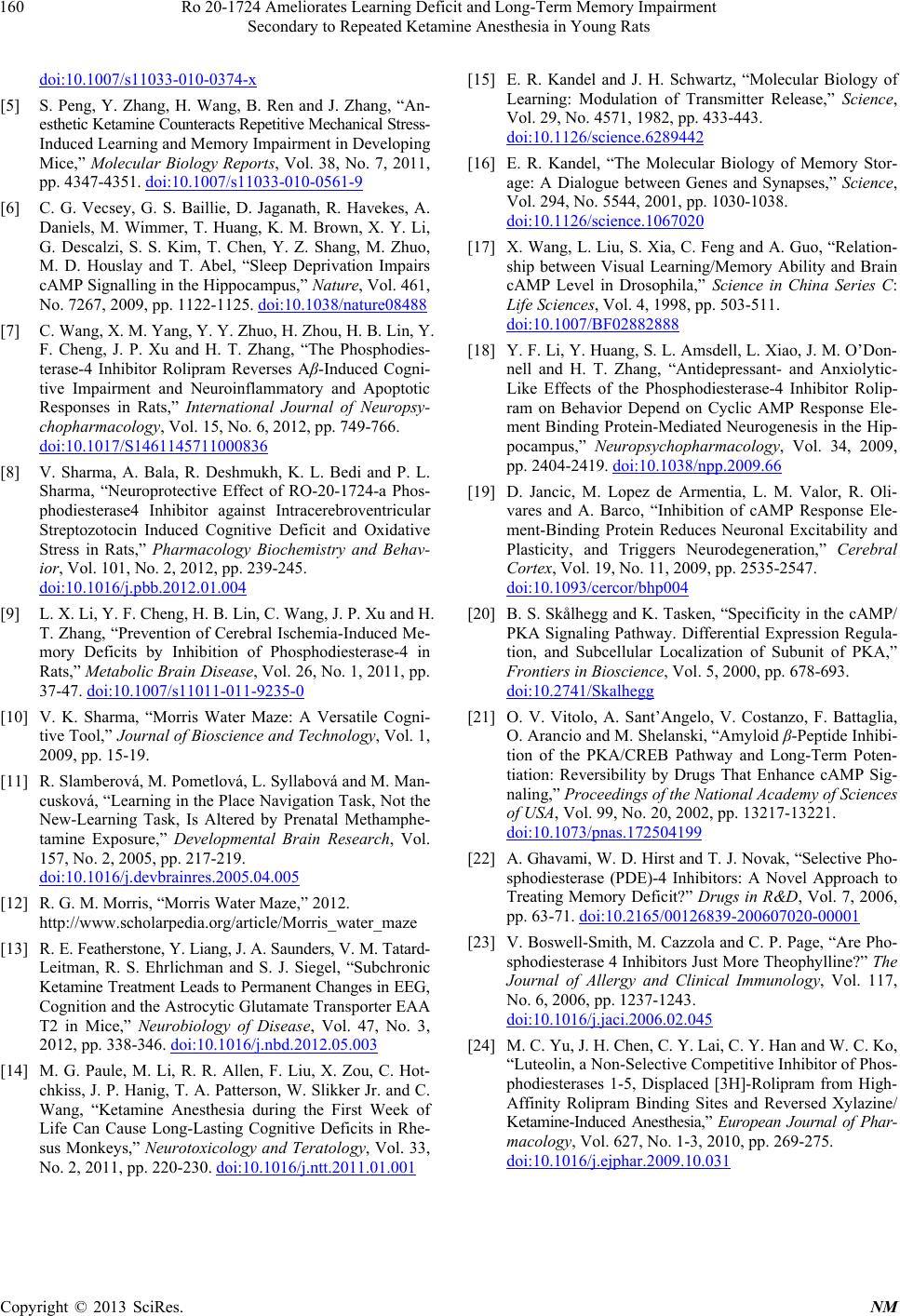
Ro 20-1724 Ameliorates Learning Deficit and Long-Term Memory Impairment
Secondary to Repeated Ketamine Anesthesia in Young Rats
Copyright © 2013 SciRes. NM
160
doi:10.1007/s11033-010-0374-x
[5] S. Peng, Y. Zhang, H. Wang, B. Ren and J. Zhang, “An-
esthetic Ketamine Counteracts Repetitive Mechanical Stress-
Induced Learning and Memory Impairment in Developing
Mice,” Molecular Biology Reports, Vol. 38, No. 7, 2011,
pp. 4347-4351. doi:10.1007/s11033-010-0561-9
[6] C. G. Vecsey, G. S. Baillie, D. Jaganath, R. Havekes, A.
