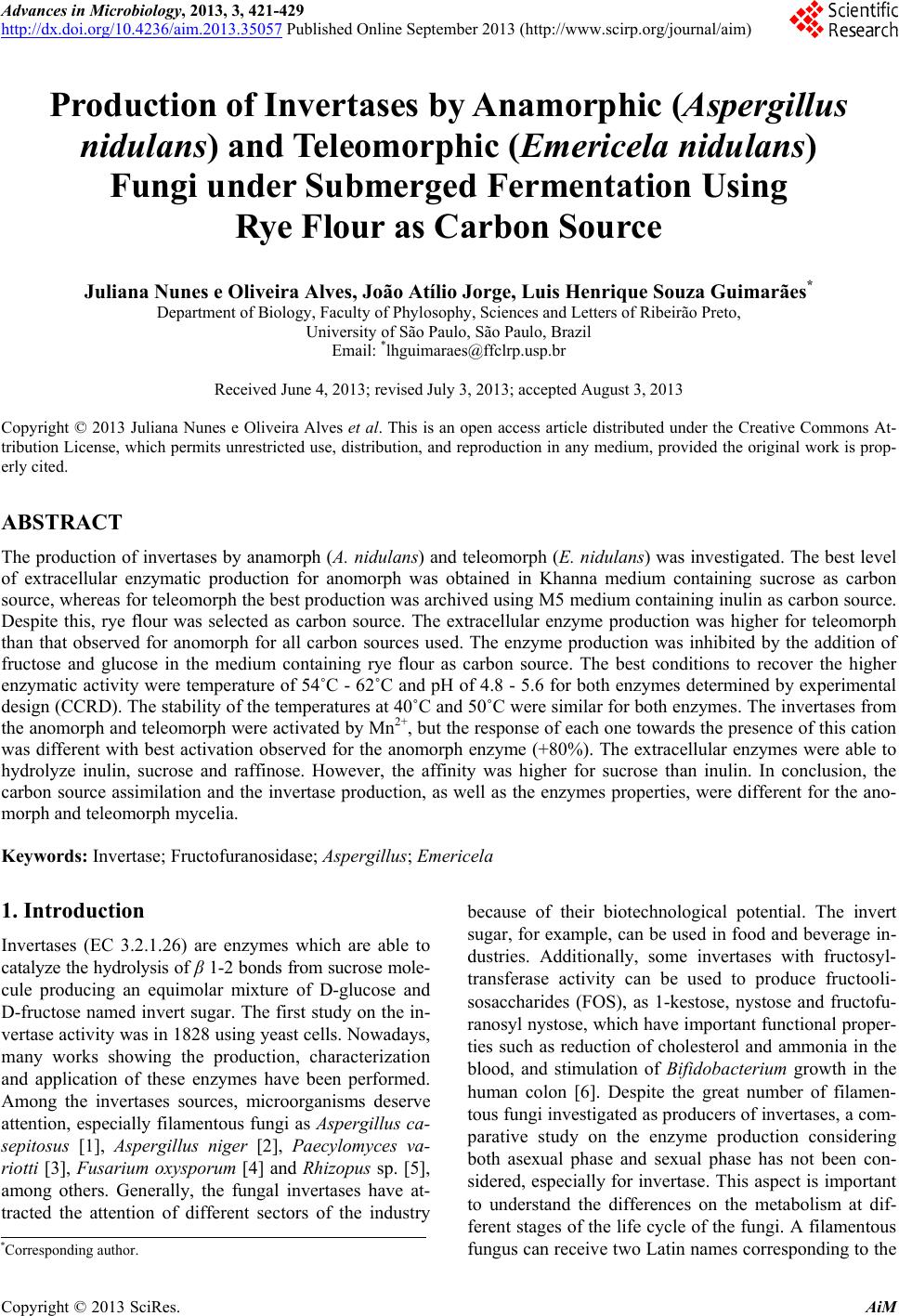 Advances in Microbiology, 2013, 3, 421-429 http://dx.doi.org/10.4236/aim.2013.35057 Published Online September 2013 (http://www.scirp.org/journal/aim) Production of Invertases by Anamorphic (Aspergillus nidulans) and Teleomorphic (Emericela nidulans) Fungi under Submerged Fermentation Using Rye Flour as Carbon Source Juliana Nunes e Oliveira Alves, João Atílio Jorge, Luis Henrique Souza Guimarães* Department of Biology, Faculty of Phylosophy, Sciences and Letters of Ribeirão Preto, University of São Paulo, São Paulo, Brazil Email: *lhguimaraes@ffclrp.usp.br Received June 4, 2013; revised July 3, 2013; accepted August 3, 2013 Copyright © 2013 Juliana Nunes e Oliveira Alves et al. This is an open access article distributed under the Creative Commons At- tribution License, which permits unrestricted use, distribution, and reproduction in any medium, provided the original work is prop- erly cited. ABSTRACT The production of invertases by anamorph (A. nidulans) and teleomorph (E. nidulans) was investigated. The best level of extracellular enzymatic production for anomorph was obtained in Khanna medium containing sucrose as carbon source, whereas for teleomorph the best production was archived using M5 medium containing inulin as carbon source. Despite this, rye flour was selected as carbon source. The extracellular enzyme production was higher for teleomorph than that observed for anomorph for all carbon sources used. The enzyme production was inhibited by the addition of fructose and glucose in the medium containing rye flour as carbon source. The best conditions to recover the higher enzymatic activity were temperature of 54˚C - 62˚C and pH of 4.8 - 5.6 for both enzymes determined by experimental design (CCRD). The stability of the temperatures at 40˚C and 50˚C were similar for both enzymes. The invertases from the anomorph and teleomorph were activated by Mn2+, but the response of each one towards the presence of this cation was different with best activation observed for the anomorph enzyme (+80%). The extracellular enzymes were able to hydrolyze inulin, sucrose and raffinose. However, the affinity was higher for sucrose than inulin. In conclusion, the carbon source assimilation and the invertase production, as well as the enzymes properties, were different for the ano- morph and teleomorph mycelia. Keywords: Invertase; Fructofuranosidase; Aspergillus; Emericela 1. Introduction Invertases (EC 3.2.1.26) are enzymes which are able to catalyze the hydrolysis of β 1-2 bonds from sucrose mole- cule producing an equimolar mixture of D-glucose and D-fructose named invert sugar. The first study on the in- vertase activity was in 1828 using yeast cells. Nowadays, many works showing the production, characterization and application of these enzymes have been performed. Among the invertases sources, microorganisms deserve attention, especially filamentous fungi as