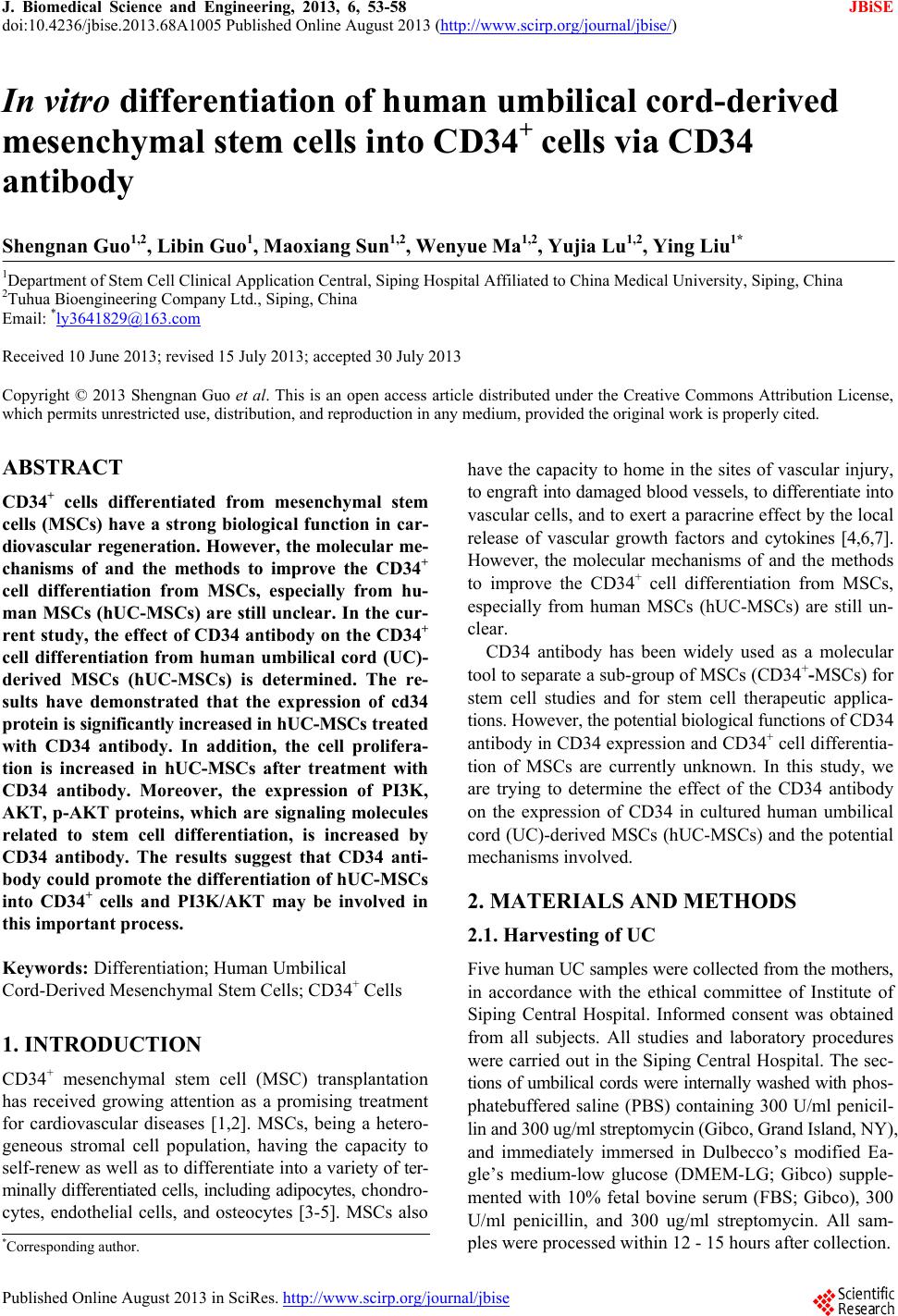 J. Biomedical Science and Engineering, 2013, 6, 53-58 JBiSE doi:10.4236/jbise.2013.68A1005 Published Online August 2013 (http://www.scirp.org/journal/jbise/) In vitro differentiation of human umbilical cord-derived mesenchymal stem cells into CD34+ cells via CD34 antibody Shengnan Guo1,2, Libin Guo1, Maoxiang Sun1,2, Wenyue Ma1,2, Yujia Lu1,2, Ying Liu1* 1Department of Stem Cell Clinical Application Central, Siping Hospital Affiliated to China Medical University, Siping, China 2Tuhua Bioengineering Company Ltd., Siping, China Email: *ly3641829@163.com Received 10 June 2013; revised 15 July 2013; accepted 30 July 2013 Copyright © 2013 Shengnan Guo et al. This is an open access article distributed under the Creative Commons Attribution License, which permits unrestricted use, distribution, and reproduction in any medium, provided the original work is properly cited. ABSTRACT CD34+ cells differentiated from mesenchymal stem cells (MSCs) have a strong biological function in car- diovascular regeneration. However, the molecular me- chanisms of and the methods to improve the CD34+ cell differentiation from MSCs, especially from hu- man MSCs (hUC-MSCs) are still unclear. In the cur- rent study, the effect of CD34 antibody on the CD34+ cell differentiation from human umbilical cord (UC)- derived MSCs (hUC-MSCs) is determined. The re- sults have demonstrated that the expression of cd34 protein is significantly increased in hUC-MSCs t reate d with CD34 antibody. In addition, the cell prolifera- tion is increased in hUC-MSCs after treatment with CD34 antibody. Moreover, the expression of PI3K, AKT, p-AKT proteins, which are signaling molecules related to stem cell differentiation, is increased by CD34 antibody. The results suggest that CD34 anti- body could promote the differentiation of hUC-MSCs into CD34+ cells and PI3K/AKT may be involved in this important process. Keywords: Differentiation; Human Umbilical Cord-Derived Mesenchymal Stem Cells; CD34+ Cells 1. INTRODUCTION CD34+ mesenchymal stem cell (MSC) transplantation has received growing attention as a promising treatment for cardiovascular diseases [1,2]. MSCs, being a hetero- geneous stromal cell population, having the capacity to self-renew as well as to differentiate into a variety of ter- minally differentiated cells, including adipocytes, chondro- cytes, endothelial cells, and osteocytes [3-5]. MSCs also have the capacity to home in the sites of vascular injury, to engraft into damaged blood vessels, to differentiate into vascular cells, and to exert a paracrine effect by the local release of vascular growth factors and cytokines [4,6,7]. However, the molecular mechanisms of and the methods to improve the CD34+ cell differentiation from MSCs, especially from human MSCs (hUC-MSCs) are still un- clear. CD34 antibody has been widely used as a molecular tool to separate a sub-group of MSCs (CD34+-MSCs) for stem cell studies and for stem cell therapeutic applica- tions. However, the potential biological functions of CD34 antibody in CD34 expression and CD34+ cell differentia- tion of MSCs are currently unknown. In this study, we are trying to determine the effect of the CD34 antibody on the expression of CD34 in cultured human umbilical cord (UC)-derived MSCs (hUC-MSCs) and the potential mechanisms involved. 