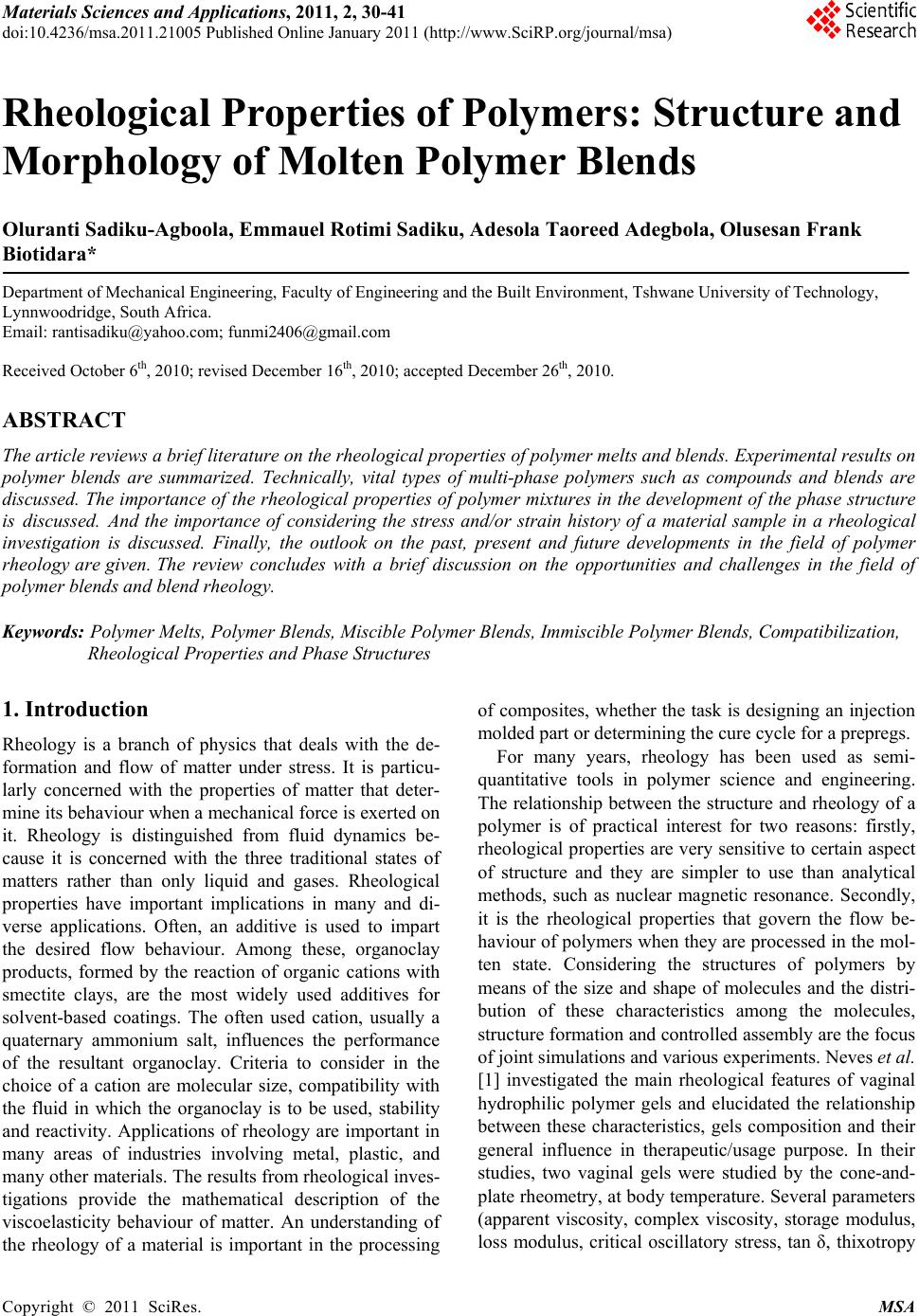 Materials Sciences and Applicatio ns, 2011, 2, 30-41 doi:10.4236/msa.2011.21005 Published Online January 2011 (http://www.SciRP.org/journal/msa) Copyright © 2011 SciRes. MSA Rheological Properties of Polymers: Structure and Morphology of Molten Polymer Blends Oluranti Sadiku-Agboola, Emmauel Rotimi Sadiku, Adesola Taoreed Adegbola, Olusesan Frank Biotidara* Department of Mechanical Engineering, Faculty of Engineering and the Built Environment, Tshwane University of Technology, Lynnwoodridge, South Africa. Email: rantisadiku@yahoo.com; funmi2406@gmail.com Received October 6th, 2010; revised December 16th, 2010; accepted December 26th, 2010. ABSTRACT The article reviews a brief literature on the rheological properties of polymer melts and blends. Experimental results on polymer blends are summarized. Technically, vital types of multi-phase polymers such as compounds and blends are discussed. The importance of the rheological properties of polymer mixtures in the development of the phase structure is discussed. And the importance of considering the stress and/or strain history of a material sample in a rheological investigation is discussed. Finally, the outlook on the past, present and future developments in the field of polymer rheology are given. The review concludes with a brief discussion on the opportunities and challenges in the field of polymer blends and blend rheology. Keywords: Polymer Melts, Polymer Blends, Miscible Polymer Blends, Immiscible Polymer Blends, Compatibilization, Rheological Properties and Phase Structures 1. Introduction Rheology is a branch of physics that deals with the de- formation and flow of matter under stress. It is particu- larly concerned with the properties of matter that deter- mine its behaviour when a mechanical force is exerted on it. Rheology is distinguished from fluid dynamics be- cause it is concerned with the three traditional states of matters rather than only liquid and gases. Rheological properties have important implications in many and di- verse applications. Often, an additive is used to impart the desired flow behaviour. Among these, organoclay products, formed by the reaction of organic cations with smectite clays, are the most widely used additives for solvent-based coatings. The often used cation, usually a quaternary ammonium salt, influences the performance of the resultant organoclay. Criteria to consider in the choice of a cation are molecular size, compatibility with the fluid in which the organoclay is to be used, stability and reactivity. Applications of rheology are important in many areas of industries involving metal, plastic, and many other materials. The results from rheological inves- tigations provide the mathematical description of the viscoelasticity behaviour of matter. An understanding of the rheology of a material is important in the processing of composites, whether the task is designing an injection molded part or determining the cure cycle for a prepregs. For many years, rheology has been used as semi- quantitative tools in polymer science and engineering. The relationship between the structure and rheology of a polymer is of practical interest for two reasons: firstly, rheological properties are very sensitive to certain aspect of structure and they are simpler to use than analytical methods, such as nuclear magnetic resonance. Secondly, it is the rheological properties that govern the flow be- haviour of polymers when they are processed in the mol- ten state. Considering the structures of polymers by means of the size and shape of molecules and the distri- bution of these characteristics among the molecules, structure formation and controlled assembly are the focus of joint simulations and various experiments. Neves et al. [1] investigated the main rheological features of vaginal hydrophilic polymer gels and elucidated the relationship between these characteristics, gels composition and their general influence in therapeutic/usage purpose. In their studies, two vaginal gels were studied by the cone-and- plate rheometry, at body temperature. Several parameters (apparent viscosity, complex viscosity, storage modulus, loss modulus, critical oscillatory stress, tan δ, thixotropy
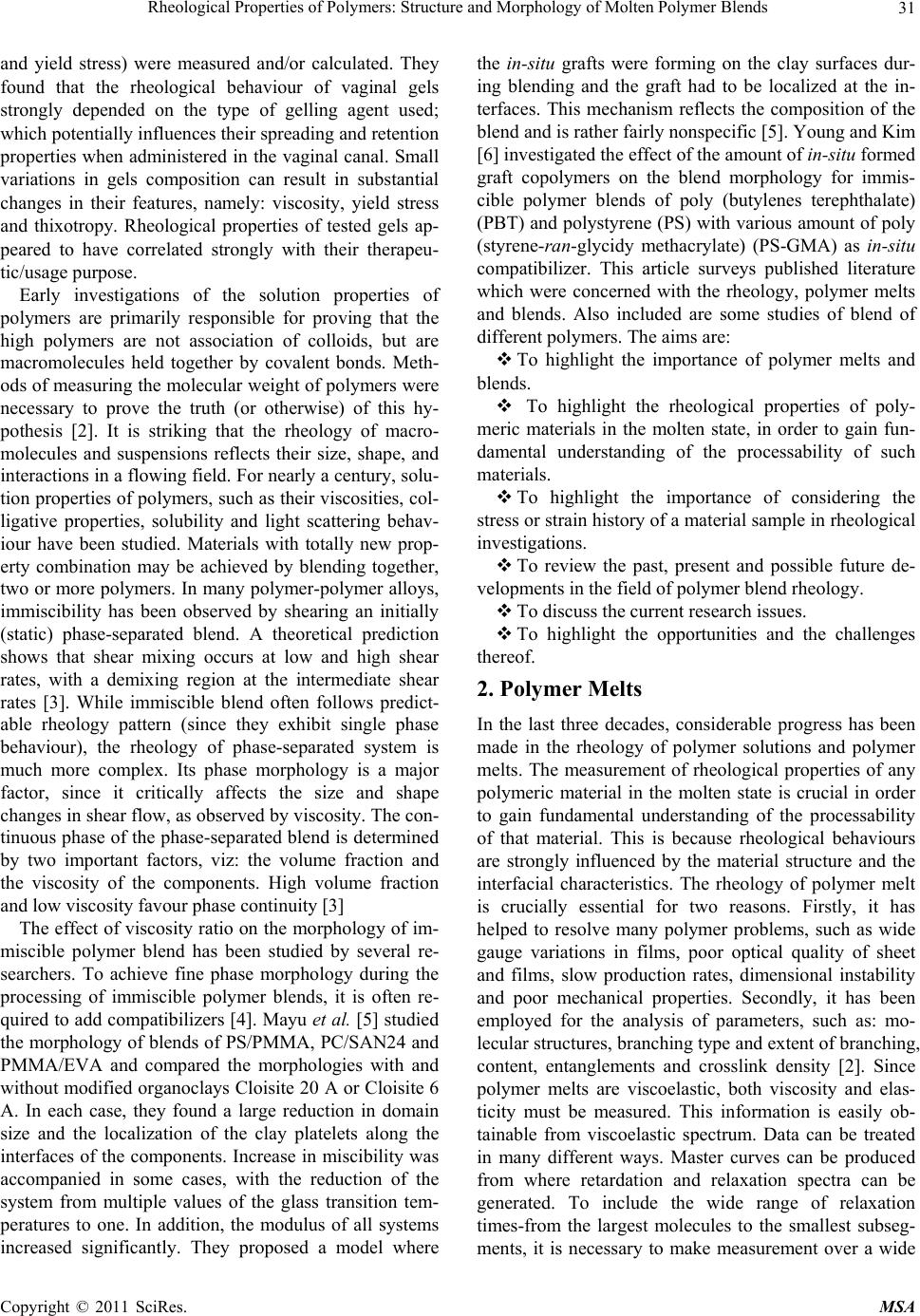 Rheological Properties of Polymers: Structure and Morphology of Molten Polymer Blends31 and yield stress) were measured and/or calculated. They found that the rheological behaviour of vaginal gels strongly depended on the type of gelling agent used; which potentially influences their spreading and retention properties when administered in the vaginal canal. Small variations in gels composition can result in substantial changes in their features, namely: viscosity, yield stress and thixotropy. Rheological properties of tested gels ap- peared to have correlated strongly with their therapeu- tic/usage purpose. Early investigations of the solution properties of polymers are primarily responsible for proving that the high polymers are not association of colloids, but are macromolecules held together by covalent bonds. Meth- ods of measuring the molecular weight of polymers were necessary to prove the truth (or otherwise) of this hy- pothesis [2]. It is striking that the rheology of macro- molecules and suspensions reflects their size, shape, and interactions in a flowing field. For nearly a century, solu- tion properties of polymers, such as their viscosities, col- ligative properties, solubility and light scattering behav- iour have been studied. Materials with totally new prop- erty combination may be achieved by blending together, two or more polymers. In many polymer-polymer alloys, immiscibility has been observed by shearing an initially (static) phase-separated blend. A theoretical prediction shows that shear mixing occurs at low and high shear rates, with a demixing region at the intermediate shear rates [3]. While immiscible blend often follows predict- able rheology pattern (since they exhibit single phase behaviour), the rheology of phase-separated system is much more complex. Its phase morphology is a major factor, since it critically affects the size and shape changes in shear flow, as observed by viscosity. The con- tinuous phase of the phase-separated blend is determined by two important factors, viz: the volume fraction and the viscosity of the components. High volume fraction and low viscosity favour phase continuity [3] The effect of viscosity ratio on the morphology of im- miscible polymer blend has been studied by several re- searchers. To achieve fine phase morphology during the processing of immiscible polymer blends, it is often re- quired to add compatibilizers [4]. Mayu et al. [5] studied the morphology of blends of PS/PMMA, PC/SAN24 and PMMA/EVA and compared the morphologies with and without modified organoclays Cloisite 20 A or Cloisite 6 A. In each case, they found a large reduction in domain size and the localization of the clay platelets along the interfaces of the components. Increase in miscibility was accompanied in some cases, with the reduction of the system from multiple values of the glass transition tem- peratures to one. In addition, the modulus of all systems increased significantly. They proposed a model where the in-situ grafts were forming on the clay surfaces dur- ing blending and the graft had to be localized at the in- terfaces. This mechanism reflects the composition of the blend and is rather fairly nonspecific [5]. Young and Kim [6] investigated the effect of the amount of in-situ formed graft copolymers on the blend morphology for immis- cible polymer blends of poly (butylenes terephthalate) (PBT) and polystyrene (PS) with various amount of poly (styrene-ran-glycidy methacrylate) (PS-GMA) as in-situ compatibilizer. This article surveys published literature which were concerned with the rheology, polymer melts and blends. Also included are some studies of blend of different polymers. The aims are: To highlight the importance of polymer melts and blends. To highlight the rheological properties of poly- meric materials in the molten state, in order to gain fun- damental understanding of the processability of such materials. To highlight the importance of considering the stress or strain history of a material sample in rheological investigations. To review the past, present and possible future de- velopments in the field of polymer blend rheology. To discuss the current research issues. To highlight the opportunities and the challenges thereof. 