Aspergillus ca- sepitosus [1], Aspergillus niger [2], Paecylomyces va- riotti [3], Fusarium oxysporum [4] and Rhizopus sp. [5], among others. Generally, the fungal invertases have at- tracted the attention of different sectors of the industry because of their biotechnological potential. The invert sugar, for example, can be used in food and beverage in- dustries. Additionally, some invertases with fructosyl- transferase activity can be used to produce fructooli- sosaccharides (FOS), as 1-kestose, nystose and fructofu- ranosyl nystose, which have important functional proper- ties such as reduction of cholesterol and ammonia in the blood, and stimulation of Bifidobacterium growth in the human colon [6]. Despite the great number of filamen- tous fungi investigated as producers of invertases, a com- parative study on the enzyme production considering both asexual phase and sexual phase has not been con- sidered, especially for invertase. This aspect is important to understand the differences on the metabolism at dif- ferent stages of the life cycle of the fungi. A filamentous fungus can receive two Latin names corresponding to the *Corresponding author. C opyright © 2013 SciRes. AiM
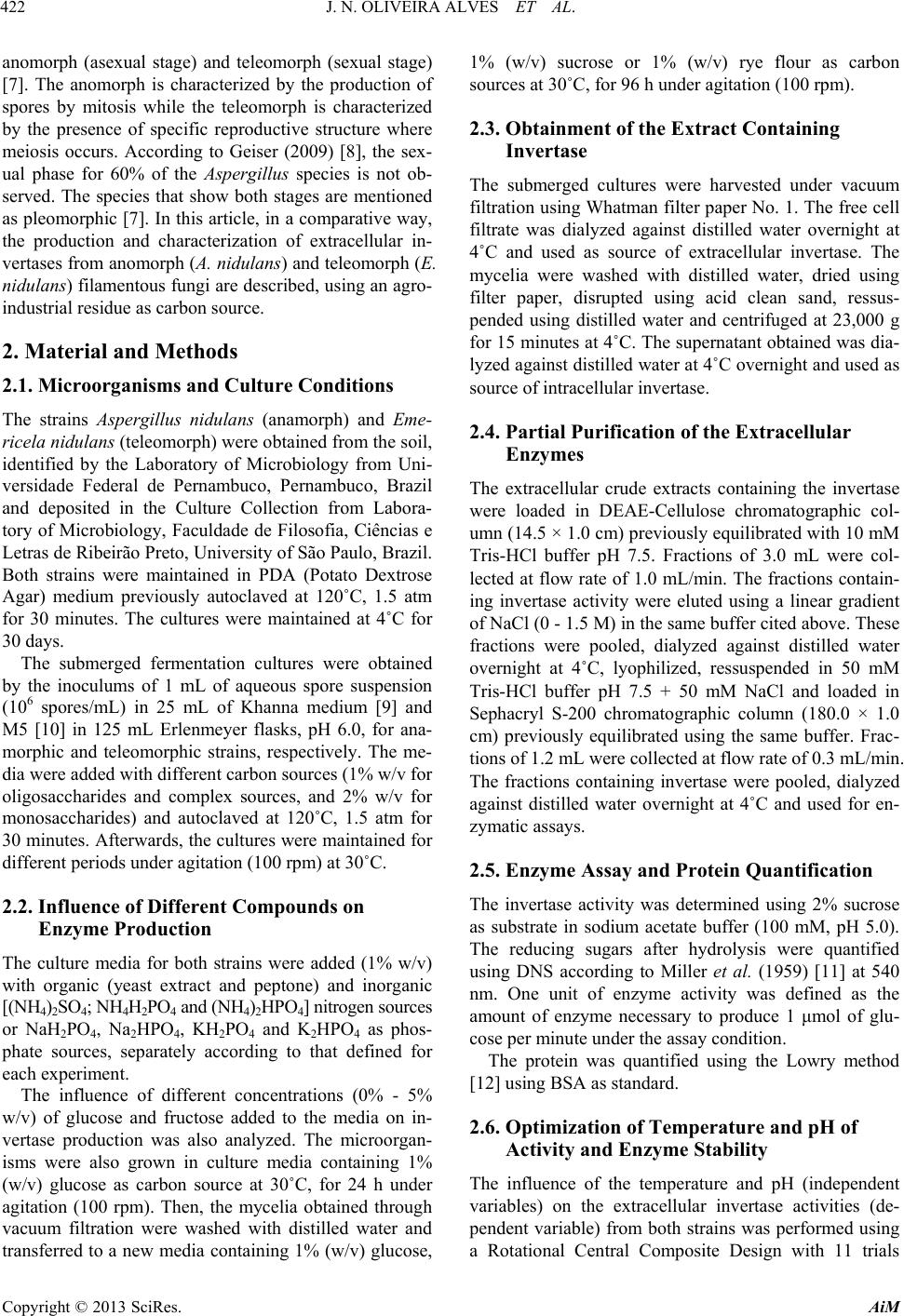 J. N. OLIVEIRA ALVES ET AL. 422 anomorph (asexual stage) and teleomorph (sexual stage) [7]. The anomorph is characterized by the production of spores by mitosis while the teleomorph is characterized by the presence of specific reproductive structure where meiosis occurs. According to Geiser (2009) [8], the sex- ual phase for 60% of the Aspergillus species is not ob- served. The species that show both stages are mentioned as pleomorphic [7]. In this article, in a comparative way, the production and characterization of extracellular in- vertases from anomorph (A. nidulans) and teleomorph (E. nidulans) filamentous fungi are described, using an agro- industrial residue as carbon source. 2. Material and Methods 2.1. Microorganisms and Culture Conditions The strains Aspergillus nidulans (anamorph) and Eme- ricela nidulans (teleomorph) were obtained from the soil, identified by the Laboratory of Microbiology from Uni- versidade Federal de Pernambuco, Pernambuco, Brazil and deposited in the Culture Collection from Labora- tory of Microbiology, Faculdade de Filosofia, Ciências e Letras de Ribeirão Preto, University of São Paulo, Brazil. Both strains were maintained in PDA (Potato Dextrose Agar) medium previously autoclaved at 120˚C, 1.5 atm for 30 minutes. The cultures were maintained at 4˚C for 30 days. The submerged fermentation cultures were obtained by the inoculums of 1 mL of aqueous spore suspension (106 spores/mL) in 25 mL of Khanna medium [9] and M5 [10] in 125 mL Erlenmeyer flasks, pH 6.0, for ana- morphic and teleomorphic strains, respectively. The me- dia were added with different carbon sources (1% w/v for oligosaccharides and complex sources, and 2% w/v for monosaccharides) and