2. MATERIALS AND METHODS 2.1. Harvesting of UC Five human UC samples were collected from the mothers, in accordance with the ethical committee of Institute of Siping Central Hospital. Informed consent was obtained from all subjects. All studies and laboratory procedures were carried out in the Siping Central Hospital. The sec- tions of umbilical cords were internally washed with phos- phatebuffered saline (PBS) containing 300 U/ml penicil- lin and 300 ug/ml streptomycin (Gibco, Grand Island, NY), and immediately immersed in Dulbecco’s modified Ea- gle’s medium-low glucose (DMEM-LG; Gibco) supple- mented with 10% fetal bovine serum (FBS; Gibco), 300 U/ml penicillin, and 300 ug/ml streptomycin. All sam- ples were processed within 12 - 15 hours after collection. *Corresponding author. Published Online August 2013 in SciRes. http://www.scirp.org/journal/jbise
 S. N. Guo et al. / J. Biomedical Science and Engineering 6 (2013) 53-58 54 2.2. Isolation and Culture of Adherent Cells from UC As described in our previous study [8], UCs were filled with 0.1% collagenase (Sigma-Aldrich, St. Louis) in PBS and incubated at 37˚C for 20 min. Each UC was washed with proliferation medium, and the detached cells were harvested after gentle massage of the UC. Cells were centrifuged at 300 g for 10 min, resuspended in prolif- eration medium, and seeded in 75-cm2 flasks at a density of 5 × 107 cells per ml. After 24 h of incubation, non- adherent cells were removed, and culture medium was added with CD34 antibody (0.1 ug/ml) and replaced eve- ry 2 days. Adherent cells were cultured until they reach- ed 80% - 90% confluence. 2.3. Flow Cytometry The hematopoietic lineage marker CD45, human leuco- cyte antigen HLA-DR, the cell-surface markers of endo- thelial progenitor cells CD31 and CD90, were deter- mined by flow cytometry. In this experiment, the adher- ent cells were incubated for 2 h at 37˚C with the rabbit antihuman fluorophore-conjugated primary antibodies (all diluted 1:1000 in 0.01 M PBS; all from Becton Dick- inson, Franklin Lakes, NJ, USA) for direct immunofluo- rescence staining. A total of 10,000 labeled cells were analyzed using a Becton Dickinson LSRFortessaTM Cell Analyzer with the Becton Dickinson FACSDivaTM soft- ware version 6.0 (Becton Dickinson Biosciences, San Jose, CA, USA). 2.4. Cell Proliferation Assay hUC-MSCs were incubated with CD34 antibody (0.01 μmol/l, 0.1 μmol/l or 1 μmol/l) for 96 h. The effects of CD34 antibody on proliferation were assessed by cell counting. hUC-MSCs without CD34 antibody treatment were selected as a control group. Cell counts were ex- pressed as a percentage of the control. 2.5. Immunofluorescence Staining. hUC-MSCs were incubated with CD34 antibody (0.01 μmol/l, 0.1 μmol/l or 1 μmol/l, defined as A, B and C group) for 7 d or 14 d. hUC-MSCs without CD34 antibody treatment were used as a control group. Adherent cells were fixed with 4% cold paraformaldehyde (Sigma) for 15 min and permeabilized with 0.1% Triton X-100 (Sigma) for 10 min. After washing with PBS, cells were blocked with 3% bovine serum albumin (BSA, Sigma) for 30 min, followed by incubating overnight at 4˚C with mouse anti-human