Daniels, M. Wimmer, T. Huang, K. M. Brown, X. Y. Li,
G. Descalzi, S. S. Kim, T. Chen, Y. Z. Shang, M. Zhuo,
M. D. Houslay and T. Abel, “Sleep Deprivation Impairs
cAMP Signalling in the Hippocampus,” Nature, Vol. 461,
No. 7267, 2009, pp. 1122-1125. doi:10.1038/nature08488
[7] C. Wang, X. M. Yang, Y. Y. Zhuo, H. Zhou, H. B. Lin, Y.
F. Cheng, J. P. Xu and H. T. Zhang, “The Phosphodies-
terase-4 Inhibitor Rolipram Reverses Aβ-Induced Cogni-
tive Impairment and Neuroinflammatory and Apoptotic
Responses in Rats,” International Journal of Neuropsy-
chopharmacology, Vol. 15, No. 6, 2012, pp. 749-766.
doi:10.1017/S1461145711000836
[8] V. Sharma, A. Bala, R. Deshmukh, K. L. Bedi and P. L.
Sharma, “Neuroprotective Effect of RO-20-1724-a Phos-
phodiesterase4 Inhibitor against Intracerebroventricular
Streptozotocin Induced Cognitive Deficit and Oxidative
Stress in Rats,” Pharmacology Biochemistry and Behav-
ior, Vol. 101, No. 2, 2012, pp. 239-245.
doi:10.1016/j.pbb.2012.01.004
[9] L. X. Li, Y. F. Cheng, H. B. Lin, C. Wang, J. P. Xu and H.
T. Zhang, “Prevention of Cerebral Ischemia-Induced Me-
mory Deficits by Inhibition of Phosphodiesterase-4 in
Rats,” Metabolic Brain Disease, Vol. 26, No. 1, 2011, pp.
37-47. doi:10.1007/s11011-011-9235-0
[10] V. K. Sharma, “Morris Water Maze: A Versatile Cogni-
tive Tool,” Journal of Bioscience and Technology, Vol. 1,
2009, pp. 15-19.
[11] R. Slamberová, M. Pometlová, L. Syllabová and M. Man-
cusková, “Learning in the Place Navigation Task, Not the
New-Learning Task, Is Altered by Prenatal Methamphe-
tamine Exposure,” Developmental Brain Research, Vol.
157, No. 2, 2005, pp. 217-219.
doi:10.1016/j.devbrainres.2005.04.005
[12] R. G. M. Morris, “Morris Water Maze,” 2012.
http://www.scholarpedia.org/article/Morris_water_maze
[13] R. E. Featherstone, Y. Liang, J. A. Saunders, V. M. Tatard-
Leitman, R. S. Ehrlichman and S. J. Siegel, “Subchronic
Ketamine Treatment Leads to Permanent Changes in EEG,
Cognition and the Astrocytic Glutamate Transporter EAA
T2 in Mice,” Neurobiology of Disease, Vol. 47, No. 3,
2012, pp. 338-346. doi:10.1016/j.nbd.2012.05.003
[14] M. G. Paule, M. Li, R. R. Allen, F. Liu, X. Zou, C. Hot-
chkiss, J. P. Hanig, T. A. Patterson, W. Slikker Jr. and C.