autoclaved at 120˚C, 1.5 atm for 30 minutes. Afterwards, the cultures were maintained for different periods under agitation (100 rpm) at 30˚C. 2.2. Influence of Different Compounds on Enzyme Production The culture media for both strains were added (1% w/v) with organic (yeast extract and peptone) and inorganic [(NH4)2SO4; NH4H2PO4 and (NH4)2HPO4] nitrogen sources or NaH2PO4, Na2HPO4, KH2PO4 and K2HPO4 as phos- phate sources, separately according to that defined for each experiment. The influence of different concentrations (0% - 5% w/v) of glucose and fructose added to the media on in- vertase production was also analyzed. The microorgan- isms were also grown in culture media containing 1% (w/v) glucose as carbon source at 30˚C, for 24 h under agitation (100 rpm). Then, the mycelia obtained through vacuum filtration were washed with distilled water and transferred to a new media containing 1% (w/v) glucose, 1% (w/v) sucrose or 1% (w/v) rye flour as carbon sources at 30˚C, for 96 h under agitation (100 rpm). 2.3. Obtainment of the Extract Containing Invertase The submerged cultures were harvested under vacuum filtration using Whatman filter paper No. 1. The free cell filtrate was dialyzed against distilled water overnight at 4˚C and used as source of extracellular invertase. The mycelia were washed with distilled water, dried using filter paper, disrupted using acid clean sand, ressus- pended using distilled water and centrifuged at 23,000 g for 15 minutes at 4˚C. The supernatant obtained was dia- lyzed against distilled water at 4˚C overnight and used as source of intracellular invertase. 2.4. Partial Purification of the Extracellular Enzymes The extracellular crude extracts containing the invertase were loaded in DEAE-Cellulose chromatographic col- umn (14.5 × 1.0 cm) previously equilibrated with 10 mM Tris-HCl buffer pH 7.5. Fractions of 3.0 mL were col- lected at flow rate of 1.0 mL/min. The fractions contain- ing invertase activity were eluted using a linear gradient of NaCl (0 - 1.5 M) in the same buffer cited above. These fractions were pooled, dialyzed against distilled water overnight at 4˚C, lyophilized, ressuspended in 50 mM Tris-HCl buffer pH 7.5 + 50 mM NaCl and loaded in Sephacryl S-200 chromatographic column (180.0 × 1.0 cm) previously equilibrated using the same buffer. Frac- tions of 1.2 mL were collected at flow rate of 0.3 mL/min. The fractions containing invertase were pooled, dialyzed against distilled water overnight at 4˚C and used for en- zymatic assays. 2.5. Enzyme Assay and Protein Quantification The invertase activity was determined using 2% sucrose as substrate in sodium acetate buffer (100 mM, pH 5.0). The reducing sugars after hydrolysis were quantified using DNS according to Miller et al. (1959) [11] at 540 nm. One unit of enzyme activity was defined as the amount of enzyme necessary to produce 1 μmol of glu- cose per minute under the assay condition. The protein was quantified using the Lowry method [12] using BSA as standard. 2.6. Optimization of Temperature and pH of Activity and Enzyme Stability The influence of the temperature and pH (independent variables) on the extracellular invertase activities (de- pendent variable) from both strains was performed using a Rotational Central Composite Design with 11 trials Copyright © 2013 SciRes. AiM