2. Polymer Melts In the last three decades, considerable progress has been made in the rheology of polymer solutions and polymer melts. The measurement of rheological properties of any polymeric material in the molten state is crucial in order to gain fundamental understanding of the processability of that material. This is because rheological behaviours are strongly influenced by the material structure and the interfacial characteristics. The rheology of polymer melt is crucially essential for two reasons. Firstly, it has helped to resolve many polymer problems, such as wide gauge variations in films, poor optical quality of sheet and films, slow production rates, dimensional instability and poor mechanical properties. Secondly, it has been employed for the analysis of parameters, such as: mo- lecular structures, branching type and extent of branching, content, entanglements and crosslink density [2]. Since polymer melts are viscoelastic, both viscosity and elas- ticity must be measured. This information is easily ob- tainable from viscoelastic spectrum. Data can be treated in many different ways. Master curves can be produced from where retardation and relaxation spectra can be generated. To include the wide range of relaxation times-from the largest molecules to the smallest subseg- ments, it is necessary to make measurement over a wide Copyright © 2011 SciRes. MSA
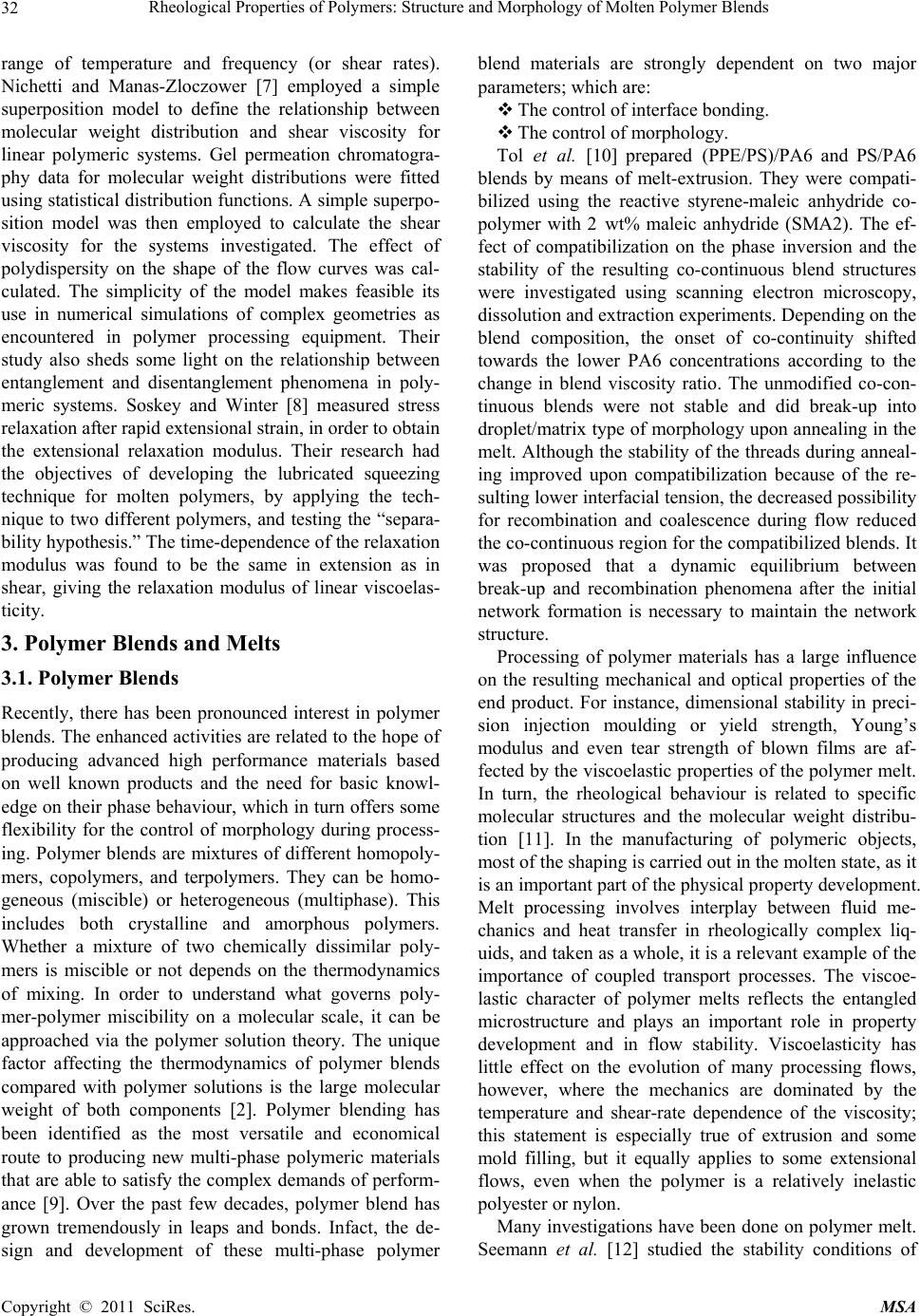 Rheological Properties of Polymers: Structure and Morphology of Molten Polymer Blends 32 range of temperature and frequency (or shear rates). Nichetti and Manas-Zloczower [7] employed a simple superposition model to define the relationship between molecular weight distribution and shear viscosity for linear polymeric systems. Gel permeation chromatogra- phy data for molecular weight distributions were fitted using statistical distribution functions. A simple superpo- sition model was then employed to calculate the shear viscosity for the systems investigated. The effect of polydispersity on the shape of the flow curves was cal- culated. The simplicity of the model makes feasible its use in numerical simulations of complex geometries as encountered in polymer processing equipment. Their study also sheds some light on the relationship between entanglement and disentanglement phenomena in poly- meric systems. Soskey and Winter [8] measured stress relaxation after rapid extensional strain, in order to obtain the extensional relaxation modulus. Their research had the objectives of developing the lubricated squeezing technique for molten polymers, by applying the tech- nique to two different polymers, and testing the “separa- bility hypothesis.” The time-dependence of the relaxation modulus was found to be the same in extension as in shear, giving the relaxation modulus of linear viscoelas- ticity. 3. Polymer Blends and Melts 3.1. Polymer Blends Recently, there has been pronounced interest in polymer blends. The enhanced activities are related to the hope of producing advanced high performance materials based on well known products and the need for basic knowl- edge on their phase behaviour, which in turn offers some flexibility for the control of morphology during process- ing. Polymer blends are mixtures of different homopoly- mers, copolymers, and terpolymers. They can be homo- geneous (miscible) or heterogeneous (multiphase). This includes both crystalline and amorphous polymers. Whether a mixture of two chemically dissimilar poly- mers is miscible or not depends on the thermodynamics of mixing. In order to understand what governs poly- mer-polymer miscibility on a molecular scale, it can be approached via the polymer solution theory. The unique factor affecting the thermodynamics of polymer blends compared with polymer solutions is the large molecular weight of both components [2]. Polymer blending has been identified as the most versatile and economical route to producing new multi-phase polymeric materials that are able to satisfy the complex demands of perform- ance [9]. Over the past few decades, polymer blend has grown tremendously in leaps and bonds. Infact, the de- sign and development of these multi-phase polymer blend materials are strongly dependent on two major parameters; which are: The control of interface bonding. The control of morphology. Tol et al. [10] prepared (PPE/PS)/PA6 and PS/PA6 blends by means of melt-extrusion. They were compati- bilized using the reactive styrene-maleic anhydride co- polymer with 2 wt% maleic anhydride (SMA2). The ef- fect of compatibilization on the phase inversion and the stability of the resulting co-continuous blend structures were investigated using scanning electron microscopy, dissolution and extraction experiments. Depending on the blend composition, the onset of co-continuity shifted towards the lower PA6 concentrations according to the change in blend viscosity ratio. The unmodified co-con- tinuous blends were not stable and did break-up into droplet/matrix type of morphology upon annealing in the melt. Although the stability of the threads during anneal- ing improved upon compatibilization because of the re- sulting lower interfacial tension, the decreased possibility for recombination and coalescence during flow reduced the co-continuous region for the compatibilized blends. It was proposed that a dynamic equilibrium between break-up and recombination phenomena after the initial network formation is necessary to maintain the network structure. Processing of polymer materials has a large influence on the resulting mechanical and optical properties of the end product. For instance, dimensional stability in preci- sion injection moulding or yield strength, Young’s modulus and even tear strength of blown films are af- fected by the viscoelastic properties of the polymer melt. In turn, the rheological behaviour is related to specific molecular structures and the molecular weight distribu- tion [11]. In the manufacturing of polymeric objects, most of the shaping is carried out in the molten state, as it is an important part of the physical property development. Melt processing involves interplay between fluid me- chanics and heat transfer in rheologically complex liq- uids, and taken as a whole, it is a relevant example of the importance of coupled transport processes. The viscoe- lastic character of polymer melts reflects the entangled microstructure and plays an important role in property development and in flow stability. Viscoelasticity has little effect on the evolution of many processing flows, however, where the mechanics are dominated by the temperature and shear-rate dependence of the viscosity; this statement is especially true of extrusion and some mold filling, but it equally applies to some extensional flows, even when the polymer is a relatively inelastic polyester or nylon. Many investigations have been done on polymer melt. Seemann et al. [12] studied the stability conditions of Copyright © 2011 SciRes. MSA
