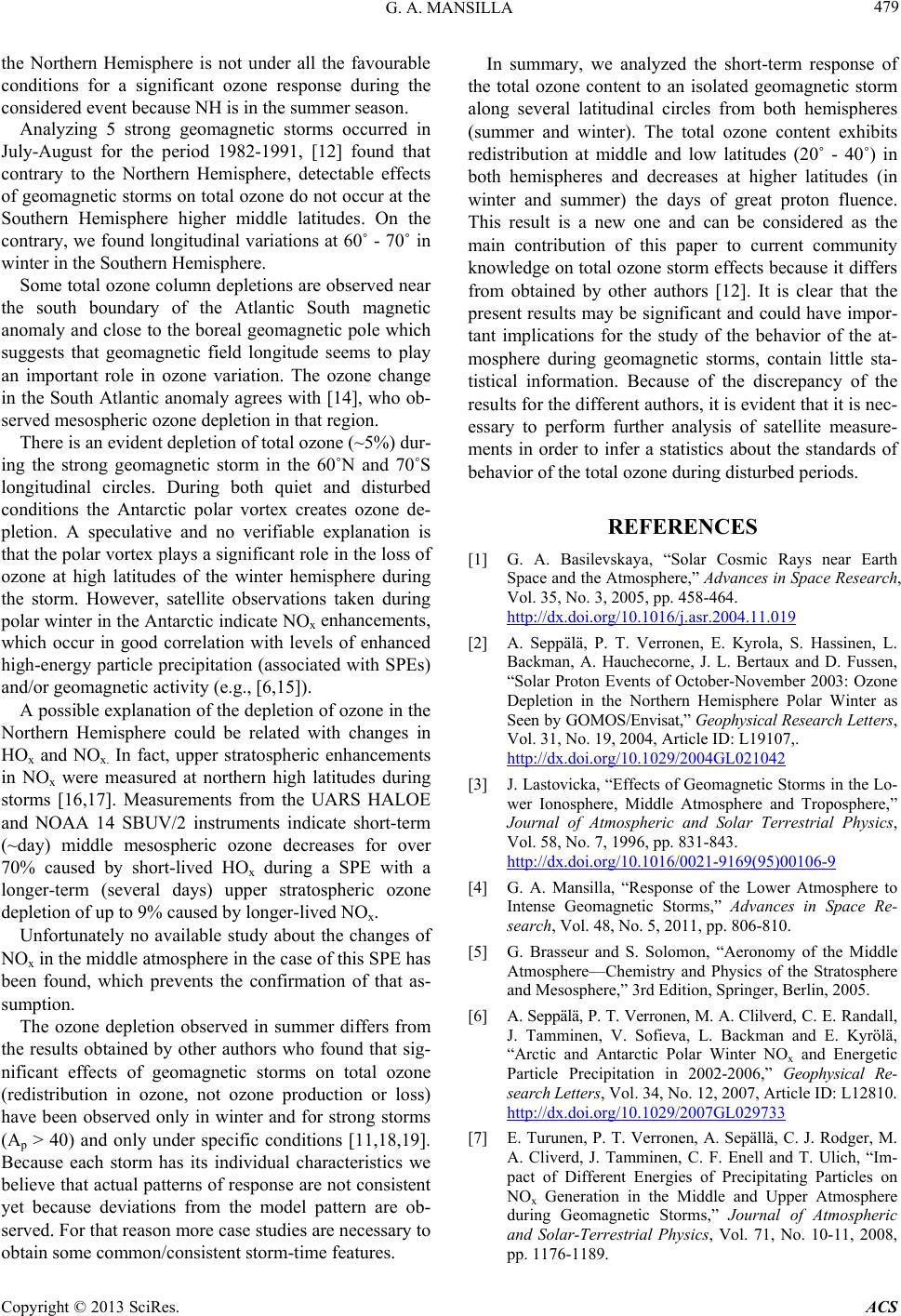
G. A. MANSILLA 479
the Northern Hemisphere is not under all the favourable
conditions for a significant ozone response during the
considered event because NH is in the summer season.
Analyzing 5 strong geomagnetic storms occurred in
July-August for the period 1982-1991, [12] found that
contrary to the Northern Hemisphere, detectable effects
of geomagnetic storms on total ozone do not occur at the
Southern Hemisphere higher middle latitudes. On the
contrary, we found longitudinal variations at 60˚ - 70˚ in
winter in the Southern Hemisphere.
Some total ozone column depletions are observed near
the south boundary of the Atlantic South magnetic
anomaly and close to the boreal geomagnetic pole which
suggests that geomagnetic field longitude seems to play
an important role in ozone variation. The ozone change
in the South Atlantic anomaly agrees with [14], who ob-
served mesospheric ozone depletion in that region.
