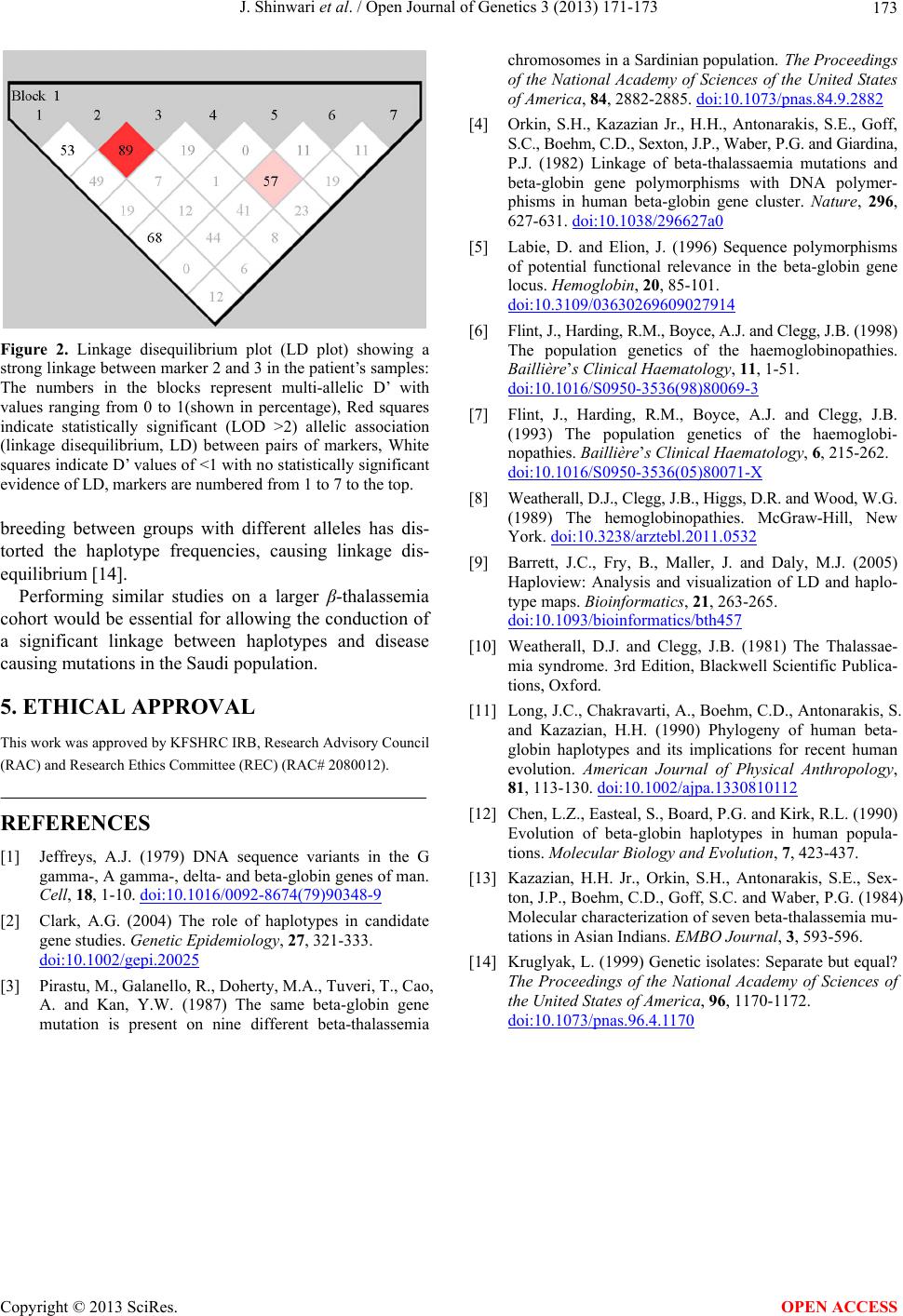
J. Shinwari et al. / Open Journal of Genetics 3 (2013) 171-173
Copyright © 2013 SciRes.
173
OPEN ACCESS
Figure 2. Linkage disequilibrium plot (LD plot) showing a
strong linkage between marker 2 and 3 in the patient’s samples:
The numbers in the blocks represent multi-allelic D’ with
values ranging from 0 to 1(shown in percentage), Red squares
indicate statistically significant (LOD >2) allelic association
(linkage disequilibrium, LD) between pairs of markers, White
squares indicate D’ values of <1 with no statistically significant
evidence of LD, markers are numbered from 1 to 7 to the top.
breeding between groups with different alleles has dis-
torted the haplotype frequencies, causing linkage dis-
equilibrium [14].