 Rheological Properties of Polymers: Structure and Morphology of Molten Polymer Blends33 thin (3 to 300 nm) liquid polymer films on various sub- strates. The key role was played by the effective interface potential, φ of the system air/film/substrate, which de- termined the de-wetting scenario, in case, if the film is not stable. In their study, they described how to distin- guish spinodal de-wetting scenario from heterogeneous and homogeneous de-wetting by analysing the emerging structures of the film surface; by e.g. Minkowski meas- ures. They also included line tension studies of tiny droplets, showing that the long-range part of φ does af- fect the drop profile, but only very close to the three phase boundary line. The dynamic properties of the films are characterized via various experimental methods: the form of the de-wetting front, for example, was recorded by scanning probe microscopy that gave insight into the boundary condition between the liquid and the substrate. They further reported experiments probing the viscosity and the glass transition temperature of nm-thick films using e.g. ellipsometry. They found that even short- chained polymer melts exhibit a significant reduction of the glass transition temperature as the film thickness is reduced below 100 nm. Daoulas et al. [13] presented an atomistic modeling approach for simulating the interface between a polymer melt and a crystalline solid substrate. As a test case, a thin film of polyethylene (PE) melt con- fined between a semi infinite graphite phase on the one side and vacuum on the other, were considered. The simulation was carried out in the isothermal-isobaric sta- tistical ensemble with an efficient Monte Carlo (MC) algorithm based on state of-the-art variable connectivity moves. The atomistic simulations are conducted by de- scribing the PE and PP chains with a united atom model, which considers each methylene (CH2) and methyl (CH3) group along the chain backbone as single interaction sites. To calculate the potential energy of interaction between polymer atoms and the semi-infinite graphite substrate, capable of incorporating the exact crystallographic struc- ture of graphite, the method designed by Steele was im- plemented. The new approach has allowed the authors to analyze the structural and conformational properties on the length scale of just a few angstroms from both sur- faces. Detailed results are presented for the local mass density, structure and conformation of PE at the two in- terfaces, obtained from simulations with model, strictly monodisperse PE samples of molecular length up to C-400. Additional structural features of the adsorbed layer, such as the distribution of skeletal carbon atoms in train, loop and tail conformations and their statistics, are also analyzed in detail and compared with the predictions of the lattice-based Scheutjens-Fleer self-consistent mean field theory in the limit of zero solvent concentration (melt case). Their atomistic simulation data demonstrated a stronger dependence of these descriptors of adsorbed layer structure on chain length than what was calculated by the mesoscopic Scheutjens-Fleer lattice model. In a second step, thoroughly equilibrated configurations of the confined model PE melt films are subjected to de- tailed molecular dynamics (MD) simulations in the iso- thermal-isobaric statistical ensemble to analyze their dy- namic behaviour. The MD simulations are carried out with the rRESPA method of multiple-time-step algorithm and have allowed them to monitor segmental and chain center-of-mass mean-square displacements over time scales on the order of a few hundreds of nanoseconds. Foteinopoulou et al. [14] subjected a large number of well- equilibrated atomistic configurations of linear, strictly monodisperse polyethylene (PE) melts of molecular length ranging from C-24 up to C-1000, obtained through extensive Monte Carlo simulations based on chain con- nectivity altering algorithms, to a detailed topological analysis. Primitive paths are geometrically constructed connecting the two ends of a polymer chain (which in all cases are considered as fixed in space) under the con- straint of no chain cross ability, such that the multiple disconnected (coarse-grained) path has minimum contour length. When applied to a given, dense polymer configu- ration in 3-D space, the algorithm returns the primitive path and the related number and positions of entangle- ments (kinks) for all chains in the simulation box, thus providing extremely useful information for the topologi- cal structure (the primitive path network) hidden in bulk PE. In particular, their analysis demonstrated that once a characteristic chain length value (around C-200) is ex- ceeded, the entanglement molecular length for PE at T 450 K reaches a plateau value, characteristic of the en- tangled polymeric behaviour). They further validated recent analytical predictions about the shape of the dis- tribution for the number of strands in a chain at equilib- rium. At the same time, they showed that the number of entanglements obtained by assuming random walk devi- ates significantly from these predictions, which they re- garded as clear evidence that by directly counting the entanglements and their distribution functions, as pro- posed in their work, offers advantages for a quantitative analysis of the statistical nature of entanglements in polymeric systems. 3.2. Vital Types of Multi-Phase Polymers: Compounds and Blends Over the years, the fact that polymer cannot mix with each other to form homogeneous mixture at segmental scale has been the driving force for a large number of studies on multiphase polymeric materials. It has been known for a long time that the physical properties of these phase-separated polymers; such as toughness, flowability, transparency and weather resistance, to men- Copyright © 2011 SciRes. MSA
 Rheological Properties of Polymers: Structure and Morphology of Molten Polymer Blends 34 tion a few, are dictated by their morphology [15]. As a consequence, a variety of chemical and physical methods has been extensively exploited to control morphology of immiscible polymer mixtures. Reactive polymers are widely utilized for various practical purposes such as the modification of surface tension between different phases via which morphology and physical properties of multi- phase polymeric material can be modified and controlled. These techniques are termed reactive compatibility [15]. Types of polymer blends are quite varied and comprise of many diverse combinations of polymeric materials for both academic and industrial interests. The primary dif- ferentiation of polymer blends involves their phase be- haviour; specifically miscibility versus phase-separation. The primary advantage of miscible versus phase-sepa- rated polymer blends is the blend property profile (me- chanical properties), which is generally intermediate be- tween that of the unblended constituents. The technology involves in polymer blends includes a multitude of polymer alloy compositions including elastomer blends, engineering polymer blends, impact modified polymer blends, etc. 3.3. Miscible Polymer Blends Miscible polymer blends are an expensive, easy-to-use approach for investigating and refining polymer combi- nations. It facilitates combinations that work and some that don’t. Miscible polymer blends behave similarly to what is expected of a single phase system. Their proper- ties are a combination of properties of pure components. The characteristics of components affecting the proper- ties of miscible blends are their chemical structure and molecular weight, their concentration and intermolecular interactions. While miscible blend system is of consid- erable scientific and practical interest, it should not be concluded that miscibility is always preferred with re- spect to properties. 