primary antibody against CD34 (1:200, Ab- cam), or rabbit anti-human primary antibody against CD105 (1:200, Abcam). The FITC-labeled secondary an- tibody (1:100; goat polyclonal to rabbit IgG; Abcam) and PRITC-labeled secondary antibody (1:100; goat poly- clonal to mouse IgG; Abcam) were then added and in- cubated for 1 h at 37˚C temperature. Cells were washed three times with PBS and counter-stained with DAPI (4’,6-diamidino-2-phenylindole) nuclear dye. The im- munofluorescence staining was observed using Leica DM5000 B fluorescence microscope. 2.6. Western Blot Analysis hUC-MSCs were incubated with CD34 antibody (0.01 μmol/l, 0.1 μmol/l or 1 μmol/l) for 7 d or 14 d. hUC- MSCs without CD34 antibody treatment were used as a control group. Homogenates were prepared from these MSCs using radioimmunoprecipitation assay buffer as described previously [8]. Equal amounts of protein (20 - 40 μg) were added and separated on a 14% Tris-glycine gel, transferred to a polyvinylidene difluoride membrane, and incubated with a PI3K, AKT or a p-AKT primary antibody (Amyjet Scientific Inc, Wuhan, China) at a di- lution of 1:500. The membranes were incubated with an anti-rabbit horseradish peroxidase antibody and develop- ed by enhanced chemiluminescence. The blots were then treated with a protein stripping buffer, reblocked with a blocking agent, and then immunostained with an anti- body against human β-actin (Santa Cruz Biotechnology, Inc., Santa Cruz, CA) as the loading controls. 2.7. Statistical Analysis All data are expressed as mean ± SEM (standard error of the mean). All the experiments were repeated independ- ently at least 3 times. Data were analyzed using analysis of variance followed by Student’s t test when only two groups were studied, taking p < 0.05 as significant. 3. RESULTS 3.1. Isolation and Culture of Adherent Cells from UC Similar to our previous studies [8], primary adherent cul- tures were generated from all UCs samples, with cells displaying a mesenchymal stem cell (MSC)-like phenol- type. After 4 days in culture the cells formed colonies, reaching confluence after 10 - 14 days. Most of the cells were spindle-shaped, resembling fibroblasts. After the second passage, adherent cells constituted homogeneous cell layers with an MSC-like phenotype. The number of adherent cells from the UCs decreased slightly after freez- ing and thawing, and the remaining viable cells were successfully expanded on consecutive days (data not shown). 3.2. Immunophenotype Very few of the adherent cells derived from the UC sam- Copyright © 2013 SciRes. JBiSE
 S. N. Guo et al. / J. Biomedical Science and Engineering 6 (2013) 53-58 55 ples had haematopoietic lineage markers CD45, endothe- lial markers (CD31) and HLA-DR (HLA-class II) on the cell surface, as assessed by flow cytometry. The majority of the cells presented high levels of CD90 protein on the cell surface. In comparison with the fibroblast control, no obvious difference in the levels of surface antigens could be observed, which were similar to our previous studies [8]. 3.3. Cell Proliferation Assay The effect of CD34 antibody on cell proliferation was as- sessed by cell counting. Compare with the control group, hUC-MSCs incubated with CD34 antibody (0.01 μmol/l, 0.1 μmol/l or 1 μmol/l) for 96 h proliferated at 21%, 53%, 28% separately (Table 1). 