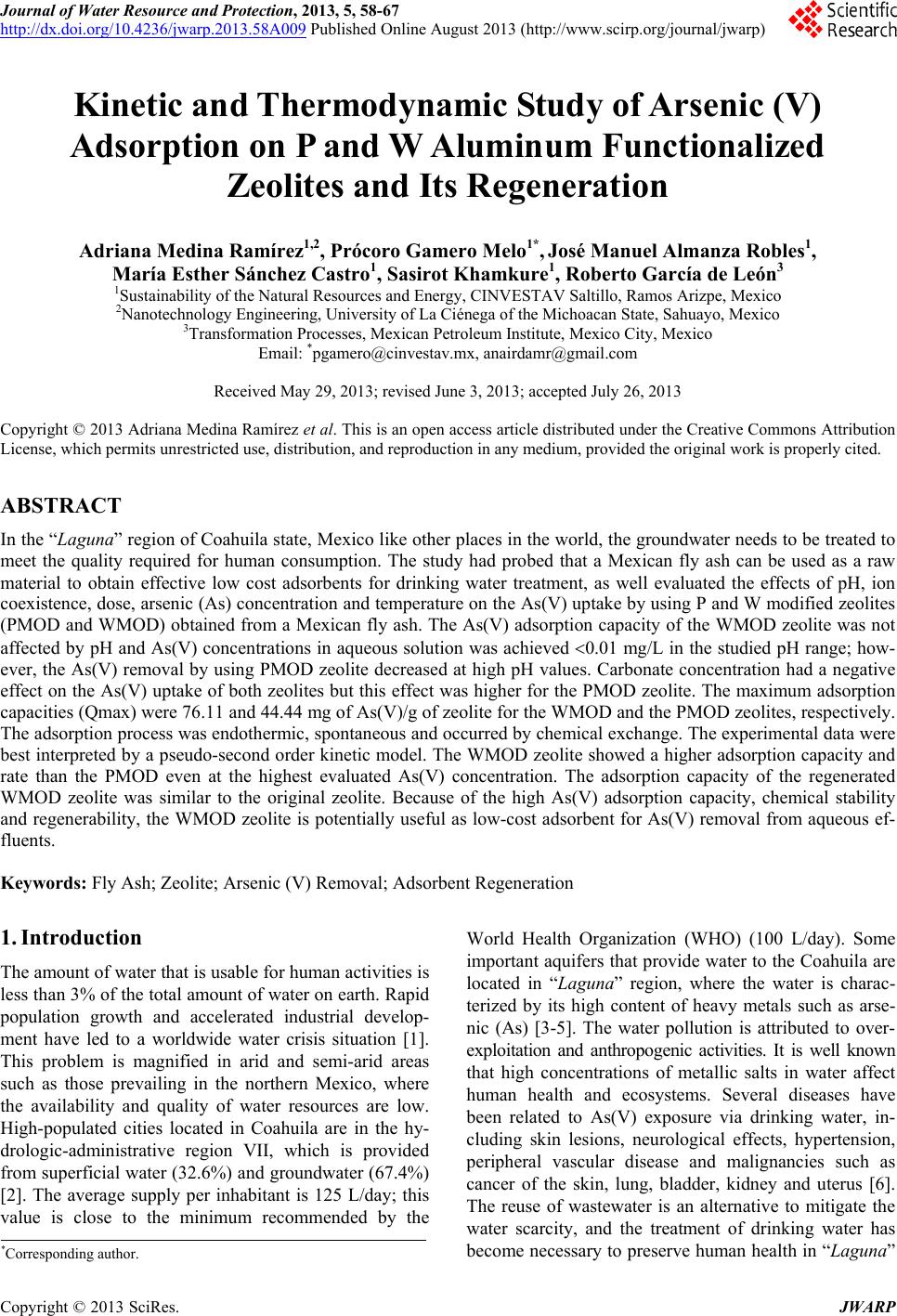 Journal of Water Resource and Protection, 2013, 5, 58-67 http://dx.doi.org/10.4236/jwarp.2013.58A009 Published Online August 2013 (http://www.scirp.org/journal/jwarp) Kinetic and Thermodynamic Study of Arsenic (V) Adsorption on P and W Aluminum Functionalized Zeolites and Its Regeneration Adriana Medina Ramírez1,2, Prócoro Gamero Melo1*, José Manuel Almanza Robles1, María Esther Sánchez Castro1, Sasirot Khamkure1, Roberto García de León3 1Sustainability of the Natural Resources and Energy, CINVESTAV Saltillo, Ramos Arizpe, Mexico 2Nanotechnology Engineering, University of La Ciénega of the Michoacan State, Sahuayo, Mexico 3Transformation Processes, Mexican Petroleum Institute, Mexico City, Mexico Email: *pgamero@cinvestav.mx, anairdamr@gmail.com Received May 29, 2013; revised June 3, 2013; accepted July 26, 2013 Copyright © 2013 Adriana Medina Ramírez et al. This is an open access article distributed under the Creative Commons Attribution License, which permits unrestricted use, distribution, and reproduction in any medium, provided the original work is properly cited. ABSTRACT In the “Laguna” region of Coahuila state, Mexico like other places in the world, the groundwater needs to be treated to meet the quality required for human consumption. The study had probed that a Mexican fly ash can be used as a raw material to obtain effective low cost adsorbents for drinking water treatment, as well evaluated the effects of pH, ion coexistence, dose, arsenic (As) concentration and temperature on the As(V) uptake by using P and W modified zeolites (PMOD and WMOD) obtained from a Mexican fly ash. The As(V) adsorption capacity of the WMOD zeolite was not affected by pH and As(V) concentrations in aqueous solution was achieved 0.01 mg/L in the studied pH range; how- ever, the As(V) removal by using PMOD zeolite decreased at high pH values. Carbonate concentration had a negative effect on the As(V) uptake of both zeolites but this effect was higher for the PMOD zeolite. The maximum adsorption capacities (Qmax) were 76.11 and 44.44 mg of As(V)/g of zeolite for the WMOD and the PMOD zeolites, respectively. The adsorption process was endothermic, spontaneous and occurred by chemical exchange. The experimental data were best interpreted by a pseudo-second order kinetic model. The WMOD zeolite showed a higher adsorption capacity and rate than the PMOD even at the highest evaluated As(V) concentration. The adsorption capacity of the regenerated WMOD zeolite was similar to the original zeolite. Because of the high As(V) adsorption capacity, chemical stability and regenerability, the WMOD zeolite is potentially useful as low-cost adsorbent for As(V) removal from aqueous ef- fluents. Keywords: Fly Ash; Zeolite; Arsenic (V) Removal; Adsorbent Regeneration 1. Introduction The amount of water that is usable for human activities is less than 3% of the total amount of water on earth. Rapid population growth and accelerated industrial develop- ment have led to a worldwide water crisis situation [1]. This problem is magnified in arid and semi-arid areas such as those prevailing in the northern Mexico, where the availability and quality of water resources are low. High-populated cities located in Coahuila are in the hy- drologic-administrative region VII, which is provided from superficial water (32.6%) and groundwater (67.4%) [2]. The average supply per inhabitant is 125 L/day; this value is close to the minimum recommended by the World Health Organization (WHO) (100 L/day). Some important aquifers that provide water to the Coahuila are located in “Laguna” region, where the water is charac- terized by its high content of heavy metals such as arse- nic (As) [3-5]. The water pollution is attributed to over- exploitation and anthropogenic activities. It is well known that high concentrations of metallic salts in water affect human health and ecosystems. Several diseases have been related to As(V) exposure via drinking water, in- cluding skin lesions, neurological effects, hypertension, peripheral vascular disease and malignancies such as cancer of the skin, lung, bladder, kidney and uterus [6]. The reuse of wastewater is an alternative to mitigate the water scarcity, and the treatment of drinking water has become necessary to preserve human health in “Laguna” *Corresponding author. C opyright © 2013 SciRes. JWARP
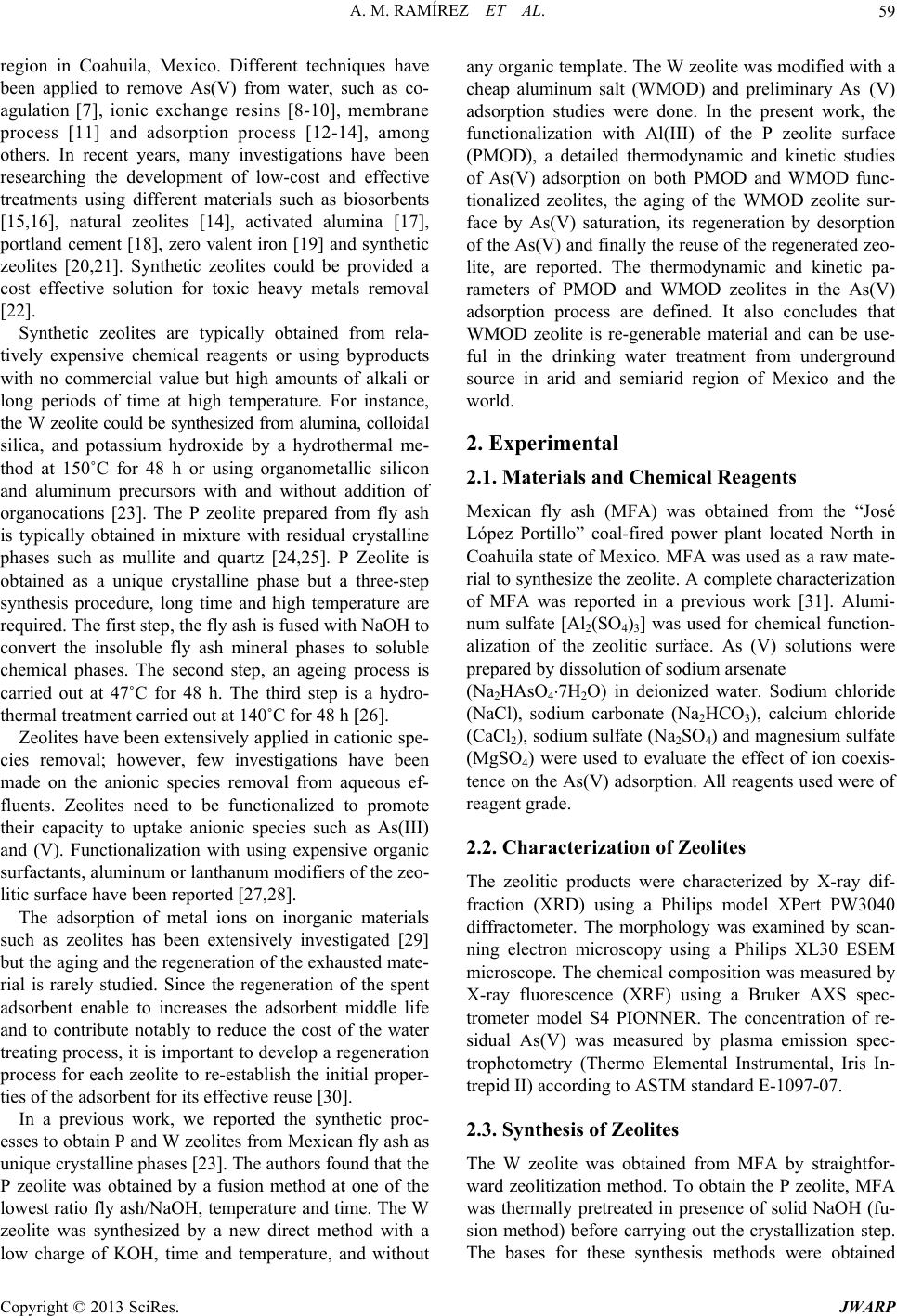 A. M. RAMÍREZ ET AL. 59 region in Coahuila, Mexico. Different techniques have been applied to remove As(V) from water, such as co- agulation [7], ionic exchange resins [8-10], membrane process [11] and adsorption process [12-14], among others. In recent years, many investigations have been researching the development of low-cost and effective treatments using different materials such as biosorbents [15,16], natural zeolites [14], activated alumina [17], portland cement [18], zero valent iron [19] and synthetic zeolites [20,21]. Synthetic zeolites could be provided a cost effective solution for toxic heavy metals removal [22]. Synthetic zeolites are typically obtained from rela- tively expensive chemical reagents or using byproducts with no commercial value but high amounts of alkali or long periods of time at high temperature. For instance, the W zeolite could be synthesized from alumina, colloidal silica, and potassium hydroxide by a hydrothermal me- thod at 150˚C for 48 h or using organometallic silicon and aluminum precursors with and without addition of organocations [23]. The P zeolite prepared from fly ash is typically obtained in mixture with residual crystalline phases such as mullite and quartz [24,25]. P Zeolite is