3.4. Immiscible Polymer Blends Mixing immiscible polymers in the liquid state can result in various phase morphologies. These depend on the na- ture and the relative amounts of the polymers used and on the flow history of the sample. Immiscible polymers behave as different materials at different flow fields. The phase morphology is an important factor in the rheology of immiscible polymer blends. Most binary polymer blends are immiscible. To a large extend, the characteris- tics of these immiscible polymer blends are determined by the state of the interface between the components. The interaction of two melts at the interface is primarily rep- resentented by a quantity k, called the interfacial tension. “Interfacial tension is the excess free energy caused by the existence of an interface, arising from the unbalanced molecular forces [16]. Generally, chemically different polymers are immiscible and their blending leads to ma- terials with weak interfacial adhesion and thus poor me- chanical integrity. The conversion of immiscible blend to useful polymeric products with the desired properties requires some manipulations of the interface. One of the classical methods to ensure adhesion between the phases (reduction of the interfacial tension) is the use of a third component, a compatibilizer, which is miscible in both cases. According to Elias et al. [17], in recent years, new concept of compatibilization using rigid nano-materials like silica nano-particles has been proposed. 3.5. Compatibilization Compatibilization is the process of modification of inter- facial properties in immiscible polymer blends. This re- sults in the reduction of the interfacial tension, stabiliza- tion of the desired morphology and improved interaction between phases of solid state. In order words, compatibi- lization results in the formation of polymer alloys. Com- patibilization is accomplished by physical or chemical means. In the former case, the desired level of dispersion is generated by physical means, then physically stabilised (e.g., by quenching, retardation, cross-linking or co-cry- stallization). In the later case, the morphology does not only depend on the level of mechanical mixing, but to a great extent, it is controlled by the compatibilizer [18]. Table 1 below, shows the different type of polymer blends [3]. 4. Experimental Results on Polymer Blends Young and Kim [6] investigated the effect of the amount of in-situ prepared graft copolymers on the blend mor- Table 1. Types of polymer blends. TYPES OF POLYMER BLENDS 1. Engineering polymer blends 2. Impact modified polymer blends 3. Emulsion blends 4. Crystalline-crystalline polymer blends 5. Crystalline-amorphous polymer blends 6. Thermosetting polymer blends 7. Impact modified polymer blends 8. Molecular composites 9. Biodegradable polymer blends 10. Reactive compatibilized blends 11. Liquid-crystalline polymer blends 12. Polyolefin blends 13. Interpenetrating polymer networks 14. Polyelectrolyte complexes 15. Recycled polymer blends 16. Water soluble polymer blends 17. Polymer blend composites 18. Black copolymer-homopolymers blends 19. Core-shell polymer system 20. Elastomer blends Copyright © 2011 SciRes. MSA
 Rheological Properties of Polymers: Structure and Morphology of Molten Polymer Blends35 phology of immiscible polymer systems of poly (buty lenes terephthalate) (PBT) and polystyrene (PS) with- various amount of poly (styrene-ran-glycidy methacry- late) (PS-GMA) as in-situ compatibilizers. They used two different blending methods to prepare the blends: A melt blending (MB) and a solution blending followed by an oscillatory shearing in the molten state (SOM). The molecular weight of the in-situ PS-g-PBT copolymers formed from the reaction between PS-GMA and PBT in the blend was determined using high temperature gel permeation chromatography (GPC). The concentration of the in-situ formed graft copolymers of PS-g-PBT in the blends [copolymer blend] prepared either by MB or SOM was determined by solvent extraction and followed by Fourier Transform Infrared Spectroscopy (FTIR) analysis. On the basis of the GPC and FTIR results, they concluded that the PS-g-PBT in the blend has between 1.3-2 PBT chains grafted onto a PS-GMA chain. From the FTIR analysis and the morphology of the blend in- vestigated by scanning electron microscopy (SEM) and transmission electron microscopy (TEM), they found that the interfacial area density of the in-situ formed PS-g-PBT is ~0.1, chains/nm2, for the blends prepared by SOM, regardless of the amounts of PS-GMA added ini- tially in the blend. Block copolymers may be added as surface-active compatibilizers in order to control the morphology of blends of immiscible polymers. Velankar et al. [19] in- vestigated the effects of such added compatibilizers on the rheological properties of droplet-matrix blends are experimentally. They studied the model blends composed of polyisobutylene (PIB) droplets in a polydimethylsi- loxane (PDMS) matrix, compatibilized with a diblock copolymer of PIB and PDMS. The viscosity and the first normal stress difference under steady shear conditions, and complex moduli after cessation of shear are meas- ured. They found that addition of the compatibilizer slightly raises the magnitude of the terminal complex viscosity of blends at all ratios of viscosity. With addi- tion of the compatibilizer, the terminal relaxation time was found to increase sharply at high viscosity ratio, whereas the steady shear was found to increase at low viscosity ratios. Lu and Isacsson [20] studied the rheological properties of styrene-butadiene-styrene co- polymer (SBS) modified bitumen. The modified binders were prepared using a laboratory mixer. Five types of bitumen from four sources were mixed with two SBS polymers at different polymer contents. The modified binders were characterized using dynamic mechanical analysis over a wide range of temperatures and frequen- cies. It was found that the addition of the SBS polymers increased the binder electrical properties at high tem- peratures and improved the binder flexibility at low tem- peratures. The temperature susceptibility of bitumen was also reduced by SBS modification. However this prop- erty cannot be evaluated with a single-valued parameter. The degree of modification with respect to the binder rheology varied with temperature and frequency, and was dependent on the bitumen source/grade and the polymer concentration and structure. William and Wagner [21] demonstrated a new method that applies the Porod limit of small angle neutron scat- tering to measure the interface morphology in concen- trated, immiscible blends under simple shear. The effect of viscoelasticity contrast on rheology and blend micro- structure was probed for a model dispersion of liquid crystalline polymer dispersed in a linear polymer matrix. In comparison to the theories demonstrated by the com- plex morphological evolution, is a consequence of the unique rheology of liquid crystalline polymers. The re- sults at low shear rates were used to predict the onset of stable micro-fibrillation at higher shear rates through consideration of the contrast in the first normal stress differences. Kuo and Chang [22] prepared polymer blends of poly (vinylphenol) (PVPh) with poly (vi- nylpyrrolidone) (PVP) by solution casting from the N, N-dimethylformamide (DMF) solution. Differential scanning calorimeter (DSC), Fourier transforms infrared spectroscopy (FTIR) and solid state Nuclear Magnetic Resonance (NMR) were used to investigate the hydrogen bonding and miscibility behaviour of the blend. The PVPh/PVP blend system has a single glass transition temperature over the entire composition range, indicating that this blend is able to form a miscible phase due to the formation of inter-hydrogen bonding between the hy- droxyl of PVPh and the carbonyl of PVP. FTIR and solid-state NMR were further employed to study the hy- drogen-bonding interaction between the PVPh hydroxyl group and the PVP carbonyl group at various composi- tions. According to the Painter-Coleman association model (PCAM), the inter-association constant for the PVPh/PVP blend is significantly higher than the self- association constant of PVPh, revealing that the tendency towards hydrogen bonding of the PVPh and PVP domi- nates the intra-hydrogen bonding of the PVPh in the mixture. Kim et al. [23] examined block copolymers of PC-b-PMMA (polycarbonate-b-polymethylmethacrylate) and PC-b-SAN (polycarbonate-b-(styrene-c-acryloni- trile)), as compatibilizers for blends of PC with SAN copolymer. The average diameter of the dispersed particles was measured with an image analyzer, and the interfacial properties of the blends were analyzed with an imbedded fiber retraction (IFR) technique. The average diameter of dispersed particles and interfacial tension of the PC/SAN blends reached a minimum value when the SAN co- polymer contained about 24 wt% AN. Interfacial tension Copyright © 2011 SciRes. MSA
