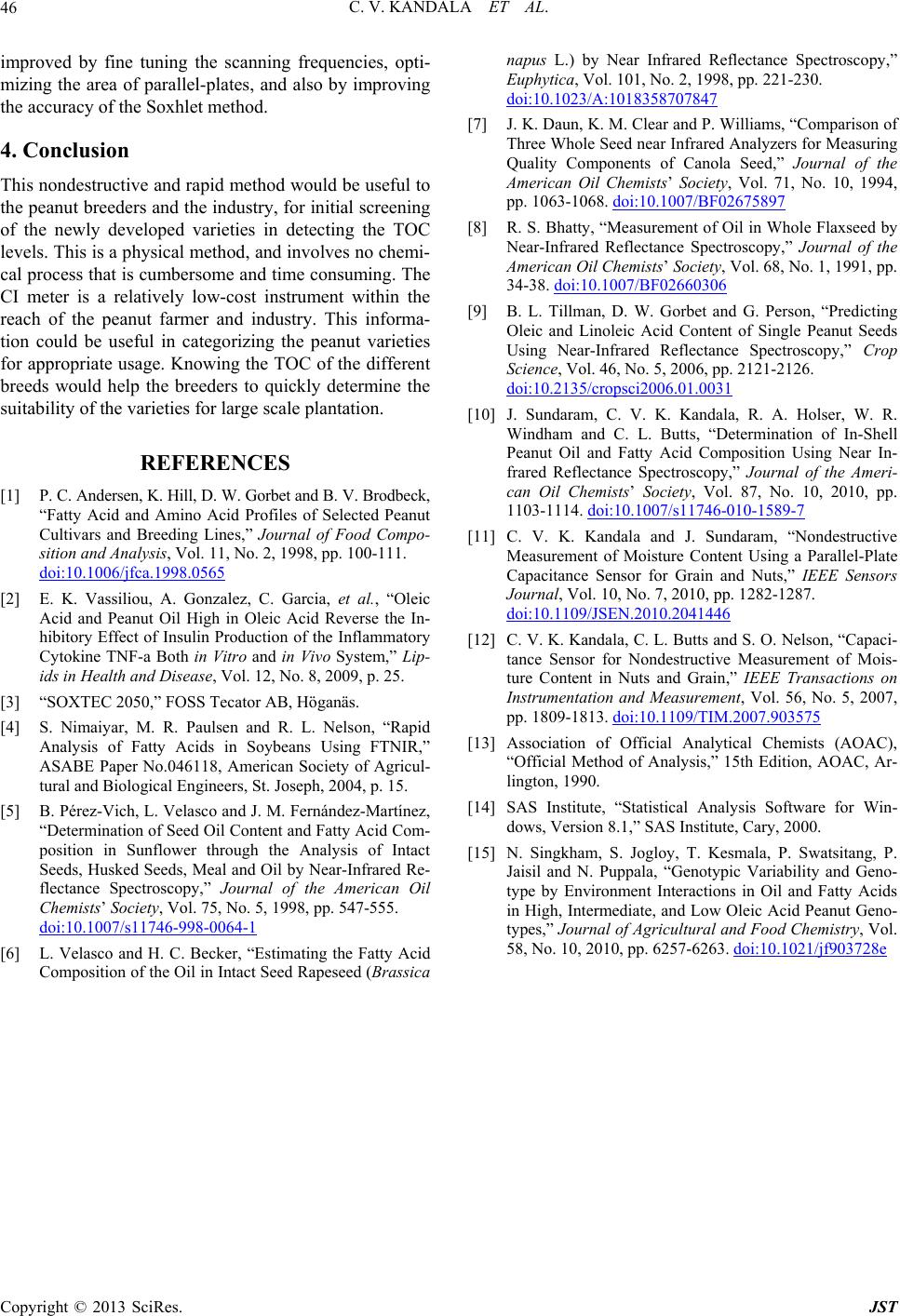
C. V. KANDALA ET AL.
Copyright © 2013 SciRes. JST
46
improved by fine tuning the scanning frequencies, opti-
mizing the area of parallel-plates, and also by improving
the accuracy of the Soxhlet method.
4. Conclusion
This nondestructive and rapid method would be useful to
the peanut breeders and the industry, for initial screening
of the newly developed varieties in detecting the TOC
levels. This is a physical method, and involves no chemi-
cal process that is cumbersome and time consuming. The
CI meter is a relatively low-cost instrument within the
reach of the peanut farmer and industry. This informa-
tion could be useful in categorizing the peanut varieties
for appropriate usage. Knowing the TOC of the different
breeds would help the breeders to quickly determine the
suitability of the varieties for large scale plantation.