 J. N. OLIVEIRA ALVES ET AL. 423 with 3 repetitions at the central point and 2 axial points. The software Statistica V8.0 (StatSoft, USA) was used to perform the analysis of variance (ANOVA) with 95% of significance level and to generate the surface response plots. The thermo stability was determined by the incubation of the samples of enzymes aqueous solution at different temperatures (40˚C - 70˚C) for 60 minutes and then as- sayed for invertase activity as described above. The pH stability was determined by the incubation of the enzyme samples in different pH values (sodium acetate buffer pH 3.7 - 5.7; Tris-HCl buffer pH 7.0 - 8.0; CAPS buffer pH 9.0 - 10.0) for 60 minutes and then used for invertase assay as described above. 2.7. Influence of Different Compounds on Invertase Activity The influence of 1 mM of different salts and β-mercap- toethanol added to the reaction medium on invertase ac- tivity was analyzed. 2.8. Hydrolysis of Different Substrates and Kinetic Parameters The hydrolytic activity from the extracellular invertases on different substrates was analyzed using 0.5% (w/v) of inulin, rafinose and sucrose in 100 mM sodium acetate buffer pH 5.0. Mixtures (1:1 v/v) of inulin plus rafinose, inulin plus sucrose and sucrose plus rafinose were also analyzed. The kinetic parameters Km and Vmax were de- termined for sucrose as substrate (0.1 - 20 mM) using Lineweaver-Burk plot obtained with Origin Graph soft- ware (OriginLab Corporation). 3. Results and Discussion 3.1. Enzyme Production The Aspergillus genus comprises many fungal species recognized as anamorph, which are classified in different teleomorph genera as, for example, A. nidulans, which is part of the teleomorph genus Emericela [13]. Aspergillus spp. has been mentioned as a good producer of different enzymes that act on polymers to obtain nutrients. In ad- dition, these enzymes, according to their properties, have biotechnological potential for many industrial purposes. Among these enzymes, invertases deserve attention. A. nidulans was selected because it has been mentioned as a model for production of different extracellular enzymes with biotechnological potential [14]. In addition, the teleomorph E. nidulans was observed as an important enzyme producer in our laboratory. So far, the compari- son between invertase production by anamorph and teleomorph filamentous fungi has not been elucidated. The growth of filamentous fungi and, consequently, the enzyme production, are influenced by the nutrients used, including the carbon source. As it can be