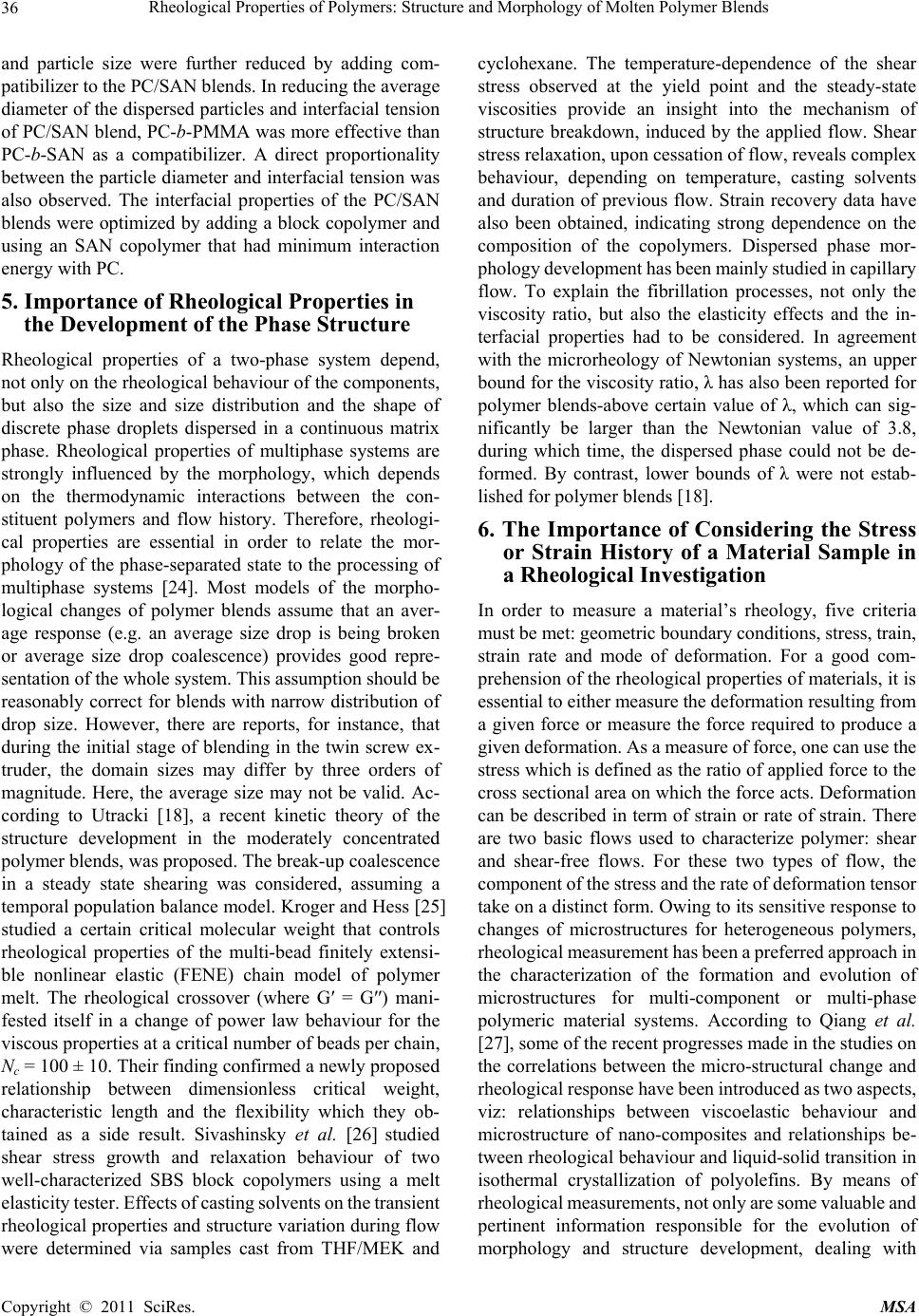 Rheological Properties of Polymers: Structure and Morphology of Molten Polymer Blends 36 and particle size were further reduced by adding com- patibilizer to the PC/SAN blends. In reducing the average diameter of the dispersed particles and interfacial tension of PC/SAN blend, PC-b-PMMA was more effective than PC-b-SAN as a compatibilizer. A direct proportionality between the particle diameter and interfacial tension was also observed. The interfacial properties of the PC/SAN blends were optimized by adding a block copolymer and using an SAN copolymer that had minimum interaction energy with PC. 5. Importance of Rheological Properties in the Development of the Phase Structure Rheological properties of a two-phase system depend, not only on the rheological behaviour of the components, but also the size and size distribution and the shape of discrete phase droplets dispersed in a continuous matrix phase. Rheological properties of multiphase systems are strongly influenced by the morphology, which depends on the thermodynamic interactions between the con- stituent polymers and flow history. Therefore, rheologi- cal properties are essential in order to relate the mor- phology of the phase-separated state to the processing of multiphase systems [24]. Most models of the morpho- logical changes of polymer blends assume that an aver- age response (e.g. an average size drop is being broken or average size drop coalescence) provides good repre- sentation of the whole system. This assumption should be reasonably correct for blends with narrow distribution of drop size. However, there are reports, for instance, that during the initial stage of blending in the twin screw ex- truder, the domain sizes may differ by three orders of magnitude. Here, the average size may not be valid. Ac- cording to Utracki [18], a recent kinetic theory of the structure development in the moderately concentrated polymer blends, was proposed. The break-up coalescence in a steady state shearing was considered, assuming a temporal population balance model. Kroger and Hess [25] studied a certain critical molecular weight that controls rheological properties of the multi-bead finitely extensi- ble nonlinear elastic (FENE) chain model of polymer melt. The rheological crossover (where G′ = G″) mani- fested itself in a change of power law behaviour for the viscous properties at a critical number of beads per chain, Nc = 100 ± 10. Their finding confirmed a newly proposed relationship between dimensionless critical weight, characteristic length and the flexibility which they ob- tained as a side result. Sivashinsky et al. [26] studied shear stress growth and relaxation behaviour of two well-characterized SBS block copolymers using a melt elasticity tester. Effects of casting solvents on the transient rheological properties and structure variation during flow were determined via samples cast from THF/MEK and cyclohexane. The temperature-dependence of the shear stress observed at the yield point and the steady-state viscosities provide an insight into the mechanism of structure breakdown, induced by the applied flow. Shear stress relaxation, upon cessation of flow, reveals complex behaviour, depending on temperature, casting solvents and duration of previous flow. Strain recovery data have also been obtained, indicating strong dependence on the composition of the copolymers. Dispersed phase mor- phology development has been mainly studied in capillary flow. To explain the fibrillation processes, not only the viscosity ratio, but also the elasticity effects and the in- terfacial properties had to be considered. In agreement with the microrheology of Newtonian systems, an upper bound for the viscosity ratio, λ has also been reported for polymer blends-above certain value of λ, which can sig- nificantly be larger than the Newtonian value of 3.8, during which time, the dispersed phase could not be de- formed. By contrast, lower bounds of λ were not estab- lished for polymer blends [18]. 6. The Importance of Considering the Stress or Strain History of a Material Sample in a Rheological Investigation In order to measure a material’s rheology, five criteria must be met: geometric boundary conditions, stress, train, strain rate and mode of deformation. For a good com- prehension of the rheological properties of materials, it is essential to either measure the deformation resulting from a given force or measure the force required to produce a given deformation. As a measure of force, one can use the stress which is defined as the ratio of applied force to the cross sectional area on which the force acts. Deformation can be described in term of strain or rate of strain. There are two basic flows used to characterize polymer: shear and shear-free flows. For these two types of flow, the component of the stress and the rate of deformation tensor take on a distinct form. Owing to its sensitive response to changes of microstructures for heterogeneous polymers, rheological measurement has been a preferred approach in the characterization of the formation and evolution of microstructures for multi-component or multi-phase polymeric material systems. According to Qiang et al. [27], some of the recent progresses made in the studies on the correlations between the micro-structural change and rheological response have been introduced as two aspects, viz: relationships between viscoelastic behaviour and microstructure of nano-composites and relationships be- tween rheological behaviour and liquid-solid transition in isothermal crystallization of polyolefins. By means of rheological measurements, not only are some valuable and pertinent information responsible for the evolution of morphology and structure development, dealing with Copyright © 2011 SciRes. MSA
 Rheological Properties of Polymers: Structure and Morphology of Molten Polymer Blends37 these polymer systems can be obtained, but also the cor- responding results are in favor of designing and preparing novel polymer-based composites and functional materials. Kohli et al. [28] assessed the effect of deformation his- tory on the morphology and properties of liquid crystal- line polymers (LCP) blended with polycarbonate resin. The addition of an immiscible LCP phase was found to improve the melt processability of the host thermoplastic polymer. In addition, by employing a suitable deforma- tion history, the LCP phase may be elongated and ori- ented such that microfibrillar morphology can be retained in the solid state. This has important ramifications for the development of self-reinforcing polymer blends to com- pete with conventional inorganic fiber reinforced poly- mers. Shear flows are generally ineffective in developing these morphologies, but flows that incorporate an exten- sional region, such as the converging flow found at the entrance to a capillary or die can produce an elongated LCP phase. Bousmina et al. [29] assessed the phase seg- regation in the poly (styrene-co-acrylonitrile)/poly (methyl methacrylate) (SAN/PMMA) blend with lower critical solution temperature (LCST) by linear viscoelastic rheology, optical microscopy and inverse gas chroma- tography (IGC) techniques for various blend composi- tions. At low temperatures, the blends showed a classical behaviour of homogeneous polymer melts, whereas in the vicinity of phase segregation, a shoulder in the stor- age modulus and in the linear relaxation modulus, G(t) was observed. The width of such a low-frequency/ longer-time plateau and the terminal relaxation time were found to increase with temperature. Such behaviour was attributed to variable morphologies appearing at different temperatures. The development of the morphology was found to take place within a given interval of temperature rather than at a single critical temperature. Optical mi- croscopy and IGC analyses supported the peculiar be- haviour observed, of such a blend. Time-temperature superposition, origin of elasticity, and the Fredrickson and Larson theory were discussed in light of the results obtained. 