obtained as a unique crystalline phase but a three-step synthesis procedure, long time and high temperature are required. The first step, the fly ash is fused with NaOH to convert the insoluble fly ash mineral phases to soluble chemical phases. The second step, an ageing process is carried out at 47˚C for 48 h. The third step is a hydro- thermal treatment carried out at 140˚C for 48 h [26]. Zeolites have been extensively applied in cationic spe- cies removal; however, few investigations have been made on the anionic species removal from aqueous ef- fluents. Zeolites need to be functionalized to promote their capacity to uptake anionic species such as As(III) and (V). Functionalization with using expensive organic surfactants, aluminum or lanthanum modifiers of the zeo- litic surface have been reported [27,28]. The adsorption of metal ions on inorganic materials such as zeolites has been extensively investigated [29] but the aging and the regeneration of the exhausted mate- rial is rarely studied. Since the regeneration of the spent adsorbent enable to increases the adsorbent middle life and to contribute notably to reduce the cost of the water treating process, it is important to develop a regeneration process for each zeolite to re-establish the initial proper- ties of the adsorbent for its effective reuse [30]. In a previous work, we reported the synthetic proc- esses to obtain P and W zeolites from Mexican fly ash as unique crystalline phases [23]. The authors found that the P zeolite was obtained by a fusion method at one of the lowest ratio fly ash/NaOH, temperature and time. The W zeolite was synthesized by a new direct method with a low charge of KOH, time and temperature, and without any organic template. The W zeolite was modified with a cheap aluminum salt (WMOD) and preliminary As (V) adsorption studies were done. In the present work, the functionalization with Al(III) of the P zeolite surface (PMOD), a detailed thermodynamic and kinetic studies of As(V) adsorption on both PMOD and WMOD func- tionalized zeolites, the aging of the WMOD zeolite sur- face by As(V) saturation, its regeneration by desorption of the As(V) and finally the reuse of the regenerated zeo- lite, are reported. The thermodynamic and kinetic pa- rameters of PMOD and WMOD zeolites in the As(V) adsorption process are defined. It also concludes that WMOD zeolite is re-generable material and can be use- ful in the drinking water treatment from underground source in arid and semiarid region of Mexico and the world. 2. Experimental 2.1. Materials and Chemical Reagents Mexican fly ash (MFA) was obtained from the “José López Portillo” coal-fired power plant located North in Coahuila state of Mexico. MFA was used as a raw mate- rial to synthesize the zeolite. A complete characterization of MFA was reported in a previous work [31]. Alumi- num sulfate [Al2(SO4)3] was used for chemical function- alization of the zeolitic surface. As (V) solutions were prepared by dissolution of sodium arsenate (Na2HAsO47H2O) in deionized water. Sodium chloride (NaCl), sodium carbonate (Na2HCO3), calcium chloride (CaCl2), sodium sulfate (Na2SO4) and magnesium sulfate (MgSO4) were used to evaluate the effect of ion coexis- tence on the As(V) adsorption. All reagents used were of reagent grade. 2.2. Characterization of Zeolites The zeolitic products were characterized by X-ray dif- fraction (XRD) using a Philips model XPert PW3040 diffractometer. The morphology was examined by scan- ning electron microscopy using a Philips XL30 ESEM microscope. The chemical composition was measured by X-ray fluorescence (XRF) using a Bruker AXS spec- trometer model S4 PIONNER. The concentration of re- sidual As(V) was measured by plasma emission spec- trophotometry (Thermo Elemental Instrumental, Iris In- trepid II) according to ASTM standard E-1097-07. 2.3. Synthesis of Zeolites The W zeolite was obtained from MFA by straightfor- ward zeolitization method. To obtain the P zeolite, MFA was thermally pretreated in presence of solid NaOH (fu- sion method) before carrying out the crystallization step. The bases for these synthesis methods were obtained Copyright © 2013 SciRes. JWARP
 A. M. RAMÍREZ ET AL. 60 from experimental design reported by our research group in Medina et al. [23]. The W zeolite was synthesized as follows: 160 g of MFA was added to 480 mL of KOH solution, maintaining a KOH/MFA ratio of 0.33. The mixture was transferred to a ParrTM reactor with a capac- ity of 1 L. A hydrothermal treatment was then performed at 175˚C for 16 h. To obtain the P zeolite, MFA (76 g) was mixed with NaOH, maintaining a NaOH/MFA ratio of 1.04. The mixture was homogenized and heated at 600˚C for 2 h. The resultant fusion product was then added to a flask containing 630 mL of deionized water and aged for 17 h at room temperature. The aged product was crystal- lized at 120˚C for 16 h. Both crystallization products were recovered and washed 3 times with 200 mL of de- ionized water. The final products were dried at 120˚C for 12 h; 160 g of the W zeolite and 77 g of the P zeolite were obtained. 2.4. Functionalization of the Zeolitic Surface The chemical modification of the zeolitic surface was per- formed according to the method reported by Xu et al. [32]. Ten grams of zeolite was added to 1 L of an Al2(SO4)3 7 mM solution and was stirred at room tem- perature (23˚C) for 15 h. Subsequently, the modified zeo- lite was washed three times with 200 mL of deionized water, filtered and dried at 110 ˚C for 12 h. Nine grams of each modified zeolite was obtained and labeled as WMOD and PMOD. 2.5. Adsorption of As(V) on Modified Zeolites 2.5.1. Effect of pH The initial pH of the As(V) solution was varied from 3 to 10 for both the WMOD and PMOD zeolites. One gram of zeolite was added to 100 mL of 0.72 mg/L As(V) so- lution. The slurry was stirred at 25˚C for 2 and 5 h for the WMOD and PMOD zeolites, respectively. Afterward, an aliquot of 10 mL was taken to determine the residual As(V) concentration. The zeolites were characterized by XRD to determine the structural changes due to pH va- riation. 