observed in the Table 1, the highest enzymatic production for both intra and extracellular invertases by E. nidulans was achieved using inulin as substrate. On the other hand, the sucrose was the best substrate for extracellular enzyme production by A. nidulans. The fungi Aspergillus niger and A. nidulans were mentioned as models for enzyme secretion, since invertase production is induced by su- crose [15]. Considering the best conditions obtained for each one, the enzyme production by E. nidulans was around 8-fold higher than that observed for intra and ex- tracellular enzymes from A. nidulans. This fact shows that the carbon source assimilation by filamentous fungi is related to the life cycle period. The extracellular en- zyme production by the teleomorph was higher than that observed for the anamorph for all carbons sources tested. The second best carbon source for both E. nidulans and A. nidulans was rye flour. Considering this agroindustrial product, the extracellular production was around 10-fold higher for E. nidulans if compared to the A. nidulans and similar for the intracellular enzymes. Agroindustrial residues/products are low-cost substrates that can be used to produce enzymes with industrial application. In addi- tion, this type of carbon source is similar to the carbon sources found in the natural environment of the filamen- tous fungi, which are able to hydrolyze different poly- mers. Table 1. Influence of different carbon sources on produc- tion of intra and extracellular invertases by A. nidulans (anamorph) and E. nidulans (telomorph) using Submerged Fermentation. Invertase activity (total U) Teleomorph Anamorph Carbon sources Extra Intra Extra Intra Without 182.9 ± 32.45.4 ± 1.2 7.8 ± 3.4 1.7 ± 0.7 Corncob 188.1 ± 13.017.5 ± 5.8 0.22 ± 0.4 9.0 ± 1.6 Crushed corn106.4 ± 19.050.1 ± 10.8 1.2 ± 0.2 4.8 ± 1.3 Fructose 64.4 ± 7.021.7 ± 3.6 3.5 ± 1.0 26.0 ± 7.3 Glucose 28.3 ± 8.430.1 ± 2.3 0 12.8 ± 0.7 Inulin 714.4 ± 100.3224.4 ± 6.5 11.4 ± 2.6 23.5 ± 4.5 Oat meal 279.8 ± 24.842.5 ± 2.4 19.0 ± 11.4 16.3 ± 4.5 Rice straw204.3 ± 11.92.5 ± 1.5 6.2 ± 1.5 3.1 ± 0.4 Rye flour 361.9 ± 30.628.3 ± 2.3 33.6 ± 8.3 30.0 ± 5.0 Sucrose 244.3 ± 17.030.0 ± 4.1 93.0 ± 12.3 28.3 ± 10.0 Sugar cane bagasse 103.8 ± 5.016.6 ± 0.1 13.7 ± 2.0 31.9 ± 7.3 The fungi A. nidulans and E. nidulans were cultured in Khanna and M5 media, respectively, with initial pH 6.0, maintained at 30˚C, under orbital agitation for 72 h. Copyright © 2013 SciRes. AiM
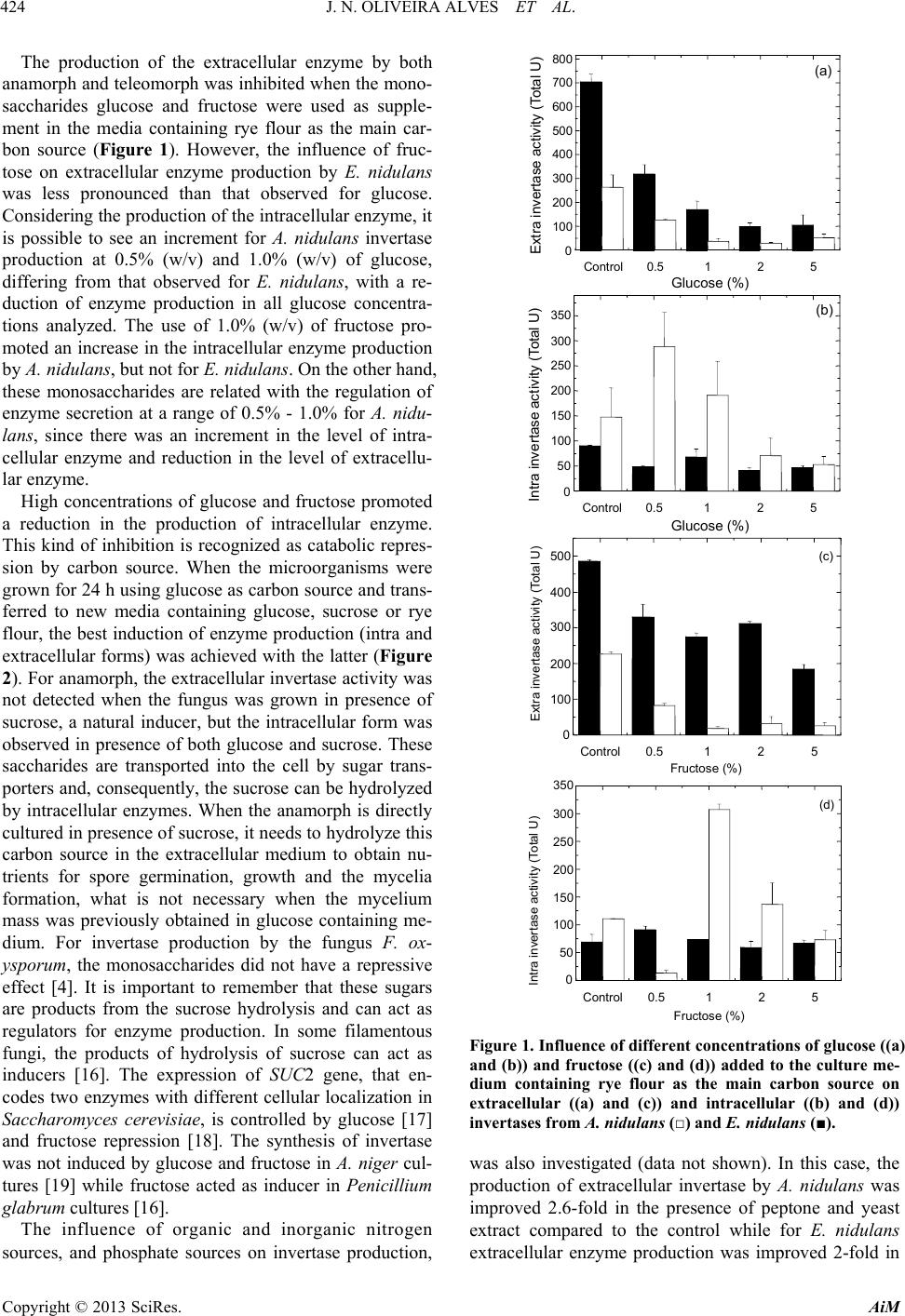 J. N. OLIVEIRA ALVES ET AL. 424 The production of the extracellular enzyme by both anamorph and teleomorph was inhibited when the mono- saccharides glucose and fructose were used as supple- ment in the media containing rye flour as the main car- bon source (Figure 1). However, the influence of fruc- tose on extracellular enzyme production by E. nidulans was less pronounced than that observed for glucose. Considering the production