7. Developments in the Field of Polymer Blend Rheology 7.1. Past, Present and Future Developments in the Field of Polymer Blend Rheology The technology of polymer blends has been one of the major areas of research and development in polymer science in the past three decades. The advantages of polymer blends versus developing new polymeric struc- tures have been well documented. The ability to combine exiting polymers into new compositions with commer- cializable properties offer the advantage of reduced re- search and development expenses compared with the development of new monomers and polymers to yield similar property profile. Another specific advantage of polymer blends versus new monomers/polymers compo- sition is that the blends can often offer property profile combination not easily obtained with new polymeric structures. In rapidly emerging technological landscape, polymer blend technology can quickly respond to devel- oping needs much faster than the time consuming re- search and development involved with new mono- mer/polymer development. The technical response to emerging needs is now primarily directed to polymer blend technology in order to determine if such needs can be met compared to the development of wholly new polymeric compositions [3]. The understanding of poly- mer blend technology to design the specific compositions to meet application requirements is of primary impor- tance. A number of useful analytical and characterization methods have been developed for polymer blends, al- lowing for an improved understanding of the nature of their miscibility and phase behaviours. While miscible polymer blends have attracted consid- erable interest due to the thermodynamic implications and commercial relevance, phase-separated blends have had a prominent role in polymer blend technology. While mechanical compatibility is assured in miscible blends, phase-separated blends can often achieve property ad- vantages not capable with single phase blends [29]. Me- chanical compatibility refers to an average property of the blend constituents in the final blend mechanical properties. Impact modification is a well known virtue of many phase-separated blends but other properties; in- cluding rheology, environmental stress, rupture resis- tance, opacity, flammability, cost reduction, specific film properties, adhesion and performance of coating can be achieved via specific combination of immiscible blends. In order to achieve mechanical compatibility in phase- separated polymer blends, different approaches have been proposed, demonstrated and in many cases com- mercialized. These methods include: addition of graft or block copolymer, interpenetrating polymer network, re- active extrusion, addition of polymeric interfacial agents and minor addition of acid and base compatibilized units. Reactive extrusion is an area that has attracted consider- able interest as a facial means of compatilizing polymer blends, which offers limited performance as simple blends. This technology initially, emerged with “super tough” polyamides, where maleic anhydride grafted onto an ethylene propylene rubber allowed for graft formation with terminal –NH2 groups of polyamide. The resultant graft structure stabilized the interface between the un- grafted constituents and allowed for the desire impact modifications. Copyright © 2011 SciRes. MSA
 Rheological Properties of Polymers: Structure and Morphology of Molten Polymer Blends 38 In engineering polymer blends, a number of advances in the technology and the commercial areas have been realized in the past decades. New commercial polymers have been manufactured by various combination of pre-existing polymers. One of the major areas has been the polyester and polycarbonate (polybutyleneterephtha- late/polycarbonate; polyarylate/polycarbonate; cyclo- hexane dimenthanol-based polyesters/polycarbonate and polyethyleneterephthalate/polycarbonate). The emergence of polymeric material for the future auto panels resulted in a large number of potential candidates, based almost exclusively on engineering polymer blends [30]. The most recent addition to the engineering polymer field is the ethylene carbon monoxide alternating copolymers, initially introduced by Shell. The commercial polymer is highly crystalline and believed to contain small amount of propylene to reduce the crystalline melting point in order to allow a broad window of process ability. There are several areas involving extensive research endeav- ours, presently been investigated that are relevant to polymer blends of the future: These areas include, but not limited to: molecular composites, liquid crystalline polymer blends, blends that involve electrically conduc- tive polymers, blends containing biodegradable polymers and theoretical studies that involve the prediction of polymer phase behaviour. Another interesting area in- volves the computation model predictions of polymer miscibility. Due to the two-phase or multiphase nature and from the macroscopic point of view, the rheological behaviour of incompatible (or heterogeneous in general) polymer blends must be dealt with from phenomenological (i.e. fluid mechanics) consideration. Conversely, the rheo- logical behaviour of miscible polymer blends can be dealt with (or interpreted) using the molecular approach. While there are few miscible polymer blends that have met with commercial success, numerous investigators have reported on the rheological behaviour of miscible polymer blends [31]. Multi-phase polymer blend systems examine the current state-of-the-art, challenges and fu- ture prospects in the field of polymer blends. The hand-picked selection of topics and expert contributors, make this survey of phase morphology in polymer blends an outstanding resource for anyone involved in the field of polymer materials design. It is now well known that morphology is a major parameter to control final proper- ties of immiscible polymer blends. Predicting this mor- phology from the mixing conditions is the next step to develop and produce new performing materials. A uni- versal acceptable theory for describing the evolution of morphology of multi-phase immiscible polymer systems may still be far off, thus polymer blends are becoming more exciting interesting research discipline, in both in- dustrial and academic laboratories around the world. It is obvious that research and development on polymer rheology is purely fundamental and serves long-term objectives. 7.2. Current Research Status The developments of melt rheology of polymer blends depend largely on the understanding of the struc- ture-properties relationship of the materials which re- quires multiscale model to predict the material properties. The current research in the modeling and simulation of polymer blends are largely limited to length and time scale. However it should be noted that some efforts have been made to develop multiscale strategies for the pre- diction of multiscale level structure, properties and proc- essing performance of polymer blends. Some of these works will be mentioned. Bouhlel et al. [32] recently used Biot’s model to describe the quasi-static deforma- tion of a continuous porous medium, saturated by in- compressible Newtonian fluid at a microscopic scale. The microscopic description involves four effective properties, i.e. the effective elasticity tensor, Biot’s ef- fective tensor, the coefficient β and the permeability ten- sor. The numerical results obtained from their work were compared with bounds, self-consistent estimation, exact expansion and experimental result available in the litera- ture on ceramic and metals. Balazs and co-workers [33] recently combined DFT with SCF to calculate phase be- haviour of clay-based polymer nanocomposites and other polymer nanoparticles mixtures. In this model, the ther- modynamic behaviour and interaction information among various components are obtained from the SCF model which served as input to a DFT to calculate the phase behaviour. Chu et al. [34] proposed a modified model of Chen’s for the calculation of the relative vis- cosity of BR-SBS blends of different compositions. The agreement between the theoretical prediction and ex- perimental results was satisfactory. Edwards et al. [35] isothermally studied the dynamic and creep behaviour of 80ln15Pb5Ag and 50Sn50Pb over nine decades of time and frequency. 