3.4. Immunohistological Findings To further study the biological characteristics of the dif- ferentiated CD34+ cells from hUC-MSCs, we determined the expression of CD34 and CD105 on the cells’ surface at 7, 14 days after treatment with CD34 antibody (Figure 1). CD34 expression was identified by red fluorescence, whereas CD105 expression was displayed by green fluo- rescence. Many green cells were observed in every time point. The numbers of red cells in these slices were sig- nificantly higher (P < 0.05) than green cells. In addition, as shown in Figures 1(b) and (c), and F-H, we observed small, solitary yellow fluorescence which indicated that cells treated with CD34 antibody could co-express CD34 and CD105 protein. More cells express CD34 protein (red fluorescence) than CD105 protein (green fluores- cence) in C group at 14 d, which suggested the loss of CD105 protein. 3.5. Expression of AKT Protein PI3K, AKT, p-AKT proteins were expressed in the diffe- rentiation process of hUC-MSCs incubated with CD34 antibody (0.01 μmol/l, 0.1 μmol/l or 1 μmol/l) for 7 d and 14 d. Compare with the control group, hUC-MSCs were incubated with CD34 antibody (0.01 μmol/l, 0.1 μmol/l or 1 μmol/l) for 7 d, PI3K protein increased by 7%, 23%, 43% separately; AKT and p-AKT were similar Table 1. hUC-MSCs were incubated with CD34 antibody (0.01 μmol/l, 0.1 μmol/l or 1 μmol/l) for 96 h. The effects of CD34 antibody on proliferation were assessed by cell counting. hUC- MSCs without CD34 antibody treatment were selected as a control group. Cell counts were expressed as a percentage of the control. Compared with the control group, hUC-MSCs were incubated with CD34 antibody (0.1 μmol/l or 1 μmol/l) for 96 h proliferated 48.6%, 28.3% separately. *P < 0.05. Control 0.01 μmol/l 0.1 μmol/l 1 μmol/l 0.45 ± 0.02 0.42 ± 0.01 0.83 ± 0.03* 0.59 ± 0.02 to PI3K protein express (see Figures 2(a) and (b)). At 14 d, the expression of PI3K, AKT, p-AKT proteins was higher than that at 7 d (see Figures 2(a) and (b)). 4. DISCUSSION In the present study, we have demonstrated for the first time that CD34 antibody could promote hUC-MSCs to co-express CD34 and CD105 proteins. While agreeing with the previously report [9,10], during this process, we have observed the cultured cells express CD105 (green staining in Figure 1) at the early phase, and express CD34 (red staining in Figure 1) in the following days. MSCs are a heterogeneous stromal cell population, and cells display some phenotypic and functional characteris- tics of endothelial cells. In our previous study, changes in hUC-MSCs have been detected utilizing multiple cul- ture methods and we have observed the osteodifferention of hUC-MSCs [8]. Changes in hUC-MSCs are coupled with human maladies [11,12]. However, these cells re- quire laborious and time intensive culture techniques. Until now, the differentiation of hUC-MSCs remains un- defined, in part because there is not a consensus defini- tion by cell surface antigen expression, such as CD34 and CD105. CD34 has been used as biomarkers in endo- thelial progenitor cells (EPCs) as well as angiogenesis therapies [13,14]. CD105 and CD34 have been found at the sites