REFERENCES
[1] P. C. Andersen, K. Hill, D. W. Gorbet and B. V. Brodbeck,
“Fatty Acid and Amino Acid Profiles of Selected Peanut
Cultivars and Breeding Lines,” Journal of Food Compo-
sition and Analysis, Vol. 11, No. 2, 1998, pp. 100-111.
doi:10.1006/jfca.1998.0565
[2] E. K. Vassiliou, A. Gonzalez, C. Garcia, et al., “Oleic
Acid and Peanut Oil High in Oleic Acid Reverse the In-
hibitory Effect of Insulin Production of the Inflammatory
Cytokine TNF-a Both in Vitro and in Vivo System,” Lip-
ids in Health and Disease, Vol. 12, No. 8, 2009, p. 25.
[3] “SOXTEC 2050,” FOSS Tecator AB, Höganäs.
[4] S. Nimaiyar, M. R. Paulsen and R. L. Nelson, “Rapid
Analysis of Fatty Acids in Soybeans Using FTNIR,”
ASABE Paper No.046118, American Society of Agricul-
tural and Biological Engineers, St. Joseph, 2004, p. 15.
[5] B. Pérez-Vich, L. Velasco and J. M. Fernández-Martínez,
“Determination of Seed Oil Content and Fatty Acid Com-
position in Sunflower through the Analysis of Intact
Seeds, Husked Seeds, Meal and Oil by Near-Infrared Re-
flectance Spectroscopy,” Journal of the American Oil
Chemists’ Society, Vol. 75, No. 5, 1998, pp. 547-555.
doi:10.1007/s11746-998-0064-1
[6] L. Velasco and H. C. Becker, “Estimating the Fatty Acid
Composition of the Oil in Intact Seed Rapeseed (Brassica
napus L.) by Near Infrared Reflectance Spectroscopy,”
Euphytica, Vol. 101, No. 2, 1998, pp. 221-230.
doi:10.1023/A:1018358707847
[7] J. K. Daun, K. M. Clear and P. Williams, “Comparison of
Three Whole Seed near Infrared Analyzers for Measuring
Quality Components of Canola Seed,” Journal of the
American Oil Chemists’ Society, Vol. 71, No. 10, 1994,
pp. 1063-1068. doi:10.1007/BF02675897
[8] R. S. Bhatty, “Measurement of Oil in Whole Flaxseed by
Near-Infrared Reflectance Spectroscopy,” Journal of the
American Oil Chemists’ Society, Vol. 68, No. 1, 1991, pp.
34-38. doi:10.1007/BF02660306
[9] B. L. Tillman, D. W. Gorbet and G. Person, “Predicting
Oleic and Linoleic Acid Content of Single Peanut Seeds
Using Near-Infrared Reflectance Spectroscopy,” Crop
Science, Vol. 46, No. 5, 2006, pp. 2121-2126.
doi:10.2135/cropsci2006.01.0031
[10] J. Sundaram, C. V. K. Kandala, R. A. Holser, W. R.
Windham and C. L. Butts, “Determination of In-Shell
Peanut Oil and Fatty Acid Composition Using Near In-
frared Reflectance Spectroscopy,” Journal of the Ameri-
can Oil Chemists’ Society, Vol. 87, No. 10, 2010, pp.
1103-1114. doi:10.1007/s11746-010-1589-7
[11] C. V. K. Kandala and J. Sundaram, “Nondestructive
Measurement of Moisture Content Using a Parallel-Plate
Capacitance Sensor for Grain and Nuts,” IEEE Sensors
Journal, Vol. 10, No. 7, 2010, pp. 1282-1287.
doi:10.1109/JSEN.2010.2041446
[12] C. V. K. Kandala, C. L. Butts and S. O. Nelson, “Capaci-
tance Sensor for Nondestructive Measurement of Mois-
ture Content in Nuts and Grain,” IEEE Transactions on
Instrumentation and Measurement, Vol. 56, No. 5, 2007,
pp. 1809-1813. doi:10.1109/TIM.2007.903575
[13] Association of Official Analytical Chemists (AOAC),
“Official Method of Analysis,” 15th Edition, AOAC, Ar-
lington, 1990.
[14] SAS Institute, “Statistical Analysis Software for Win-
dows, Version 8.1,” SAS Institute, Cary, 2000.
[15] N. Singkham, S. Jogloy, T. Kesmala, P. Swatsitang, P.
Jaisil and N. Puppala, “Genotypic Variability and Geno-
type by Environment Interactions in Oil and Fatty Acids
in High, Intermediate, and Low Oleic Acid Peanut Geno-
types,” Journal of Agricultural and Food Chemistry, Vol.
58, No. 10, 2010, pp. 6257-6263. doi:10.1021/jf903728e