80ln15Pb5Ag was examined at –6, 21, and 50˚C, while 50Sn50Pb was examined at 21˚C. Vis- coelastic behaviour as a function of temperature and time/frequency was observed at strain less than 10-5. At the small strain level employed in the study, the alloys exhibit linear viscoelasticity rather than the viscoplastic- ity observed at larger strain. Viscoelasticity was subject to constitutive modeling. The creep followed a superpo- sition of a stretched exponential and power law, in time and an Arrhenius form, in temperature. Behaviour was thermorheologically simple over at least nine decades of true time and frequency. Copyright © 2011 SciRes. MSA
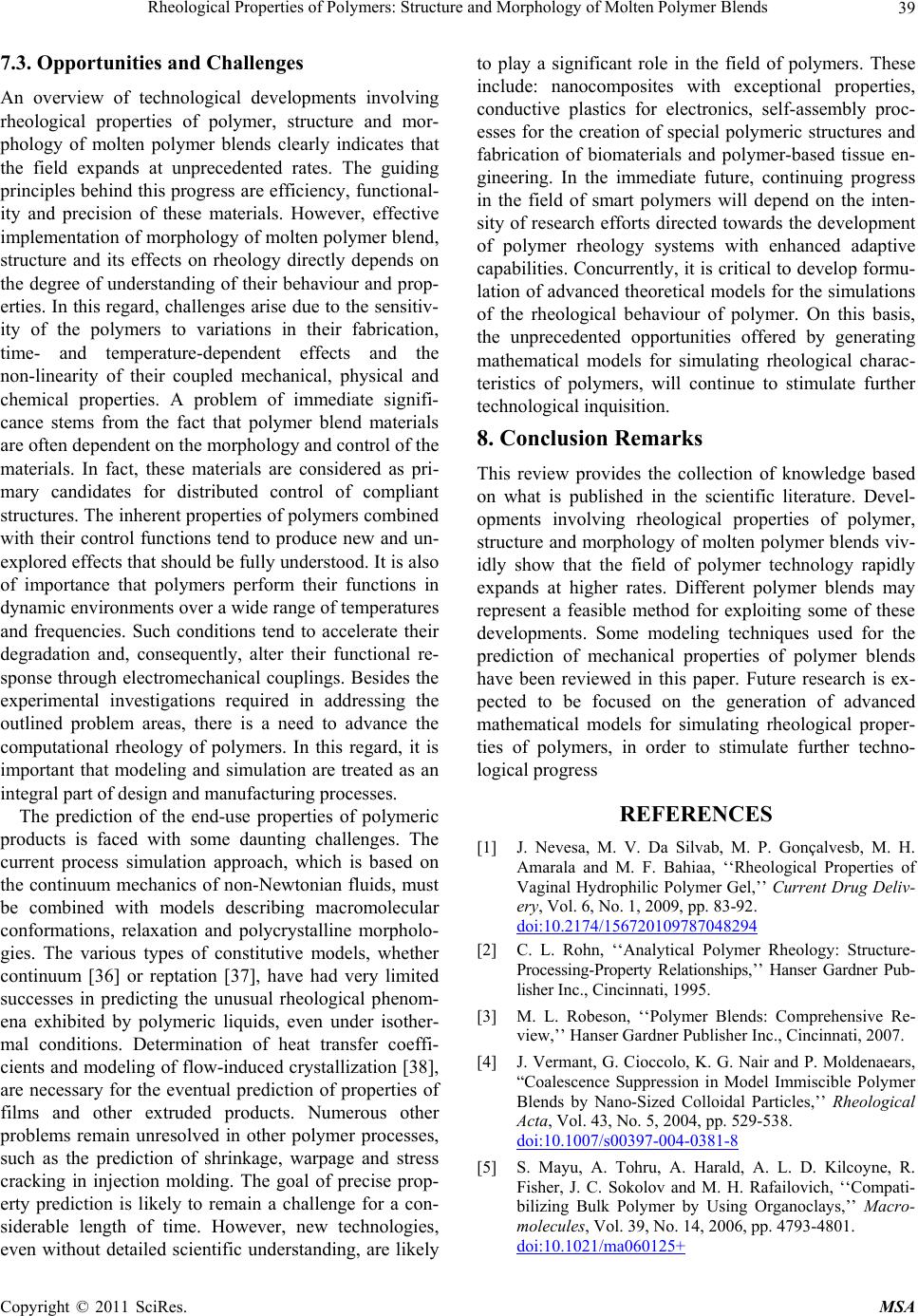 Rheological Properties of Polymers: Structure and Morphology of Molten Polymer Blends39 7.3. Opportunities and Challenges An overview of technological developments involving rheological properties of polymer, structure and mor- phology of molten polymer blends clearly indicates that the field expands at unprecedented rates. The guiding principles behind this progress are efficiency, functional- ity and precision of these materials. However, effective implementation of morphology of molten polymer blend, structure and its effects on rheology directly depends on the degree of understanding of their behaviour and prop- erties. In this regard, challenges arise due to the sensitiv- ity of the polymers to variations in their fabrication, time- and temperature-dependent effects and the non-linearity of their coupled mechanical, physical and chemical properties. A problem of immediate signifi- cance stems from the fact that polymer blend materials are often dependent on the morphology and control of the materials. In fact, these materials are considered as pri- mary candidates for distributed control of compliant structures. The inherent properties of polymers combined with their control functions tend to produce new and un- explored effects that should be fully understood. It is also of importance that polymers perform their functions in dynamic environments over a wide range of temperatures and frequencies. Such conditions tend to accelerate their degradation and, consequently, alter their functional re- sponse through electromechanical couplings. Besides the experimental investigations required in addressing the outlined problem areas, there is a need to advance the computational rheology of polymers. In this regard, it is important that modeling and simulation are treated as an integral part of design and manufacturing processes. The prediction of the end-use properties of polymeric products is faced with some daunting challenges. The current process simulation approach, which is based on the continuum mechanics of non-Newtonian fluids, must be combined with models describing macromolecular conformations, relaxation and polycrystalline morpholo- gies. The various types of constitutive models, whether continuum [36] or reptation [37], have had very limited successes in predicting the unusual rheological phenom- ena exhibited by polymeric liquids, even under isother- mal conditions. Determination of heat transfer coeffi- cients and modeling of flow-induced crystallization [38], are necessary for the eventual prediction of properties of films and other extruded products. Numerous other problems remain unresolved in other polymer processes, such as the prediction of shrinkage, warpage and stress cracking in injection molding. The goal of precise prop- erty prediction is likely to remain a challenge for a con- siderable length of time. However, new technologies, even without detailed scientific understanding, are likely to play a significant role in the field of polymers. These include: nanocomposites with exceptional properties, conductive plastics for electronics, self-assembly proc- esses for the creation of special polymeric structures and fabrication of biomaterials and polymer-based tissue en- gineering. In the immediate future, continuing progress in the field of smart polymers will depend on the inten- sity of research efforts directed towards the development of polymer rheology systems with enhanced adaptive capabilities. Concurrently, it is critical to develop formu- lation of advanced theoretical models for the simulations of the rheological behaviour of polymer. On this basis, the unprecedented opportunities offered by generating mathematical models for simulating rheological charac- teristics of polymers, will continue to stimulate further technological inquisition. 8. Conclusion Remarks This review provides the collection of knowledge based on what is published in the scientific literature. Devel- opments involving rheological properties of polymer, structure and morphology of molten polymer blends viv- idly show that the field of polymer technology rapidly expands at higher rates. Different polymer blends may represent a feasible method for exploiting some of these developments. Some modeling techniques used for the prediction of mechanical properties of polymer blends have been reviewed in this paper. Future research is ex- pected to be focused on the generation of advanced mathematical models for simulating rheological proper- ties of polymers, in order to stimulate further techno- logical progress REFERENCES [1] J. Nevesa, M. V. Da Silvab, M. P. Gonçalvesb, M. H. Amarala and M. F. Bahiaa, ‘‘Rheological Properties of Vaginal Hydrophilic Polymer Gel,’’ Current Drug Deliv- ery, Vol. 6, No. 1, 2009, pp. 83-92. doi:10.2174/156720109787048294 [2] C. L. Rohn, ‘‘Analytical Polymer Rheology: Structure- Processing-Property Relationships,’’ Hanser Gardner Pub- lisher Inc., Cincinnati, 1995. [3] M. L. Robeson, ‘‘Polymer Blends: Comprehensive Re- view,’’ Hanser Gardner Publisher Inc., Cincinnati, 2007. [4] J. Vermant, G. Cioccolo, K. G. Nair and P. Moldenaears, “Coalescence Suppression in Model Immiscible Polymer Blends by Nano-Sized Colloidal Particles,’’ Rheological Acta, Vol. 43, No. 5, 2004, pp. 529-538. doi:10.1007/s00397-004-0381-8 [5] S. Mayu, A. Tohru, A. Harald, A. L. D. Kilcoyne, R. Fisher, J. C. Sokolov and M. H. Rafailovich, ‘‘Compati- bilizing Bulk Polymer by Using Organoclays,’’ Macro- molecules, Vol. 39, No. 14, 2006, pp. 4793-4801. doi:10.1021/ma060125+ Copyright © 2011 SciRes. MSA