2.5.2. Effect of Ions Coexistence The groundwater in arid regions of Mexico presents a high content of ions such as calcium, magnesium, car- bonates, sulfates and chlorides. To establish the basis of a process of drinking water treatment, the effect of these ions on As(V) adsorption was evaluated in model solu- tions at different concentrations of: Ca2+, Mg2+ and SO42− (100, 500 and 100 mg/L) and Cl− and HCO31− (100, 300 and 500 mg/L). Salt was added to 100 mL of a 1.4 mg/L As(V) solution under stirring, and then 0.5 g of the WMOD zeolite was added. The mixture was stirred for 2 h. Then, an aliquot was taken to measure the As(V) con- centration. The same procedure was applied for the PMOD zeolite, but the zeolite dosage was 1 g, the As(V) concentration was 0.72 mg/L and the adsorption time was 5 h. 2.5.3. Effect of Temperature and Zeolite Dosage To determine the adsorption capacity of As(V) on the modified zeolites, three temperatures were studied: 15˚C, 25˚C and 35˚C. The experiments were performed in a constant temperature bath. The zeolite dosage was varied 0.05 - 1.2 g and was added to 100 mL of an As(V) solu- tion of 0.72 mg/L. The samples were stirred for 18 h. The filtrates were analyzed for residual As(V) concentration. 2.5.4. Effect of Arsenic Concentration Five different As(V) concentrations were evaluated (8.45, 1.45, 0.72, 0.45 and 0.1 mg/L), the dosage used was of 1 g, except for the two last concentrations, where the dos- age was 0.05 g and 0.01 g, respectively. The zeolite was added to 100 mL of an As(V) solution under stirring and was kept for 8 h. Aliquots of 10 mL were taken at dif- ferent intervals of time. 2.6. Accelerated Aging and Regeneration of the WMOD Zeolite Exhausted with As(V) The WMOD zeolite was aged using three high As(V) concentrations: 8, 35 and 359 mg/L. The experiments were performed with 100 mL of As(V) solution and 1 g of zeolite during a time period of 15 h. For a concentra- tion of 359 mg of As(V)/L, the adsorption time was 48 h. The procedure was as follows: the zeolite was added to 100 mL of As(V) solution, this was kept under stirring for a time period. Then an aliquot of 10 mL was taken in order to determine the residual arsenic concentration. Afterward the zeolite was recovered and dried in air. Then, the zeolite was treated with fresh arsenic solution; this procedure was repeated until the zeolite was ex- hausted. In the first step of the regeneration process As(V) was desorbed from the WMOD zeolite using NaOH 0.01 M during a time period of 2 h. In the second step the origi- nal active surface of the WMOD zeolite was regenerated with aluminum salt following the method described in the Section 2.4. The adsorption capacity of the regenerated adsorbent WMOD was evaluated under the same conditions to de- termine the effect of the treatments to which the zeolite was subjected. 3. Results and Discussion 3.1. Characterization of the WMOD and PMOD Zeolites The physicochemical properties of the unmodified and Copyright © 2013 SciRes. JWARP
 A. M. RAMÍREZ ET AL. 61 modified zeolites are summarized in Table 1. The Si/Al molar ratio of both zeolites decreased after the modifica- tion treatment due to an increase in the aluminum content. The textural properties of the W zeolite were affected by the surface modification (the specific surface area calcu- lated by the BET method (SBET) and the pore volume decreased to approximately 40%), whereas the P zeolite’s textural properties were not affected. This result could be related to the difference in the zeolite’s structure and the positions of the extra-frame- work ions (Figure 1). The W zeolite’s structure is similar to that of merli- noite, a natural zeolite. The framework is built of double 8-member rings laterally linked by 4-member rings. This framework results in the formation of two types of cages [33]. According to the last refinement [34], three extra- framework cation sites can be identified in merlinoite. One site is at the center of the buckled 8-member rings of the pau cage and has a high occupancy (M1) that is gen- erally filled by K+ ions. The other sites are two mirror positions above and below the flat 8-member ring win- dow; the former (M2) has a reasonably high occupancy that is still available for K+, and the latter (M3) is inside of the 8-8 SBU with a low occupancy. There is dis- agreement among other authors about site M2, who indi- cate other additional positions, one of which is at the center of the pau cage and is usually filled by smaller cations (Na+, Ca2+). Consequently, a high number of po- tassium ions occupy the center of the D8R (double eight-member rings); when these ions are exchanged with aluminum ions, they partially obstruct the entry of Table 1. Zeolites physicochemical properties. Zeolite Si/Al Bulk density (g·cm−3) SBET ( m2·g−1) Vpore (cm3·g−1) Al3+ exchanged (mmol·g−1) W 2.05 - 28.48 0.132 - WMOD 1.78 0.62 17.26 0.084 0.59 P 1.46 - 51.58 0.124 - PMOD 1.15 0.45 51.24 0.148 0.85 SBET = specific surface area determined by BET method, Vpore = total pore volume. Figure 1. Extra-framework sites in the structure of the W and P zeolites (Adapted from Mortier [37]). nitrogen to larger cavities, resulting in a decrease of the specific surface area. However, the P zeolite has a similar structure to that of gismondine. This zeolite’s framework is characterized by perpendicular double crankshaft chains of tetrahedra. These tetrahedra are connected at all corners, thus form- ing pore systems that consist of two eight-membered ring (8 MR) channels connected by four-membered rings (4 MRs). This topology has a high framework flexibility, which allows the accommodation of a wide range of extra- framework cations [35,36]. There are three sites for cations; sodium ions are lo- cated in both sides of the eight-ring channels, coordi- nated to the walls of both 8 MR channels, allowing pas- sage through the channels [36]. This behavior could ex- plain the fact that the BET area was not modified after the exchange treatment. 