of the intracellular enzyme, it is possible to see an increment for A. nidulans invertase production at 0.5% (w/v) and 1.0% (w/v) of glucose, differing from that observed for E. nidulans, with a re- duction of enzyme production in all glucose concentra- tions analyzed. The use of 1.0% (w/v) of fructose pro- moted an increase in the intracellular enzyme production by A. nidulans, but not for E. nidulans. On the other hand, these monosaccharides are related with the regulation of enzyme secretion at a range of 0.5% - 1.0% for A. nidu- lans, since there was an increment in the level of intra- cellular enzyme and reduction in the level of extracellu- lar enzyme. High concentrations of glucose and fructose promoted a reduction in the production of intracellular enzyme. This kind of inhibition is recognized as catabolic repres- sion by carbon source. When the microorganisms were grown for 24 h using glucose as carbon source and trans- ferred to new media containing glucose, sucrose or rye flour, the best induction of enzyme production (intra and extracellular forms) was achieved with the latter (Figure 2). For anamorph, the extracellular invertase activity was not detected when the fungus was grown in presence of sucrose, a natural inducer, but the intracellular form was observed in presence of both glucose and sucrose. These saccharides are transported into the cell by sugar trans- porters and, consequently, the sucrose can be hydrolyzed by intracellular enzymes. When the anamorph is directly cultured in presence of sucrose, it needs to hydrolyze this carbon source in the extracellular medium to obtain nu- trients for spore germination, growth and the mycelia formation, what is not necessary when the mycelium mass was previously obtained in glucose containing me- dium. For invertase production by the fungus F. ox- ysporum, the monosaccharides did not have a repressive effect [4]. It is important to remember that these sugars are products from the sucrose hydrolysis and can act as regulators for enzyme production. In some filamentous fungi, the products of hydrolysis of sucrose can act as inducers [16]. The expression of SUC2 gene, that en- codes two enzymes with different cellular localization in Saccharomyces cerevisiae, is controlled by glucose [17] and fructose repression [18]. The synthesis of invertase was not induced by glucose and fructose in A. niger cul- tures [19] while fructose acted as inducer in Penicillium glabrum cultures [16]. The influence of organic and inorganic nitrogen sources, and phosphate sources on invertase production, Control 0.5 1 2 5 0 100 200 300 400 500 600 700 800 (a) Extra invertase activity (Total U) Glucose (%) Control 0.5 1 2 5 0 50 100 150 200 250 300 350 (b) Intra invertase activity (Total U) Glucose (%) Control 0.51 2 5 0 100 200 300 400 500 (c) Extra invertase activity (Total U) Fruc tose (% ) Control 0.51 2 5 0 50 100 150 200 250 300 350 (d) Intra invertase