of blood vessel formation [15,16]. In this study, the expression of CD34 and CD105 in different phase sug- gests that CD34 antibody could promote the hUC-MSC differentiation into CD34+ cells. Multiple signaling pathways are involved in the dif- ferentiation of MSCs. Akt is known to be part of a pro- survival pathway and the phosphorylation at Thr308 is required for Akt activation [17,18]. In the current study, we have found that PI 3-kinase and phospho-Akt (Thr308) are elevated in CD34 antibody-treated hUC-MSCs com- pared with the control group. However, the effect of CD34 antibody-mediated up-regulation of CD34 expres- sion is reduced when the concentration of the CD34 anti- body reaches at 1 umol/l. The result suggests that maxi- mal effect of CD34 antibody on the CD34 expression may occur at 0.1 umol/l. These observations were com- plied with survival of hUC-MSCs in 96 h at different CD34 antibody concentrations. It is still unclear why Akt (Thr308) is changed, since this would promote but not inhibit apoptosis. However, other phosphorylation sites or downstream effectors may be involved in the prosur- vival and differentiated effect of hUC-MSCs-condition- ed media. Limitations of this current study were: 1) the lack of cell proliferation assay continuous data, which could have confirmed a direct positive effect of CD34 antibody on hUC-MSC differentiation capability; 2) The lack of Copyright © 2013 SciRes. JBiSE
 S. N. Guo et al. / J. Biomedical Science and Engineering 6 (2013) 53-58 Copyright © 2013 SciRes. 56 (a) (b) Figure 1. CD34 and CD105 double positive cells immunofluorescence findings. (a) Immunofluorescence Findings (CD34 protein: red fluorescence; CD105 protein: green fluorescence). A. CD105 protein expression of hUC-MSCs incubated without CD34 antibody at 7 d; B. CD105 protein expression of hUC-MSCs incubated with 0.01 μmol/l CD34 antibody at 7 d; C. CD105 and CD34 protein expression of hUC-MSCs incubated with 0.1 μmol/l CD34 antibody at 7 d; D. CD105 and CD34 protein expression of hUC-MSCs incubated with 1 μmol/l CD34 antibody at 7 d; E. CD105 protein expression of hUC-MSCs incubated without CD34 antibody at 14d; F. CD105 and CD34 protein expression of hUC-MSCs incubated with 0.01 μmol/l CD34 antibody at 14 d; G. CD105 and CD34 pro- tein expression of hUC-MSCs incubated with 0.1 μmol/l CD34 antibody at 14 d; H. CD105 and CD34 protein expression of hUC-MSCs incubated with 1 μmol/l CD34 antibody at 14 d; (b) The percentage of CD34 and CD105 double positive cells analysis. The data were shown as the percentage of CD34 and CD105 double positive cells of the total cells. telomerase activity analysis, which could have confirmed maturation of cells; 3) The lack of the necessary func- tional assays to verify these cells function in vessel for- mation in vivo. Further controlled basically experiments will be required to confirm our preliminary findings and to elucidate the full characterization and mechanistic de- termination involved in improving hUC-MSCs differen- tiation, following treated with CD34 antibody. In summary, our work demonstrated a new method to promote hUC-MSC differentiation via CD34 antibody. Administration of CD34 antibody is able to accelerate the differentiation of hUC-MSCs into CD34+ cells and AKT may play a role in this process. These findings re- inforce our previous observation on the importance of JBiSE