3.2. pH Effect on the As(V) Removal The effect of the initial pH on the As(V) removal is shown in Figure 2. The As(V) adsorption capacity of the WMOD zeolite was not affected by the initial pH; the As (V) removal was higher than 98% for the range of pH evaluated (3 - 10). The equilibrium pH was lower than 6, with the exception of when the initial pH was 10. The PMOD zeolite’s As(V) uptake decreased at a pHo higher than 8. At pHo < 8, the As(V) removal was 90%, whereas at pHo > 8, the As(V) removal was only 48%. According to Onyango et al. [27], when the effect of anionic adsorption is considered, the overall changes in the solution pH are related to the protonated (2 AlOH ), neutral (AlOH) and hydrolyzed (AlO−) surface sites pre- sent in the zeolite-metal surface. When the pH system Figure 2. pH effect on As(V) adsorption on the functional- ized zeolites [Co = 0.72 mg/L; dose = 1 g; t = 5 h]. Copyright © 2013 SciRes. JWARP
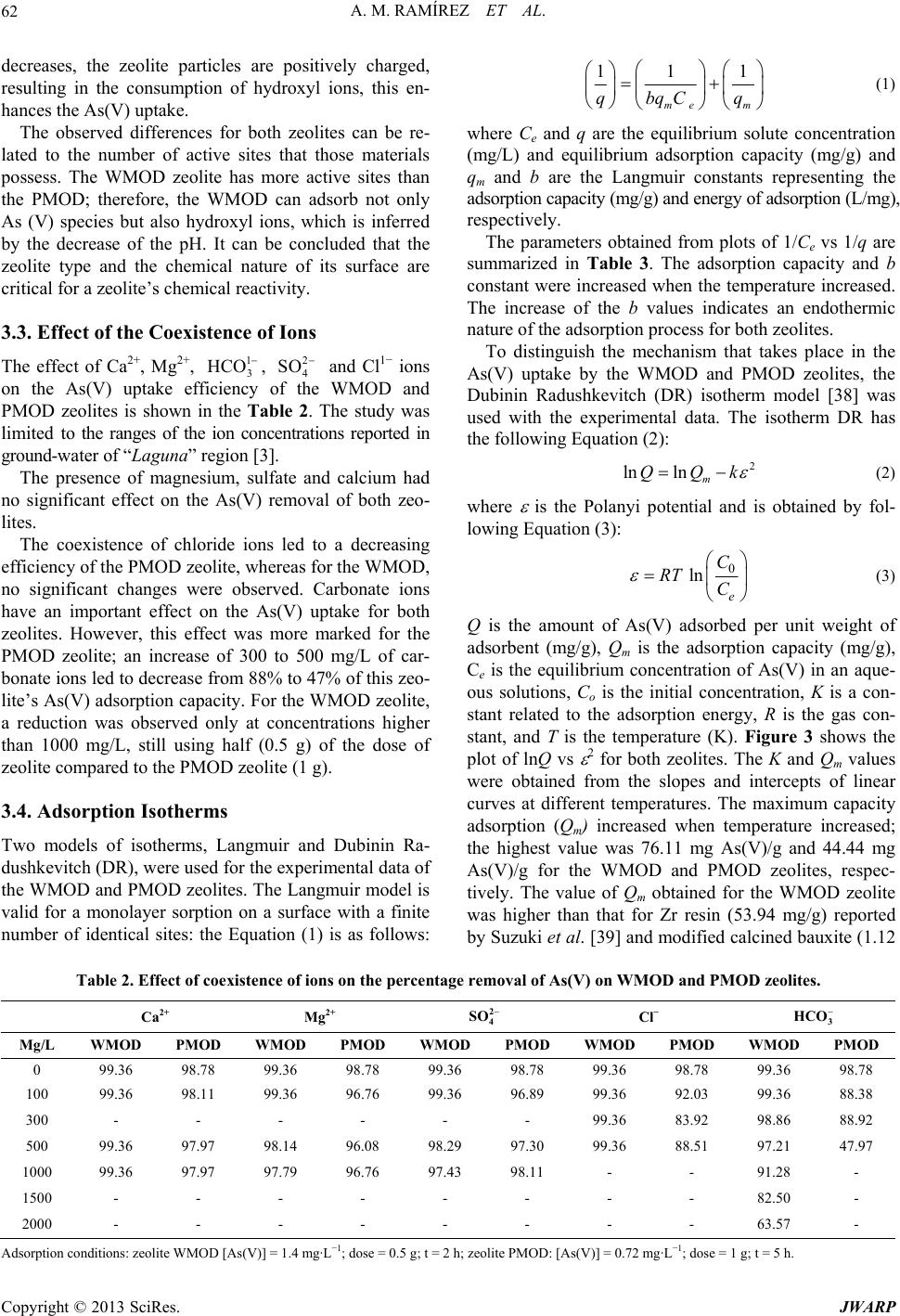 A. M. RAMÍREZ ET AL. Copyright © 2013 SciRes. JWARP 62 1 HCO 2 SO decreases, the zeolite particles are positively charged, resulting in the consumption of hydroxyl ions, this en- hances the As(V) uptake. The observed differences for both zeolites can be re- lated to the number of active sites that those materials possess. The WMOD zeolite has more active sites than the PMOD; therefore, the WMOD can adsorb not only As (V) species but also hydroxyl ions, which is inferred by the decrease of the pH. It can be concluded that the zeolite type and the chemical nature of its surface are critical for a zeolite’s chemical reactivity. 3.3. Effect of the Coexistence of Ions The effect of Ca2+, Mg2+, 3, 4 and Cl1− ions on the As(V) uptake efficiency of the WMOD and PMOD zeolites is shown in the Table 2. The study was limited to the ranges of the ion concentrations reported in ground-water of “Lag una” region [3]. The presence of magnesium, sulfate and calcium had no significant effect on the As(V) removal of both zeo- lites. The coexistence of chloride ions led to a decreasing efficiency of the PMOD zeolite, whereas for the WMOD, no significant changes were observed. Carbonate ions have an important effect on the As(V) uptake for both zeolites. However, this effect was more marked for the PMOD zeolite; an increase of 300 to 500 mg/L of car- bonate ions led to decrease from 88% to 47% of this zeo- lite’s As(V) adsorption capacity. For the WMOD zeolite, a reduction was observed only at concentrations higher than 1000 mg/L, still using half (0.5 g) of the dose of zeolite compared to the PMOD zeolite (1 g). 3.4. Adsorption Isotherms Two models of isotherms, Langmuir and Dubinin Ra- dushkevitch (DR), were used for the experimental data of the WMOD and PMOD zeolites. The Langmuir model is valid for a monolayer sorption on a surface with a finite number of identical sites: the Equation (1) is as follows: 11 1 me m qbqC q 2 lnln m QQk (1) where Ce and q are the equilibrium solute concentration (mg/L) and equilibrium adsorption capacity (mg/g) and qm and b are the Langmuir constants representing the adsorption capacity (mg/g) and energy of adsorption (L/mg), respectively. The parameters obtained from plots of 1/Ce vs 1/q are summarized in Table 3. The adsorption capacity and b constant were increased when the temperature increased. The increase of the b values indicates an endothermic nature of the adsorption process for both zeolites. To distinguish the mechanism that takes place in the As(V) uptake by the WMOD and PMOD zeolites, the Dubinin Radushkevitch (DR) isotherm model [38] was used with the experimental data. The isotherm DR has the following Equation (2): (2) where is the Polanyi potential and is obtained by fol- lowing Equation (3): 0 ln e C RT C (3) Q is the amount of As(V) adsorbed per unit weight of adsorbent (mg/g), Qm is the adsorption capacity (mg/g), Ce is the equilibrium concentration of As(V) in an aque- ous solutions, Co is the initial concentration, K is a con- stant related to the adsorption energy, R is the gas con- stant, and T is the temperature (K). Figure 3 shows the plot of lnQ vs 2 for both zeolites. The K and Qm values were obtained from the slopes and intercepts of linear curves at different temperatures. The maximum capacity adsorption (Qm) increased when temperature increased; the highest value was 76.11 mg As(V)/g and 44.44 mg As(V)/g for the WMOD and PMOD zeolites, respec- tively. The value of Qm obtained for the WMOD zeolite was higher than that for Zr resin (53.94 mg/g) reported by Suzuki et al. [39] and modified calcined bauxite (1.12 Table 2. Effect of coexistence of ions on the percentage removal of As(V) on WMOD and PMOD zeolites. 2 4 SO Cl− Ca2+ Mg2+ 3 HCO Mg/L WMOD PMOD WMOD PMOD WMOD PMOD WMOD PMOD WMOD PMOD 0 99.36 98.78 99.36 98.78 99.36 98.78 99.36 98.78 99.36 98.78 100 99.36 98.11 99.36 96.76 99.36 96.89 99.36 92.03 99.36 88.38 300 - - - - - - 99.36 83.92 98.86 88.92 500 99.36 97.97 98.14 96.08 98.29 97.30 99.36 88.51 97.21 47.97 1000 99.36 97.97 97.79 96.76 97.43 98.11 - - 91.28 - 1500 - - - - - - - - 82.50 - 2000 - - - - - - - - 63.57 - A dsorption conditions: zeolite WMOD [As(V)] = 1.4 mg·L−1; dose = 0.5 g; t = 2 h; zeolite PMOD: [As(V)] = 0.72 mg·L−1; dose = 1 g; t = 5 h.