Performing similar studies on a larger β-thalassemia
cohort would be essential for allowing the conduction of
a significant linkage between haplotypes and disease
causing mutations in the Saudi population.
5. ETHICAL APPROVAL
This work was approved by KFSHRC IRB, Research Advisory Council
(RAC) and Research Ethics Committee (REC) (RAC# 2080012).
REFERENCES
[1] Jeffreys, A.J. (1979) DNA sequence variants in the G
gamma-, A gamma-, delta- and beta-globin genes of man.
Cell, 18, 1-10. doi:10.1016/0092-8674(79)90348-9
[2] Clark, A.G. (2004) The role of haplotypes in candidate
gene studies. Genetic Epidemiology, 27, 321-333.
doi:10.1002/gepi.20025
[3] Pirastu, M., Galanello, R., Doherty, M.A., Tuveri, T., Cao,
A. and Kan, Y.W. (1987) The same beta-globin gene
mutation is present on nine different beta-thalassemia
chromosomes in a Sardinian population. The Proceedings
of the National Academy of Sciences of the United States
of America, 84, 2882-2885. doi:10.1073/pnas.84.9.2882
[4] Orkin, S.H., Kazazian Jr., H.H., Antonarakis, S.E., Goff,
S.C., Boehm, C.D., Sexton, J.P., Waber, P.G. and Giardina,
P.J. (1982) Linkage of beta-thalassaemia mutations and
beta-globin gene polymorphisms with DNA polymer-
phisms in human beta-globin gene cluster. Nature, 296,
627-631. doi:10.1038/296627a0
[5] Labie, D. and Elion, J. (1996) Sequence polymorphisms
of potential functional relevance in the beta-globin gene
locus. Hemoglobin, 20, 85-101.
doi:10.3109/03630269609027914
[6] Flint, J., Harding, R.M., Boyce, A.J. and Clegg, J.B. (1998)
The population genetics of the haemoglobinopathies.
Baillière’s Clinical Haematology, 11, 1-51.
doi:10.1016/S0950-3536(98)80069-3
[7] Flint, J., Harding, R.M., Boyce, A.J. and Clegg, J.B.
(1993) The population genetics of the haemoglobi-
nopathies. Baillière’s Clinical Haematology, 6, 215-262.
doi:10.1016/S0950-3536(05)80071-X
[8] Weatherall, D.J., Clegg, J.B., Higgs, D.R. and Wood, W.G.
(1989) The hemoglobinopathies. McGraw-Hill, New
York. doi:10.3238/arztebl.2011.0532
[9] Barrett, J.C., Fry, B., Maller, J. and Daly, M.J. (2005)
Haploview: Analysis and visualization of LD and haplo-
type maps. Bioinformatics, 21, 263-265.
doi:10.1093/bioinformatics/bth457
[10] Weatherall, D.J. and Clegg, J.B. (1981) The Thalassae-
mia syndrome. 3rd Edition, Blackwell Scientific Publica-
tions, Oxford.
[11] Long, J.C., Chakravarti, A., Boehm, C.D., Antonarakis, S.
and Kazazian, H.H. (1990) Phylogeny of human beta-
globin haplotypes and its implications for recent human
evolution. American Journal of Physical Anthropology,
81, 113-130. doi:10.1002/ajpa.1330810112
[12] Chen, L.Z., Easteal, S., Board, P.G. and Kirk, R.L. (1990)
Evolution of beta-globin haplotypes in human popula-
tions. Molecular Biology and Evolution, 7, 423-437.
[13] Kazazian, H.H. Jr., Orkin, S.H., Antonarakis, S.E., Sex-
ton, J.P., Boehm, C.D., Goff, S.C. and Waber, P.G. (1984)
Molecular characterization of seven beta-thalassemia mu-
tations in Asian Indians. EMBO Journal, 3, 593-596.
[14] Kruglyak, L. (1999) Genetic isolates: Separate but equal?
The Proceedings of the National Academy of Sciences of
the United States of America, 96, 1170-1172.
doi:10.1073/pnas.96.4.1170