 S. N. Guo et al. / J. Biomedical Science and Engineering 6 (2013) 53-58 57 (a1) (a2) (b1) (b2) Figure 2. Expression of protein. (a) Expression of protein. PI3K, AKT, p-AKT protein expressed in the differentiation phase of hUC-MSCs incubated with CD34 antibody (0.01 μmol/l, 0.1 μmol/l or 1 μmol/l) as A, B and C group for 7 d and 14 d separately; con: control group. (a1) Expression of protein in 7 d; (a2) Expression of protein in 14 d. (b) Expression of protein analysis. (b1) Ex- pression of protein analysis in 7 d. Compared with the control group, hUC-MSCs incubated with CD34 antibody (0.01 μmol/l, 0.1 μmol/l or 1 μmol/l) for 7 d, PI3K protein expression increased 7%, 23%, 43% separately; AKT and p-AKT protein expression were similar to PI3K protein express; (b2) Expression of protein analysis in 14 d. Compared with the control group, hUC-MSCs incubated with CD34 antibody (0.01 μmol/l, 0.1 μmol/l or 1 μmol/l) for 14 d, PI3K protein expression increased 24%, 46% in A, and B groups separately; Compared with the B group, PI3K protein expression decreased 26% in C group. *p < 0.05; **p < 0.01; #p < 0.05. hUC-MSCs for research and future therapeutic use. REFERENCES [1] Qiu, X., Sun, C., Yu, W., Lin, H., Sun, Z., Chen, Y., Wang, R. and Dai, Y. (2012) Combined strategy of mesenchy- mal stem cell injection with vascular endothelial growth factor gene therapy for the treatment of diabetes-associ- ated erectile dysfunction. Journal of Andrology, 33, 37- 44. doi:10.2164/jandrol.110.012666 [2] Radhakrishnan, V., Muthurangan, M., May, A.-N., Bala- muthu, K., Abdullah, A., Nehad, M.A. (2012) In vitro differentiation of human skin-derived multipotent stromal cells into putative endothelial-like cells. BMC Develop- mental Biology, 12, 7. doi:10.1186/1471-213X-12-7 [3] Uccelli, A., Moretta, L. and Pistoia, V. (2008) Mesenchy- mal stem cells in health and disease. Nature Reviews Im- munology, 8, 726-736. doi:10.1038/nri2395 [4] Oswald, J., Boxberger, S., Jorgensen, B., Feldmann, S., Ehninger, G., Bornhauser, M. and Werner, C. (2004) Me- senchymal stem cells can be differentiated into endothe- lial cells in vitro. Stem Cells, 22, 377-384. doi:10.1634/stemcells.22-3-377 [5] Pittenger, M.F., Mackay, A.M., Beck, S.C., Jaiswal, R.K., Douglas, R., Mosca, J.D., Moorman, M.A., Simonetti, D.W., Craig, S. and Marshak, D.R. (1999) Multilineage poten- tial of adult human mesenchymal stem cells. Science, 284, 143-147. doi:10.1126/science.284.5411.143 [6] Lian, Q., Zhang, Y., Zhang, J., Zhang, H.K., Wu, X., Lam, F.F., Kang, S., Xia, J.C., Lai, W.H., Au, K.W., Chow, Y.Y., Siu, C.W., Lee, C.N. and Tse, H.F. (2010) Functio- nal mesenchymal stem cells derived from human induced pluripotent stem cells attenuate limb ischemia in mice. Circulation, 121, 1113-1123. doi:10.1161/CIRCULATIONAHA.109.898312 [7] Liu, Z.J., Zhuge, Y. and Velazquez, O.C. (2009) Trafck- ing and differentiation of mesenchymalfi stem cells. Journal of Cellular Biochemistry, 106, 984-991. doi:10.1002/jcb.22091 [8] Qu, Z.G., Guo, L.B., Fang, G.J., Cui, Z.H., Guo, S.N. and Liu, Y. (2012) Biological characteristics and effect of human umbilical