 A. M. RAMÍREZ ET AL. 63 Table 3. Langmuir parameters for As(V) adsorption on WMOD and PMOD zeolites. WMOD Zeolite PMOD Zeolite T (˚C) qm (mg·g−1) b R2 qm (mg·g−1) b R2 15 0.5906 62.7037 0.93830.5763 5.46030.9604 25 0.4385 162.8571 0.91960.6337 12.72580.9801 35 0.6747 247.00 0.96210.6729 13.75920.9601 Figure 3. DR isotherms for As(V) adsorption on the (a) WMOD and (b) PMOD zeolites [Co = 0.72 mg·L−1; pHo = 7; dose = 0.05 - 1.2 g; t = 18 h]. mg/g) [40]. To evaluate the nature of the interaction between As(V) and the binding sites, the mean energy of adsorption per mole of the sorbate (E) was calculated by following Equa- tion (4): 12 2Ek (4) where E is the free energy change when one mole of ion is transferred from an infinite solution to the surface of a solid. If this magnitude is between 8 and 16 kJ/mol, the adsorption process proceeds by ion exchange, whereas for values of E 8 kJ/mol, the adsorption process is of a physical nature [39]. For both zeolites, at the three tem- peratures studied, E was higher than 8 kJ/mol (Table 4). Therefore, the As(V) uptake on the WMOD and PMOD zeolites occurs by an ionic exchange. The efficiency of the adsorption process was determined by a dimension- less equilibrium parameter r by the next Equation (5): 0 1 1 rbC (5) where b is the Langmuir constant and C0 is the initial concentration of As(V) in mg/L. A value < 1 indicates a favorable adsorption, whereas a value > 1 indicates an unfavorable adsorption. For both zeolites, the r values were lower than 1, which means the adsorption of As(V) is favorable. In addition to the isothermal adsorption, thermody- namic parameters such as the standard Gibb’s free en- ergy (G°), the enthalpy change (H°) and the entropy change (S°) were calculated to evaluate the feasibility and nature of the adsorption process. According to the Langmuir model, adsorption occurs on active sites with the same energy level. The adsorp- tion enthalpy and the corresponding Langmuir constant b depend on the specific pair of adsorbent ions on the ac- tive site and on the temperature [41]. As(V) adsorption is a spontaneous process, the value of the Langmuir con- stant for adsorption reactions can be expressed according with the Equation (6): 0 G bK RT (6) The Gibb’s free energy change is related to the en- thalpy change and the entropy change at a constant tem- perature by the following Equation (7): 0 ln SH KRRT AlOH (7) The thermodynamic parameters are shown in Table 5. For both the WMOD and PMOD zeolites, the enthalpy change was positive, which indicates that the process is endothermic. Negative values of G° confirm the feasi- bility of the process and its spontaneous nature. When As(V) ions react with an 2 ligand on the sorbent surface, water molecules previously bonded to the metal ion are released and dispersed in the solution, resulting in an increase in the entropy [42]. The positive change in the enthalpy and the entropy has been reported for the sorption of several heavy metals onto various low-cost adsorbents such as kaolinite [43] and activated carbon [44]. 3.5. Kinetic Studies The initial concentration of As(V) was varied to deter- mine the kinetic parameters such as the adsorption rate constant (k2) and the mass transfer coefficient ( ) for As(V) adsorption on the WMOD and PMOD zeolites. The Ho’s pseudo-second order kinetic model was used for the experimental data of both zeolites. According the following linearized Equation (8) [45]. 22 2t ttt qkqq (8) where k2 is the rate constant of the pseudo-second order adsorption (g·mg−1·min−1). The values of the parameters of the pseudo-second order model were calculated from plots of t/qt against t at different As(V) concentrations. These parameters are shown in the Table 6. In all cases, the WMOD zeolite showed a higher ad- sorption capacity and rate than the PMOD zeolite, even at the highest concentration evaluated. The WMOD zeo- lite was able to diminish the As(V) concentration to lev- els lower than 0.01 mg/L. The mass transfer coefficient was calculated according to Equation (9) [46]: Copyright © 2013 SciRes. JWARP
 A. M. RAMÍREZ ET AL. 64 Table 4. DR isotherm parameters for As(V) adsorption on WMOD and PMOD zeolites. K × 103 (kJ2·mol−2) Qmax (mg·g−1) r E (kJ·mol−1) Process type R2 T(˚C) WMOD PMOD WMOD PMOD WMOD PMOD WMOD PMOD WMOD PMOD WMOD PMOD 15 −3.616 −3.84 30.48 14.90 0.021 0.120 11.76 11.41 Ionic exchange 0.90940.9557 25 −3.518 −4.21 57.12 43.87 0.080 0.098 11.92 10.90 Ionic exchange 0.94390.9586 35 −3.056 −3.82 76.11 44.44 0.005 0.091 12.79 11.43 Ionic exchange 0.94460.9585 Table 5. Thermodynamic parameters of As(V) adsorption on WMOD and PMOD zeolites. T (˚C) KL × 10−5 (L·mol−1) G° (kJ·mol−1) H° (kJ·mol−1) S° (kJ·mol−1·K−1) WMOD PMOD WMOD PMOD WMOD PMOD WMOD PMOD 15 4.698 0.760 −36.785 −32.424 +50.757 + 11.282 +0.305 +0.152 25 12.201 0.953 −40.427 −34.111 35 18.506 1.039 −42.850 −35.455 Table 6. Parameters of pseudo-second order model for As(V) adsorption on both zeolites. WMOD Zeolite PMOD Zeolite As(V) (mg·L−1) Dose (g) k2 (g·mg−1·min−1) q2 (mg·g−1) R2 Dose (g)k2 (g·mg−1·min −1) q2 (mg·g−1) R2 8.5 1 5.4975 0.8446 0.9999 1 1.0408 0.8347 0.9999 1.45 1 54.572 0.1593 0.9999 1 14.7158 0.1430 0.9999 0.72 1 206.4923 0.0733 0.9999 1 3.5646 0.0687 0.9999 0.45 0.05 0.8646 0.8591 0.9999 0.05 61.2817 0.0192 0.9999 0.10 0.01 0.8441 0.9487 0.9999 0.03 26.7142 0.0073 0.9999 1bp 1 ln ln 11 bp t obp bp C CM K MK bp MKMK St MK (9) where Co is the initial solute concentration (mg/L), Ct is the solute concentration after time t (mg/L), Kbq is the constant obtained by multiplying the Langmuir constants qm and b (L/g), M is the mass of the adsorbent per unit volume of particle free adsorbate solution (g/L), Ss is the external surface area of the adsorbent per unit volume of particle free slurry (cm−1), and the mass transfer coeffi- cient (cm/s). Plots of lnCt/Co − [1/(1+MKbq)] vs t are shown in Figure 4. The values were obtained from the slope and were 9.06 × 10−3 cm/s and 8.86 × 10−3 cm/s for the WMOD and PMOD zeolites, respectively. The mass transfer co- efficients for both zeolites were higher than those for activated alumina (7.2 × 10−3 cm/s) reported by Sar- vinder and Pant [47]. 