activity (Total U) Fructos e (% ) Figure 1. Influence of different concentrations of glucose ((a) and (b)) and fructose ((c) and (d)) added to the culture me- dium containing rye flour as the main carbon source on extracellular ((a) and (c)) and intracellular ((b) and (d)) invertases from A. nidulans (□) and E. nidulans (■). was also investigated (data not shown). In this case, the production of extracellular invertase by A. nidulans was improved 2.6-fold in the presence of peptone and yeast extract compared to the control while for E. nidulans extracellular enzyme production was improved 2-fold in Copyright © 2013 SciRes. AiM
 J. N. OLIVEIRA ALVES ET AL. 425 glucosesucroserye flour 0 50 100 150 200 250 300 A Extra invertase activity (Total U) Carbon source (1% w/v) (a) glucosesucroserye flour 0 5 10 15 20 25 30 35 40 B Intra invertase activity (Total U) Carbon source (1% w/v) (b) glucosesucroserye flour 0 100 200 300 400 500 600 700 C Extra invertase activity (Total U) Carbon source (1% w/v) (c) glucosesucroserye flour 0 100 200 300 400 500 600 700 800 D Intra invertase ac tivity (Total U) Carbon source (1% w/v) (d) Figure 2. Influence of glucose, sucrose and rye flour on ex- tracellular ((a) and (c)) and intracellular ((b) and (d)) in- vertases production by A. nidulans ((a) and (b)) and E. ni- dulans ((c) and (d)) pre-cultured by 24 h in M5 and Khanna media, respectively, containing 1% (w/v) glucose. the presence of (NH4)2SO4 as also observed for intracel- lular enzyme. The invertase production by other fugal strains in the presence of peptone and yeast extract has been mentioned [20-22]. Considering the phosphate sources, the production of intra and extracellular inverta- ses by E. nidulans was not significantly affected, while the extracellular enzyme from A. nidulans was reduced. On the other hand, the production of intracellular form was not significantly affected by the phosphate sources used. This fact indicates that the influence of the phos- phate source is not on enzyme production, but on the secretion. Production of invertases by A. caespitosus was influenced positively in the presence of Na2HPO4 and KH2PO4 [1] as also observed for S. cerevisiae GCB-K5 [23]. Another aspect that should be considered is the myce- lium morphology. It is recognized that the mycelium is heterogeneous considering the gene expression and, con- sequently, affecting the growth and secretion. The het- erogeneity has been mentioned for peripheral and central zones of the colony. Under submerged fermentation, three types of growth can be observed: 1) disperse, 2) as clumps and 3) as pellets. These possibilities have consid- erable impact on enzyme production [13]. For both ana- morph and teleomorph stages, development as pellets was observed (data not shown), indicating that the dif- ferential enzyme production is not associated with the type of growth. The differential expression of SUC genes for anamorph and teleomorph stages can be hypothesized, probably related to nutrient obtainment for development of vegetative hyphae and reproductive structures. 