cord mesenchymal stem cells (hUC- MSCs) grafting with blood plasma on bone regeneration in rats. Cell Biochemistry and Biophysics, 63, 171-181. doi:10.1007/s12013-012-9354-1 [9] Timmermans, F., Van Hauwermeiren, F., De Smedt, M., Raedt, R., Plasschaert, F., De Buyzere, M.L., Gillebert, T.C., Plum, J. and Vandekerckhove, B. (2007) Endothe- lial outgrowth cells are not derived from CD133+ cells or CD45+ hematopoietic precursors. Arteriosclerosis, Throm- bosis, and Vascular Biology, 7, 1572-1579. doi:10.1161/ATVBAHA.107.144972 [10] Zhang, Y., Fisher, N., Newey, S.E., Smythe, J., Tatton, L., Tsaknakis, G., Forde, S.P., Carpenter, L., Athanassopou- los, T., Hale, S.J., Ferguson, D.J., Tyler, M.P. and Watt, S.M. (2009) The impact of proliferative potential of um- bilical cord-derived endothelial progenitor cells and hy- poxia on vascular tubule formation in vitro. Stem Cells and Development, 18, 359-375. Copyright © 2013 SciRes. JBiSE
 S. N. Guo et al. / J. Biomedical Science and Engineering 6 (2013) 53-58 58 doi:10.1089/scd.2008.0071 [11] Wu, K.H., Sheu, J.N., Wu, H.P., Tsai, C., Sieber, M., Peng, C.T. and Chao, Y.H. (2013) Cotransplantation of umbili- cal cord-derived mesenchymal stem cells promote hema- topoietic engraftment in cord blood transplantation: A pilot study. Transplantation, 95, 773-777. doi:10.1097/TP.0b013e31827a93dd [12] Fan, C.G., Zhang, Q.J. and Zhou, J.R. (2011) Therapeutic potentials of mesenchymal stem cells derived from hu- man umbilical cord. Stem Cells and Development, 7, 195- 207. doi:10.1007/s12015-010-9168-8 [13] Bayat, H., Fathi, F., Peyrovi, H. and Mowla, S.J. (2013) Evaluating the expression of self-renewal genes in human endothelial progenitor cells. Cell Journal, 14, 298-305. [14] Cheng, C.C., Chang, S.J., Chueh, Y.N., Huang, T.S., Huang, P.H., Cheng, S.M., Tsai, T.N., Chen, J.W. and Wang, H.W. (2013) Distinct angiogenesis roles and surface markers of early and late endothelial progenitor cells revealed by functional group analyses. BMC Genomics, 15, 14-182. [15] Stuart, J., Smith, Hanna, T., Jennifer, H.W., Donald, C.M., James, L., Beth, C. and Richard, G.G. (2012) CD105 (En- doglin) exerts prognostic effects via its role in the micro- vascular niche of paediatric high grade glioma. Acta Neu- ropathologica, 124, 99-110. doi:10.1007/s00401-012-0952-1 [16] Jason, D.R., Rajendra, S.-M., Matthew, P.B., Steven, M.J., Lesley, D., Deepak, A.R., Tai, Y., Tamar, L.M., Ani, N., Brooks, U., Narutoshi, H., Toshiharu, S., Saltzman, W.M., Edward, S., Themis, R.K., Jordan, S.P., Christopher, K.B. (2010) Tissue-engineered vascular grafts transform into mature blood vessels via an inflammation-mediated pro- cess of vascular remodeling. Proceedings of the National Academy of Sciences of the USA, 107, 4669-4674. [17] Bellacosa, A., Kumar, C.C., Di Cristofano, A. and Testa, J.R. (2005) Activation of AKT kinases in cancer: Implica- tions for therapeutic targeting. Advanced Cancer Resear- ch, 94, 29-86. doi:10.1016/S0065-230X(05)94002-5 [18] Boomsma, R.A. and Geenen, D.L. (2012) Mesenchymal stem cells secrete multiple cytokines that promote angio- genesis and have contrasting effects on chemotaxis and apoptosis. PLoS One, 7, e35685. doi:10.1371/journal.pone.0035685 Copyright © 2013 SciRes. JBiSE
|