Wang, “Ketamine Anesthesia during the First Week of
Life Can Cause Long-Lasting Cognitive Deficits in Rhe-
sus Monkeys,” Neurotoxicology and Teratology, Vol. 33,
No. 2, 2011, pp. 220-230. doi:10.1016/j.ntt.2011.01.001
[15] E. R. Kandel and J. H. Schwartz, “Molecular Biology of
Learning: Modulation of Transmitter Release,” Science,
Vol. 29, No. 4571, 1982, pp. 433-443.
doi:10.1126/science.6289442
[16] E. R. Kandel, “The Molecular Biology of Memory Stor-
age: A Dialogue between Genes and Synapses,” Science,
Vol. 294, No. 5544, 2001, pp. 1030-1038.
doi:10.1126/science.1067020
[17] X. Wang, L. Liu, S. Xia, C. Feng and A. Guo, “Relation-
ship between Visual Learning/Memory Ability and Brain
cAMP Level in Drosophila,” Science in China Series C:
Life Sciences, Vol. 4, 1998, pp. 503-511.
doi:10.1007/BF02882888
[18] Y. F. Li, Y. Huang, S. L. Amsdell, L. Xiao, J. M. O’Don-
nell and H. T. Zhang, “Antidepressant- and Anxiolytic-
Like Effects of the Phosphodiesterase-4 Inhibitor Rolip-
ram on Behavior Depend on Cyclic AMP Response Ele-
ment Binding Protein-Mediated Neurogenesis in the Hip-
pocampus,” Neuropsychopharmacology, Vol. 34, 2009,
pp. 2404-2419. doi:10.1038/npp.2009.66
[19] D. Jancic, M. Lopez de Armentia, L. M. Valor, R. Oli-
vares and A. Barco, “Inhibition of cAMP Response Ele-
ment-Binding Protein Reduces Neuronal Excitability and
Plasticity, and Triggers Neurodegeneration,” Cerebral
Cortex, Vol. 19, No. 11, 2009, pp. 2535-2547.
doi:10.1093/cercor/bhp004
[20] B. S. Skålhegg and K. Tasken, “Specificity in the cAMP/
PKA Signaling Pathway. Differential Expression Regula-
tion, and Subcellular Localization of Subunit of PKA,”
Frontiers in Bioscience, Vol. 5, 2000, pp. 678-693.
doi:10.2741/Skalhegg
[21] O. V. Vitolo, A. Sant’Angelo, V. Costanzo, F. Battaglia,
O. Arancio and M. Shelanski, “Amyloid β-Peptide Inhibi-
tion of the PKA/CREB Pathway and Long-Term Poten-
tiation: Reversibility by Drugs That Enhance cAMP Sig-
naling,” Proceedings of the National Academy of Sciences
of USA, Vol. 99, No. 20, 2002, pp. 13217-13221.
doi:10.1073/pnas.172504199
[22] A. Ghavami, W. D. Hirst and T. J. Novak, “Selective Pho-
sphodiesterase (PDE)-4 Inhibitors: A Novel Approach to
Treating Memory Deficit?” Drugs in R&D, Vol. 7, 2006,
pp. 63-71. doi:10.2165/00126839-200607020-00001
[23] V. Boswell-Smith, M. Cazzola and C. P. Page, “Are Pho-
sphodiesterase 4 Inhibitors Just More Theophylline?” The
Journal of Allergy and Clinical Immunology, Vol. 117,
No. 6, 2006, pp. 1237-1243.
doi:10.1016/j.jaci.2006.02.045
[24] M. C. Yu, J. H. Chen, C. Y. Lai, C. Y. Han and W. C. Ko,
“Luteolin, a Non-Selective Competitive Inhibitor of Phos-
phodiesterases 1-5, Displaced [3H]-Rolipram from High-
Affinity Rolipram Binding Sites and Reversed Xylazine/
Ketamine-Induced Anesthesia,” European Journal of Phar-
macology, Vol. 627, No. 1-3, 2010, pp. 269-275.
doi:10.1016/j.ejphar.2009.10.031