3.6. Regeneration of Exhausted WMOD Zeolite The WMOD zeolite showed potential for better per- formance on As(V) adsorption than the PMOD zeolite. The WMOD zeolite was accelerated aged at the point that surface of the zeolite was saturated with As(V) spe- cies and the removal was not enough to reach the con- Figure 4. Estimation of the mass transfer coefficient for the adsorption of As(V) on the WMOD and PMOD zeolites [Co = 0.72 mg/L; pH = 7; dosage zeolite = 1 g]. centration required in the effluent. The WMOD was re- generated and the resultant material was retested to evaluate its regeneration capacity. The WMOD zeolite was exhausted with As(V) using different high concen- trations; zeolite was not substituted in any cycle. Figure 5 shows the As(V) removal of the fresh, exhausted and Copyright © 2013 SciRes. JWARP
 A. M. RAMÍREZ ET AL. 65 Figure 5. As(V) removal on the fresh, exhausted and regenerated WMOD zeolites. regenerated zeolites. The WMOD zeolite was able to re- move As(V) to concentrations lower than 0.01 mg/L from a solution of 8 mg/L. This behavior was observed during three cycles; after the fourth cycle, the adsorption capacity decreased pro- gressively. In the seventh cycle, the WMOD zeolite only removed 15% of the As(V). The total As(V) adsorbed was 3.94 mg/g of zeolite. Similar results were obtained when a concentration of 35.9 mg/L was used. In this case, the zeolite adsorbed 9.56 mg of As(V)/g of zeolite. In both experiments, there was no leaching of aluminum from the zeolitic material to the solution. An additional experiment was performed with an As(V) solution of 359 mg/L; after 48 h, the enhanced zeolite adsorbed 78.22%, that is, 28.08 mg As(V)/g of zeolite. After this experiment, aluminum was detected in the re- sidual solution (0.91 mg/L), indicating that aluminum was released from the zeolite to the solution. The As(V) of the exhausted materials was desorbed using a NaOH solution. For the zeolites exhausted with solutions of 8 mg/L and 35.9 mg/L, the As(V) desorbed was 41% and 57%, respectively. For the zeolite exhausted with 359 mg/L, only 14% of the As(V) was desorbed. The desorbed As(V) could be recovered in controlled conditions as a salt and could be evaluated the possibility of application in the electronic, textile and glass indus- tries. During the desorption trials, aluminum was also released. Accordingly, the zeolites were retreated with an aluminum solution as described in Section 2.4. All of the regenerated zeolites exhibited the same As (V) adsorption capacity compared with the original func- tionalized zeolite. These results indicated that the WMOD zeolite has a high adsorption capacity for As(V) removal from water solutions model. WMOD zeolite is easily re- generable and could be a good low-cost material for drinking water and wastewater treatment. 4. Conclusions WMOD is a new material prepared by two-step simple process. In the first step, the fly ash is directly trans- formed with excellent yield (100%) to W zeolite by using one of the lowest amounts of KOH per gram of fly ash (0.33) and soft conditions (175˚C, 16 h). In a second step, the W zeolite surface is functional- ized by ionic exchange with Al (III) (WMOD) to pro- mote As(V) adsorption capacity. According with our findings, WMOD zeolite is che- mical and mineralogically stable in the pH range 3 - 10. It is able to remove As(V) anions from concentrated aqueous solutions (8 mg/L) to meet the value recom- mended by the WHO for drinking water (0.01 mg/L). The presence of carbonates ions only affected the zeo- lite’s performance when the ions’ concentration was high- er than 500 mg/L. One major advantage of the WMOD zeolite is that the spent WMOD zeolite can be regener- ated by adding fresh aluminum salt and its high As(V) adsorption capacity can be recovered. The thermodynamic and kinetic studies of As(V) on PMOD and WMOD are fundamental in the technological development to establish an innovative process to treat drinking water. The final results could contribute in an important way to find an useful low-cost process to re- move As(V) from the contaminated groundwater from arid and semi-arid regions in Mexico and the world. 5. Acknowledgements This research was funded by Sener-Conacyt-Hidrocar- buros 144453 project, FOMIX-COAH 62158 and FOMIX- COAH-C14-149610 projects, Mexico. A. Medina acknowledges M. S. García-Guillermo for her contribution to this work and to CONACyT, Mexico, for the scholarship provided. Copyright © 2013 SciRes. JWARP