There is an evident depletion of total ozone (~5%) dur-
ing the strong geomagnetic storm in the 60˚N and 70˚S
longitudinal circles. During both quiet and disturbed
conditions the Antarctic polar vortex creates ozone de-
pletion. A speculative and no verifiable explanation is
that the polar vortex plays a significant role in the loss of
ozone at high latitudes of the winter hemisphere during
the storm. However, satellite observations taken during
polar winter in the Antarctic indicate NOx enhancements,
which occur in good correlation with levels of enhanced
high-energy particle precipitation (associated with SPEs)
and/or geomagnetic activity (e.g., [6,15]).
A possible explanation of the depletion of ozone in the
Northern Hemisphere could be related with changes in
HOx and NOx. In fact, upper stratospheric enhancements
in NOx were measured at northern high latitudes during
storms [16,17]. Measurements from the UARS HALOE
and NOAA 14 SBUV/2 instruments indicate short-term
(~day) middle mesospheric ozone decreases for over
70% caused by short-lived HOx during a SPE with a
longer-term (several days) upper stratospheric ozone
depletion of up to 9% caused by longer-lived NOx.
Unfortunately no available study about the changes of
NOx in the middle atmosphere in the case of this SPE has
been found, which prevents the confirmation of that as-
sumption.
The ozone depletion observed in summer differs from
the results obtained by other authors who found that sig-
nificant effects of geomagnetic storms on total ozone
(redistribution in ozone, not ozone production or loss)
have been observed only in winter and for strong storms
(Ap > 40) and only under specific conditions [11,18,19].
Because each storm has its individual characteristics we
believe that actual patterns of response are not consistent
yet because deviations from the model pattern are ob-
served. For that reason more case studies are necessary to
obtain some common/consistent storm-time features.
In summary, we analyzed the short-term response of
the total ozone content to an isolated geomagnetic storm
along several latitudinal circles from both hemispheres
(summer and winter). The total ozone content exhibits
redistribution at middle and low latitudes (20˚ - 40˚) in
both hemispheres and decreases at higher latitudes (in
winter and summer) the days of great proton fluence.
This result is a new one and can be considered as the
main contribution of this paper to current community
knowledge on total ozone storm effects because it differs
from obtained by other authors [12]. It is clear that the
present results may be significant and could have impor-
tant implications for the study of the behavior of the at-
mosphere during geomagnetic storms, contain little sta-
tistical information. Because of the discrepancy of the
results for the different authors, it is evident that it is nec-
essary to perform further analysis of satellite measure-
ments in order to infer a statistics about the standards of
behavior of the total ozone during disturbed periods.
REFERENCES
[1] G. A. Basilevskaya, “Solar Cosmic Rays near Earth
Space and the Atmosphere,” Advances in Space Research,
Vol. 35, No. 3, 2005, pp. 458-464.
http://dx.doi.org/10.1016/j.asr.2004.11.019
[2] A. Seppälä, P. T. Verronen, E. Kyrola, S. Hassinen, L.
Backman, A. Hauchecorne, J. L. Bertaux and D. Fussen,
“Solar Proton Events of October-November 2003: Ozone
Depletion in the Northern Hemisphere Polar Winter as
Seen by GOMOS/Envisat,” Geophysical Research Letters,
Vol. 31, No. 19, 2004, Article ID: L19107,.
http://dx.doi.org/10.1029/2004GL021042
[3] J. Lastovicka, “Effects of Geomagnetic Storms in the Lo-
wer Ionosphere, Middle Atmosphere and Troposphere,”
Journal of Atmospheric and Solar Terrestrial Physics,
Vol. 58, No. 7, 1996, pp. 831-843.
http://dx.doi.org/10.1016/0021-9169(95)00106-9
[4] G. A. Mansilla, “Response of the Lower Atmosphere to
Intense Geomagnetic Storms,” Advances in Space Re-
search, Vol. 48, No. 5, 2011, pp. 806-810.
[5] G. Brasseur and S. Solomon, “Aeronomy of the Middle
Atmosphere—Chemistry and Physics of the Stratosphere
and Mesosphere,” 3rd Edition, Springer, Berlin, 2005.
[6] A. Seppälä, P. T. Verronen, M. A. Clilverd, C. E. Randall,
J. Tamminen, V. Sofieva, L. Backman and E. Kyrölä,
“Arctic and Antarctic Polar Winter NOx and Energetic
Particle Precipitation in 2002-2006,” Geophysical Re-
search Letters, Vol. 34, No. 12, 2007, Article ID: L12810.
http://dx.doi.org/10.1029/2007GL029733
[7] E. Turunen, P. T. Verronen, A. Sepällä, C. J. Rodger, M.
A. Cliverd, J. Tamminen, C. F. Enell and T. Ulich, “Im-
pact of Different Energies of Precipitating Particles on
NOx Generation in the Middle and Upper Atmosphere
during Geomagnetic Storms,” Journal of Atmospheric
and Solar-Terrestrial Physics, Vol. 71, No. 10-11, 2008,
pp. 1176-1189.
Copyright © 2013 SciRes. ACS