 Rheological Properties of Polymers: Structure and Morphology of Molten Polymer Blends 40 [6] H.-K. Young and J.-K. Kim, ‘‘The Effect of the Amount of in Situ Formed Copolymers on the Final Morphology of Reactive Polymer Blends and with in-Situ Compatibi- lizers,’’ Macromolecules, Vol. 31, No. 26, 1998, pp. 9273-9280. doi:10.1021/ma971002f [7] D. Nichetti and I. Manas-Zloczower, ‘‘Viscosity Model for Polydisperse Polymer Melts,’’ Journal of Rheology, Vol. 42, No. 4, 1988, pp. 951-969. doi:10.1122/1.550908 [8] P. R. Soskey and H. H. Winter, ‘‘Equibiaxial Extension of Two Polymer Melts: Polystyrene and Low Density Polyethylene,’’ Journal of Rheology, Vol. 29, No. 5, 1985, pp. 493-517. doi:10.1122/1.549799 [9] C. Harrats, S. Thomas and G. Groeninckx, ‘‘Micro- and Nanostructured Multiphase Polymer Blend Systems: Phase Morphology and Interface,’’ CRC Press, Taylor and Francis, Boca Raton, 2006. [10] R. T. Tol, G. Groeninckx, I. Vinckier, P. Moldenaers and J. Mewis, ‘‘Phase Morphology and Stability of Co-Con- tinuous (PPE/PS)/PA6 and PS/PA6 Blends: Effect of Rheology and Reactive Compatibilization,’’ Polymer, Vol. 45, No. 8, 2004, pp. 2587-2601. doi:10.1016/j.polymer.2003.12.072 [11] W. M. H. Verbeeten, “Computational Polymer Melt Rheology,” Technische Universiteit Eindhoven, Eindho- ven, 2001. [12] R. Seemann, S. Herminghaus, C. Neto, S. Schlagowski, D. Podzimek, R. Konrad, H. Mantz and K. Jacob. “Dynam- ics and Structure Formation in Thin Polymer Melt Films,” Journal of Physics: Condensed Matter, Vol. 17, 2005, pp. S267-S290. doi:10.1088/0953-8984/17/9/001 [13] K. C. Daoulas, V. A. Harmandaris and V. G. Mayrantzas, “Detailed Atomistic Simulation of a Polymer Melt/Solid Interface: Structure, Density, and Conformation of a Thin Film of Polyethylene Melt Adsorbed on Graphite,” Mac- romolecules, Vol. 38, 2005, pp. 5780-5795. doi:10.1021/ma050176r [14] K. Foteinopoulou, N. C. Karayiannis and V. G. Mayrant- zas, “Primitive Path Identification and Entanglement Sta- tistics in Polymer Melts: Results from Direct Topological Analysis on Atomistic Polyethylene Models,” Macro- molecules, Vol. 39, pp. 4207-4216. doi:10.1021/ma060306b [15] T. Araki, Q. Tran-Cong and M. Shibayama, ‘‘Structure and Properties of Multiphase Polymeric Materials,’’ Marcel Dekker Inc., New York, 1998. [16] J. L. White and K. Kim, ‘‘Thermoplastic and Rubber Compounds: Technology and Physical Chemistry,” Han- ser Gardner Publisher Inc., Cincinnati, 2008. [17] L. Elias, F. Fenouillot, J. C. Majeste and P. H. Cassagnau, ‘‘Morphology and Rheology of Polymer Blends Filled with Silica Nano Particles,’’ 34th Journees d’Etude des Polymers, Lorient, September 2006, pp. 24-29. [18] L. A. Utracki, ‘‘Polymer Blends,’’ RAPRA Review Re- ports, Vol. 11, No. 3, 2000, pp. 1-170. [19] S. Velankar, P. V. Puyvelde, J. Mewis and P. Moldenaers, ‘‘Steady-Shear Rheological Properties of Model Com- patibilized Blends,’’ Journal of Rheology, Vol. 48, No. 725, 2004, pp. 725-744. doi:10.1122/1.1765662 [20] X. Lu and U. Isacsson, ‘‘Rheological Characterization of Styrene-Butadiene-Styrene Copolymer Modified Bitu- men,’’ Construction and Building Materials, Vol. 11, No. 1, 1997, pp. 23-32. doi:10.1016/S0950-0618(96)00033-5 [21] A. K. William and N. J. Wagner, ‘‘The Role of Liquid- Crystalline Polymer Rheology on the Evolving Morphol- ogy of Immiscible Blends Containing Liquid -Crystalline Polymers,’’ Journal of Rheology, Vol. 43, No. 521, 1999, pp. 521-549. [22] S. W. Kuo and F. C. Chang, ‘Studies of Miscibility Be- haviour and Hydrogen Bonding in Blends of Poly (Vi- nylphenol) and Poly (Vinylpyrrolidone),’’ Macromole- cules, Vol. 34, No. 15, 2001, pp. 5224-5228. doi:10.1021/ma010517a [23] J.-H. Kim, M.-J. Kim, C.-K. Kim and J.-W. Lee, ‘‘Con- trol of Morphology and Interfacial Tension of PC/SAN Blends with Compatibilizer,’’ Korea Australia Rheology Journal, Vol. 13, No. 3, 2001, pp. 125-130. [24] H. S. Jeon, A. I. Nakatani, C. C. Han and R. H. Colby, ‘‘Melt Rheology of Lower Critical Solution Temperature Polybutadiene/Polyisoprene Blends,’’ Macromolecules, Vol. 33, 2000, pp. 9732-9739. doi:10.1021/ma000714u [25] M. Kroger and S. Hess, ‘‘Rheological Evidence of Dy- namical Crossover in Polymer Melts via Nonequilibrium Molecular Dynamic,’’ Physical Review Letters, Vol. 85, No. 5, 2000, pp. 1128-1131. doi:10.1103/PhysRevLett.85.1128 [26] N. Sivashinsky, T. J. Moon and D. S. Soong, ‘‘Transient Structures and Rheological Properties of SBS Block Co- polymer Melts,’’ Journal of Macromolecular Science, Part B, Vol. 22, No. 2, 1983, pp. 213-229. [27] Q. Zheng, M. Zuo, M. Peng, L. Shen and Y. Fan, ‘‘Rheological Study of Microstructures and Properties for Polymeric Materials,’’ Frontiers of Material Science in China, Vol. 1, No. 1, 2007, pp. 1-6. doi:10.1007/s11706-007-0001-5 [28] A. Kohli, N. Chung and R. A. Weiss, ‘‘The Effect of Deformation History on the Morphology and Properties of Blends of Polycarbonate and a Thermotropic Liquid Crystalline Polymer,’’ Polymer Engineering and Science, Vol. 29, 1989, pp. 573-580. doi:10.1002/pen.760290902 [29] M. Bousmina, A. Lavoie and B. Riedl, ‘‘Phase Segrega- tion in SAN/PMMA Blends Probed by Rheology, Mi- croscopy, and Inverse Gas Chromatography Tech- niques,’’ Macromolecules, Vol. 35, No. 16, 2002, pp. 6274-6283. doi:10.1021/ma020053w [30] L. A. Utracki, ‘‘Polymer Blends Handbook,’’ Kluwer Copyright © 2011 SciRes. MSA
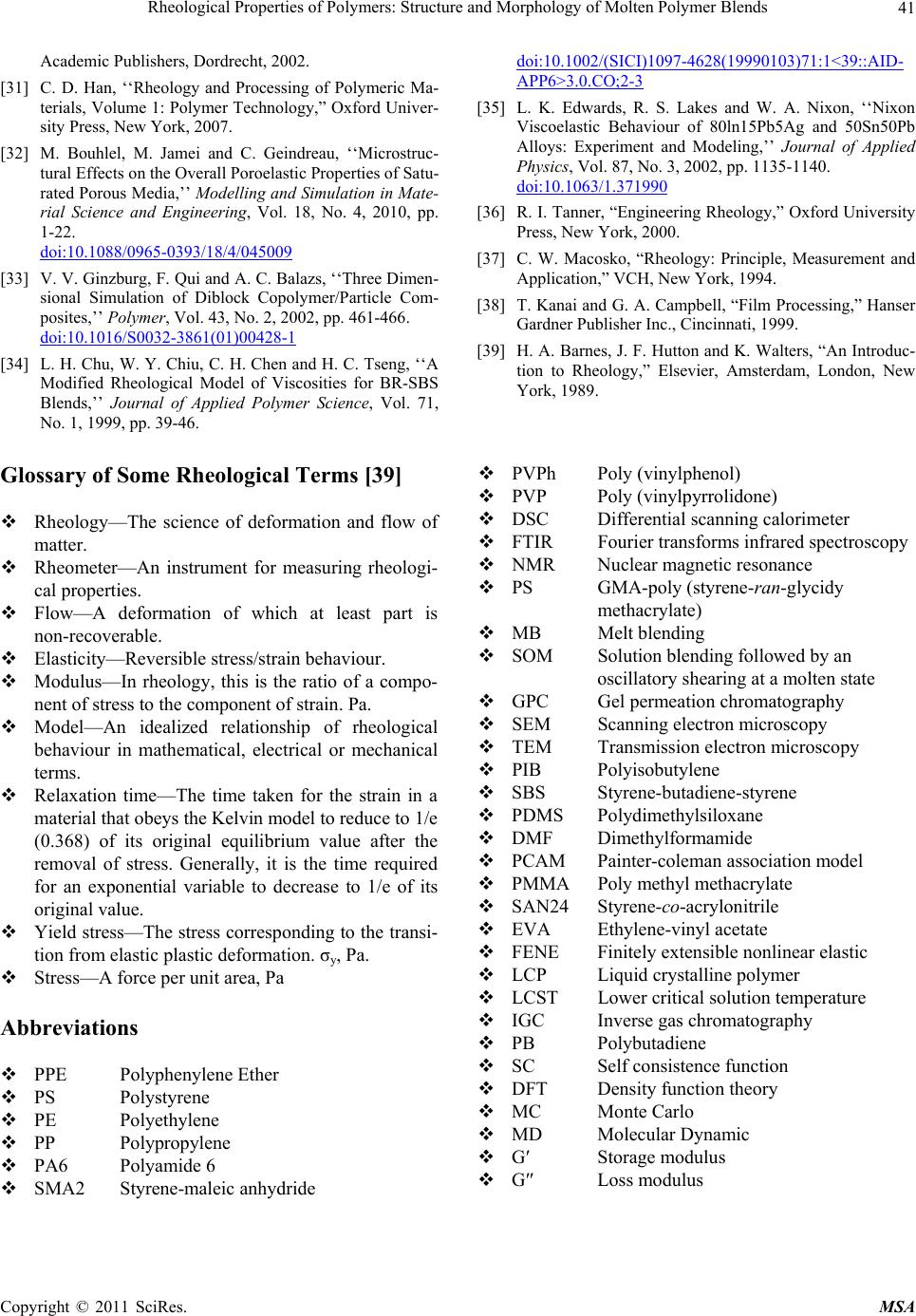 Rheological Properties of Polymers: Structure and Morphology of Molten Polymer Blends Copyright © 2011 SciRes. MSA 41 Academic Publishers, Dordrecht, 2002. [31] C. D. Han, ‘‘Rheology and Processing of Polymeric Ma- terials, Volume 1: Polymer Technology,” Oxford Univer- sity Press, New York, 2007. [32] M. Bouhlel, M. Jamei and C. Geindreau, ‘‘Microstruc- tural Effects on the Overall Poroelastic Properties of Satu- rated Porous Media,’’ Modelling and Simulation in Mate- rial Science and Engineering, Vol. 18, No. 4, 2010, pp. 1-22. doi:10.1088/0965-0393/18/4/045009 [33] V. V. Ginzburg, F. Qui and A. C. Balazs, ‘‘Three Dimen- sional Simulation of Diblock Copolymer/Particle Com- posites,’’ Polymer, Vol. 43, No. 2, 2002, pp. 461-466. doi:10.1016/S0032-3861(01)00428-1 [34] L. H. Chu, W. Y. Chiu, C. H. Chen and H. C. Tseng, ‘‘A Modified Rheological Model of Viscosities for BR-SBS Blends,’’ Journal of Applied Polymer Science, Vol. 71, No. 1, 1999, pp. 39-46. doi:10.1002/(SICI)1097-4628(19990103)71:1<39::AID- APP6>3.0.CO;2-3 [35] L. K. Edwards, R. S. Lakes and W. A. Nixon, ‘‘Nixon Viscoelastic Behaviour of 80ln15Pb5Ag and 50Sn50Pb Alloys: Experiment and Modeling,’’ Journal of Applied Physics, Vol. 87, No. 3, 2002, pp. 1135-1140. doi:10.1063/1.371990 [36] R. I. Tanner, “Engineering Rheology,” Oxford University Press, New York, 2000. [37] C. W. Macosko, “Rheology: Principle, Measurement and Application,” VCH, New York, 1994. [38] T. Kanai and G. A. Campbell, “Film Processing,” Hanser Gardner Publisher Inc., Cincinnati, 1999. [39] H. A. Barnes, J. F. Hutton and K. Walters, “An Introduc- tion to Rheology,” Elsevier, Amsterdam, London, New York, 1989. Glossary of Some Rheological Terms [39] Rheology—The science of deformation and flow of matter. Rheometer—An instrument for measuring rheologi- cal properties. Flow—A deformation of which at least part is non-recoverable. Elasticity—Reversible stress/strain behaviour. Modulus—In rheology, this is the ratio of a compo- nent of stress to the component of strain. Pa. Model—An idealized relationship of rheological behaviour in mathematical, electrical or mechanical terms. Relaxation time—The time taken for the strain in a material that obeys the Kelvin model to reduce to 1/e (0.368) of its original equilibrium value after the removal of stress. Generally, it is the time required for an exponential variable to decrease to 1/e of its original value. Yield stress—The stress corresponding to the transi- tion from elastic plastic deformation. σy, Pa. Stress—A force per unit area, Pa Abbreviations PPE Polyphenylene Ether PS Polystyrene PE Polyethylene PP Polypropylene PA6 Polyamide 6 SMA2 Styrene-maleic anhydride PVPh Poly (vinylphenol) PVP Poly (vinylpyrrolidone) DSC Differential scanning calorimeter FTIR Fourier transforms infrared spectroscopy NMR Nuclear magnetic resonance PS GMA-poly (styrene-ran-glycidy methacrylate) MB Melt blending SOM Solution blending followed by an oscillatory shearing at a molten state GPC Gel permeation chromatography SEM Scanning electron microscopy TEM Transmission electron microscopy PIB Polyisobutylene SBS Styrene-butadiene-styrene PDMS Polydimethylsiloxane DMF Dimethylformamide PCAM Painter-coleman association model PMMA Poly methyl methacrylate SAN24 Styrene-co-acrylonitrile EVA Ethylene-vinyl acetate FENE Finitely extensible nonlinear elastic LCP Liquid crystalline polymer LCST Lower critical solution temperature IGC Inverse gas chromatography PB Polybutadiene SC Self consistence function DFT Density function theory MC Monte Carlo MD Molecular Dynamic G′ Storage modulus G″ Loss modulus
|