 A. M. RAMÍREZ ET AL. 66 REFERENCES [1] C. Perego, R. Bagatin, M. Tagliabue and R. Vignola, “Zeo- lites and Related Mesoporous Materials for Multi-Tal- ented Environmental Solutions,” Microporous and Meso- porous Materials, Vol. 166, No. 1, 2013, pp. 37-49. doi:10.1016/j.micromeso.2012.04.048 [2] Comisión Nacional del Agua, “Estadísticas del Agua en México,” 2013. http://www.conagua.gob.mx/CONAGUA07/Noticias/EA M2010.pdf [3] M. A. Armienta and N. Segovia, “Arsenic and Fluoride in the Groundwater of Mexico,” Environmental Geochemis- try and Health, Vol. 30, No. 4, 2008, pp. 345-353. doi:10.1007/s10653-008-9167-8 [4] M. M. Meza, M. J. Kopplin, J. L. Burgess and A. J. Gan- dolfi, “Arsenic Drinking Water Exposure and Urinary Excretion among Adults in the Yaqui Valley, Sonora, Mexico,” Environmental Research, Vol. 96, No. 2, 2004, pp. 119-126. doi:10.1016/j.envres.2003.08.010 [5] M. F. Díaz, “Arsenic in Scales of Drinking Water Distri- bution Networks in the North of Mexico and Its Detach- ment,” 2013. http://www.agualatinoamerica.com/docs/pdf/030405%20 Nivel%203.pdf [6] T. Yoshida, H. Yamauchi and G. Fan-Sun, “Chronic Health Effects in People Exposed to Arsenic via the Drinking Water: Dose-Response Relationships in Review,” Toxi- cology Applied Pharmacology, Vol. 198, No. 3, 2004, pp. 243-252. doi:10.1016/j.taap.2003.10.022 [7] H. K. Hansen, P. Nuñez and R. Grandon, “Electrocoagu- lation as a Remediation Tool for Wastewaters Containing Arsenic,” Minerals Engineering, Vol. 19, No. 5, 2006, pp. 521-524. doi:10.1016/j.mineng.2005.09.048 [8] I. Peleanu, M. Zaharescu, I. Rau, M. Crisan, A. Jitianu and A. Meghea, “Nanocomposite Materials for As(V) Removal by Magnetically Intensified Adsorption,” Separation Science Technology, Vol. 37, No. 16, 2002, pp. 3693-3701. doi:10.1081/SS-120014827 [9] T. S. Anirudhan and M. R. Unnithan, “Arsenic(V) Re- moval from Aqueous Solutions Using an Anion Ex- changer Derived from Coconut Coir Pith and Its Recov- ery,” Chemosphere, Vol. 66, No. 1, 2007, pp. 60-66. [10] T. F. Lin, C. C. Liu and W. H. Hsieh, “Adsorption Kinet- Ics and Equilibrium of Arsenic onto an Iron-Based Ad- sorbent and an Ion Exchange Resin,” Water Science Tech- nology Water Supply, Vol. 6, No. 2, 2006, pp. 201-207. [11] A. E. Pagana, S. D. Sklari, E. S. Kikkinides and V. T. Zaspalis, “Microporous Ceramic Membrane Technology for the Removal of Arsenic and Chromium Ions from Contaminated Water,” Microporous and Mesoporous Ma- terials, Vol. 110, No. 1, 2008, pp. 150-156. doi:10.1016/j.micromeso.2007.10.013 [12] C. L. Chuang, M. Fan, M. Xu, R. C. Brown, S. Sung, B. Saha and C. P. Huang, “Adsorption of Arsenic(V) by Ac- tivated Carbon Prepared from Oat Hulls,” Chemosphere, Vol. 61, No. 4, 2005, pp. 478-483. doi:10.1016/j.chemosphere.2005.03.012 [13] S. Bang, M. Patel, L. Lippincott and X. Meng, “Removal of Arsenic from Groundwater by Granular Titanium Di- oxide Adsorbent,” Chemosphere, Vol. 60, No. 3, 2005, pp. 389-397. doi:10.1016/j.chemosphere.2004.12.008 [14] M. P. Elizalde-González, J. Mattusch and R. Wennrich, “Application of Natural Zeolites for Preconcentration of Arsenic Species in Water Samples,” Journal Environ- mental Monitoring, Vol. 3, No. 1, 2001, pp. 22-26. doi:10.1039/b006636m [15] C. Y. Chen, T. H. Chang, J. T. Kuo, Y. F. Chen and Y. C. Chung, “Characteristics of Molybdate-Impregnated Chi- tosan Beads (MICB) in Terms of Arsenic Removal from Water and the Application of a MICB-Packed Column to Remove Arsenic from Wastewater,” Bioresource Tech- nology, Vol. 99, No. 16, 2008, pp. 7487-7494. doi:10.1016/j.biortech.2008.02.015 [16] H. K. Hansen, A. Ribeiro and E. Mateus, “Biosorption of Arsenic(V) with Lessonia Nigrescens,” Minerals Engi- neering, Vol. 19, No. 5, 2006, pp. 486-490. doi:10.1016/j.mineng.2005.08.018 [17] S. Kuriakose, T. S. Singh and K. K. Pant, “Adsorption of As(III) from Aqueous Solution onto Iron Oxide Impreg- nated Activated Alumina,” Water Quality Research, Jour- nal of Canada, Vol. 39, No. 3, 2004, pp. 258-266. http://www.cawq.ca/cgi-bin/journal/abstract.cgi?language =english&pk_article=27 [18] S. Kundu, S. S. Kavalakatt, A. Pal, S. K. Ghosh, M. Man- dal and T. Pal, “Removal of Arsenic Using Hardened Paste of Portland Cement: Batch Adsorption and Column Study,” Water Research , Vol. 38, No. 17, 2004, pp. 3780- 3790. doi:10.1016/j.watres.2004.06.018 [19] O. Eljamal, K. Sasaki and T. Hirajima, “Sorption Kinetic of Arsenate as Water Contaminant on Zero Valent Iron,” Journal of Water Resource and Protection, Vol. 5, No. 6, 2013, pp. 563-567. doi:10.4236/jwarp.2013.56057 [20] S. Shevade and R. G. Ford, “Use of Synthetic Zeolites for Arsenate Removal from Pollutant Water,” Water Re- search, Vol. 38, No. 14-15, 2004, pp. 3197-3204. doi:10.1016/j.watres.2004.04.026 [21] C. Resmini, H. Gracher, N. Cabral, E. Angioletto, A. Resmini, A. M. Bernardin, M. R. da Rocha and L. da Silva, “Synthesis of 4A Zeolites from Kaolin for Obtain- ing 5A Zeolites through Ionic Exchange for Adsorption of Arsenic,” Materials Science and Engineering: B, Vol. 177, No. 4, 2012, pp. 345-349. doi:10.1016/j.mseb.2012.01.015 [22] A. M. Ziyath, P. Mahbub, A. Goonetilleke, M. O. Ade- bajo, S. Kokot and A. Oloyede, “Influence of Physical and Chemical Parameters on the Treatment of Heavy Metals in Polluted Stormwater Using Zeolite—A Re- view,” Journal of Water Resource and Protection, Vol. 3, No. 20, 2011, pp. 758-767. doi:10.4236/jwarp.2011.310086 [23] A. Medina, P. Gamero, J. M. Almanza, A. Vargas, A. Montoya, G. Vargas and M. Izquierdo, “Fly Ash from a Mexican Mineral Coal II. Source of W Zeolite and Its Effectiveness in Arsenic (V) Adsorption,” Journal Haz- ardous Materials, Vol. 181, No. 1-3, 2010, pp. 91-104. doi:10.1016/j.jhazmat.2010.04.102 [24] X. Querol, N. Moreno, J. C. Umaña, A. Alastuey, E. Her- Copyright © 2013 SciRes. JWARP
 A. M. RAMÍREZ ET AL. Copyright © 2013 SciRes. JWARP 67 nandez, A. Lopez-Soler and F. Plana, “Synthesis of Zeo- lites from Coal Fly Ash: An Overview,” International Journal of Coal Geology, Vol. 50, No. 1-4, 2002, pp. 413-423. doi:10.1016/S0166-5162(02)00124-6 [25] J. Li, Z. Zhang, W. Khunjar and K. Zhao, “Enhanced Nu- trient Sequestration from Swine Wastewater Using Zeo- lite Synthesized from Fly Ash Integrated with Surface Amendment Technique,” Fuel, Vol. 111, No. 20, 2013, pp. 57-65. doi:10.1016/j.fuel.2013.04.019 [26] N. M. Musyoka, L. Petrik and E. Hums, “Synthesis of Zeolite A, X and P from a South African Coal Fly Ash,” Advanced Materials Research, Vol. 512-515, 2012, pp. 1757-1762. doi:10.4028/www.scientific.net/AMR.512-515.1757 [27] M. S. Onyango, Y. Kojima, O. Aoyi, E. C. Bernardo and H. Matsuda, “Adsorption Equilibrium Modeling and So- lution Chemistry Dependence of Fluoride Removal from Water by Trivalent-Cation-Exchanged Zeolite F-9,” Jour- nal of Colloid and Interface Science, Vol. 279, No. 2, 2004, pp. 341-350. doi:10.1016/j.jcis.2004.06.038 [28] P. Kumar, P. D. Jadhav, S. S. Rayalu and S. Devotta, “Sur- face-Modified-Zeolite a Sequestration of Arsenic and Chro- mium Anions,” Current Science, Vol. 92, No. 4, 2007, pp. 512-517. http://www.currentscience.ac.in/cs/Downloads/article_id_ 092_04_0512_0517_0.pdf [29] S. S. Gupta and K. G. Bhattacharyya, “Kinetics of Ad- Sorption of Metal Ions on Inorganic Materials: A Re- view,” Advances in Colloid and Interface Science, Vol. 162, No. 1-2, 2011, pp. 39-58. doi:10.1016/j.cis.2010.12.004 [30] D. Mohan and C. U. Pittman, “Arsenic Removal from Water/Wastewater Using Adsorbents—A Critical Re- view,” Journal Hazardous Materials, Vol. 142, No. 1-2, 2007, pp. 1-53. doi:10.1016/j.jhazmat.2007.01.006 [31] A. Medina, P. Gamero, X. Querol, N. Moreno, B. de León, M. Almanza, G. Vargas, M. Izquierdo and O. Font, “Fly Ash from a Mexican Mineral Coal I: Mineralogical and Chemical Characterization,” Journal Hazardous Materi- als, Vol. 181, No. 1-3, 2010, pp. 82-90. doi:10.1016/j.jhazmat.2010.04.096 [32] Y. Xu, A. Ohki and S. Maeda, “Adsorption of As(V) by Used Aluminum-Loaded Shirasu Zeolites,” Chemistry Letters, Vol. 27, No. 10, 1998, pp. 1015-1016. doi:10.1246/cl.1998.1015 [33] C. Collela, D. Caputo, B. de Genaro and B. E. Torraca, “Ion Exchange Equilibria in a Synthetic Merlinoite,” Stu- dies and Surface Science and Catalysis, Vol. 154, 2004, pp. 1920-1928. doi:10.1016/S0167-2991(04)80728-6 [34] B. M. Skofteland, O. H. Ellestad and K. P. Lillerud, “Po- tassium Merlinoite Crystallization, Structural and Ther- mal Properties,” Microporous Mesoporous Materials, Vol. 43, No. 1, 2001, pp. 61-71. doi:10.1016/S1387-1811(00)00347-4 [35] A. J. Celestian, J. B. Parise, A. Tripathi, A. Kvick and G. M. V. Vaughan, “(K4Li4)Al8Ge8O32·8H2O: An Li+-Ex- changed Potassium Alumino-Germanate with the Zeolite Gismondine (GIS) Topology,” Acta Crystallographic Sec- tion C, Vol. 59, No. 8, 2003, pp. i74-i76. doi:10.1107/S0108270103011739 [36] A. Tripathi, J. B. Parise, S. J. Kim, Y. Lee, G. M. Johnson and Y. S. Uh, “Structural Changes and Cation Site Or- dering in Na and K Forms of Aluminogermanates with the Zeolite Gismondine Topology,” Chemistry Materials, Vol. 12, No. 12, 2000, pp. 3760-3769. doi:10.1021/cm000459s [37] J. W. Mortier, “Compilation of Extra Framework Sites in Zeolites,” Butterworth Scientific Limited, Surrey, 1982. [38] A. Kilislioglu and B. Bilgin, “Thermodynamic and Ki- netic Investigations of Uranium Adsorption on Amberlite IR-118H Resin,” Applied Radiation and Isotopes, Vol. 58, No. 2, 2003, pp. 155-160. doi:10.1016/S0969-8043(02)00316-0 [39] T. M. Suzuki, J. O. Bomani, H. Matsunaga and T. Yoko- yama, “Preparation of Porous Resin Loaded with Crystal- line Hydrous Zirconium Oxide and Its Application to the Removal of Arsenic,” Reactive and Functional Polymers, Vol. 43, No. 1-2, 2000, pp. 165-172. doi:10.1016/S1381-5148(99)00038-3 [40] P. B. Bhakat, A. K. Gupta, S. Ayoob and S. Kundu, “In- vestigations on Arsenic(V) Removal by Modified Cal- cined Bauxite,” Colloids and Surfaces A: Physicochemi- cal and Engineering Aspects, Vol. 281, No. 1-3, 2006, pp. 237-245. doi:10.1016/j.colsurfa.2006.02.045 [41] F. Di Natale, A. Erto, A. Lancia and D. Musmarra, “Expe- rimental and Modelling Analysis of As(V) Ions Adsorp- tion on Granular Activated Carbon,” Water Research, Vol. 42, No. 8-9, 2007, pp. 2007-2016. doi:10.1016/j.watres.2007.12.008 [42] E. Oguz, “Equilibrium Isotherms and Kinetics Studies for the Sorption of Fluoride on Light Weight Concrete Mate- rials,” Colloids and Surface A. Physicochemical and En- gineering Aspects, Vol. 295, No. 1-3, 2007, pp. 258-263. doi:10.1016/j.colsurfa.2006.09.009 [43] Ö. Yavuz, Y. Altunkaynak and F. Güzel, “Removal of Copper, Nickel, Cobalt and Manganese from Aqueous Solution by Kaolinite,” Water Research, Vol. 37, No. 4, 2003, pp. 948-952. doi:10.1016/S0043-1354(02)00409-8 [44] D. Mohan and K. P. Singh, “Single- and Multi-Compo- nent Adsorption of Cadmium and Zinc Using Activated Carbon Derived from Bagasse—An Agricultural Waste,” Water Research, Vol. 36, No. 9, 2002, pp. 2304-2318. doi:10.1016/S0043-1354(01)00447-X [45] Y. S. Ho and G. A. McKay, “A Comparison of Chemi- sorption Kinetic Models Applied to pollutant Removal on Various Sorbents,” Transactions of the Institution of Che- mical Engineers, Vol. 76, No. 2, 1998, pp. 332-340. doi:10.1205/095758298529696 [46] G. McKay, M. S. Otterburn and A. G. Sweeney, “Surface Mass Transfer Processes during Color Removal from Ef- fluent Using Silica,” Water Research, Vol. 15, No. 3, 1981, pp. 327-331. doi:10.1016/0043-1354(81)90036-1 [47] S. T. Sarvinder and K. K. Pant, “Equilibrium, Kinetics and Thermodynamic Studies for Adsorption of As(III) on Activated Alumina,” Separation Purification Technology, Vol. 36, No. 2, 2004, pp. 139-147. doi:10.1016/S1383-5866(03)00209-0
|