3.2. Influence of Temperature and pH on Extracellular Invertase Activity The influence of the independent variables temperature (X1) and pH (X2) on the extracellular activity (depend- ent variable) was determined using factorial design (Ta- ble 2). According to the ANOVA (Table 3) for enzyme activity from E. nidulans, the quadratic temperature, lin- ear and quadratic pH effects were significant (α < 0.05) with R2 value of 0.92. For the enzyme activity from A. nidulans, the linear temperature and pH, and quadratic pH were significant (α < 0.05) with R2 value of 0.94. For both enzymes, the values of Fcalc. were higher than the value of Ftab, showing that the models are significant and predictive (data not shown). The equations (Equation (1)) and (Equation (2)) represent the models for E. nidulans and A. nidulans extracellular invertase activities, respec- tively. 2 12 2 212 1 Total 267828.8 79.5.3 132.7 271.5 103.8 UX X X XX (1) 2 11 22 21 Total 242.832.12.377.7 69.7 2.3 UXX XXX 2 X (2) The surface responses for both extracellular invertase activities (E. nidulans and A. nidulans) are presented in Figure 3. The best conditions to recover the higher en- zymatic activity were temperature of 54˚C - 62˚C and pH Copyright © 2013 SciRes. AiM
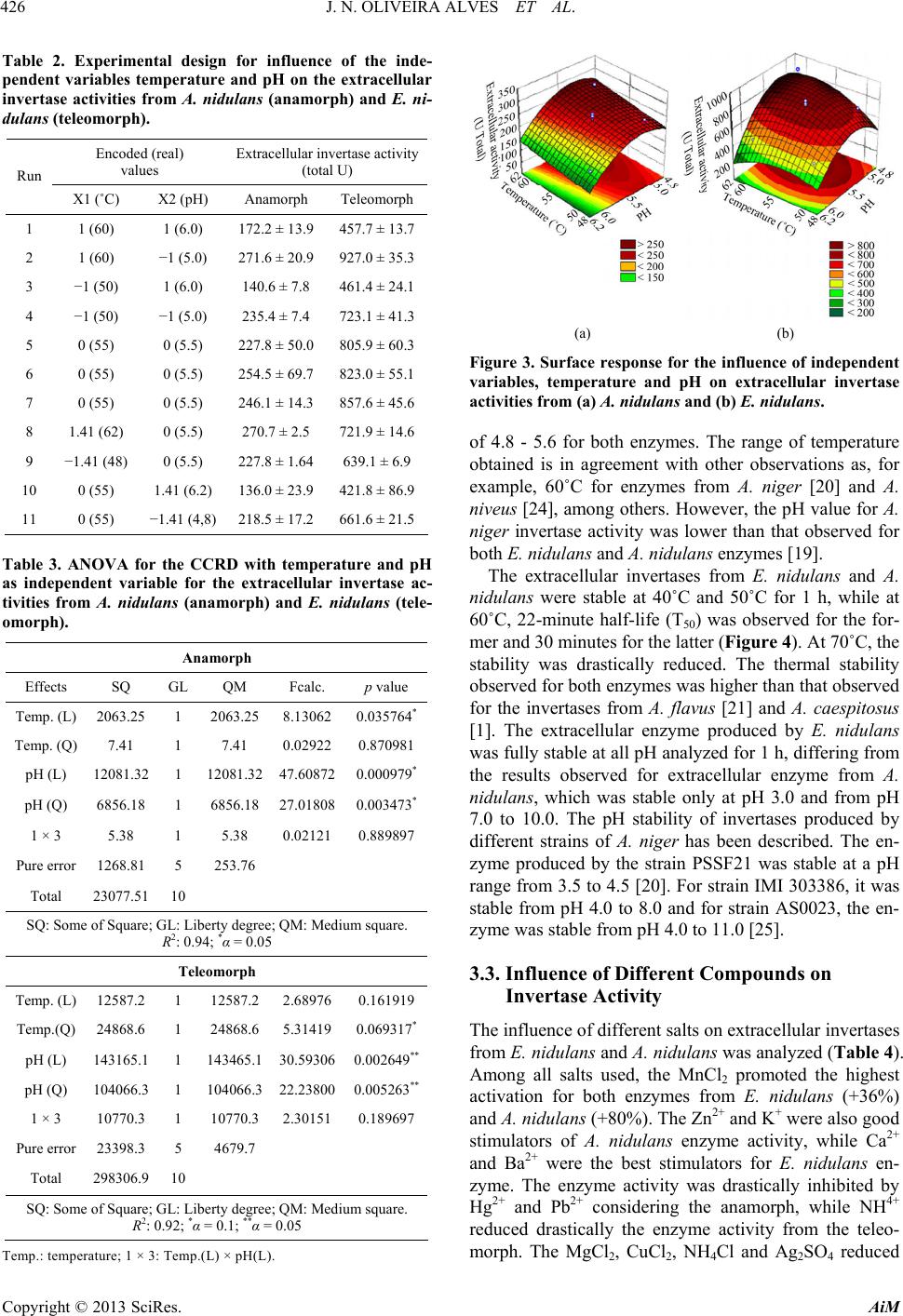 J. N. OLIVEIRA ALVES ET AL. 426 Table 2. Experimental design for influence of the inde- pendent variables temperature and pH on the extracellular invertase activities from A. nidulans (anamorph) and E. ni- dulans (teleomorph). Encoded (real) values Extracellular invertase activity (total U) Run X1 (˚C) X2 (pH) Anamorph Teleomorph 1 1 (60) 1 (6.0) 172.2 ± 13.9 457.7 ± 13.7 2 1 (60) −1 (5.0) 271.6 ± 20.9 927.0 ± 35.3 3 −1 (50) 1 (6.0) 140.6 ± 7.8 461.4 ± 24.1 4 −1 (50) −1 (5.0) 235.4 ± 7.4 723.1 ± 41.3 5 0 (55) 0 (5.5) 227.8 ± 50.0 805.9 ± 60.3 6 0 (55) 0 (5.5) 254.5 ± 69.7 823.0 ± 55.1 7 0 (55) 0 (5.5) 246.1 ± 14.3 857.6 ± 45.6 8 1.41 (62) 0 (5.5) 270.7 ± 2.5 721.9 ± 14.6 9 −1.41 (48) 0 (5.5) 227.8 ± 1.64 639.1 ± 6.9 10 0 (55) 1.41 (6.2) 136.0 ± 23.9 421.8 ± 86.9 11 0 (55) −1.41 (4,8) 218.5 ± 17.2 661.6 ± 21.5 Table 3. ANOVA for the CCRD with temperature and pH as independent variable for the extracellular invertase ac- tivities from A. nidulans (anamorph) and E. nidulans (tele- omorph). Anamorph Effects SQ GL QM Fcalc. p value Temp. (L) 2063.25 1 2063.25 8.13062 0.035764* Temp. (Q) 7.41 1 7.41 0.02922 0.870981 pH (L) 12081.32 1 12081.32 47.60872 0.000979* pH (Q) 6856.18 1 6856.18 27.01808 0.003473* 1 × 3 5.38 1 5.38 0.02121 0.889897 Pure error 1268.81 5 253.76 Total 23077.51 10 SQ: Some of Square; GL: Liberty degree; QM: Medium square. R2: 0.94; *α = 0.05 Teleomorph Temp. (L) 12587.2 1 12587.2 2.68976 0.161919 Temp.(Q) 24868.6 1 24868.6 5.31419 0.069317* pH (L) 143165.1 1 143465.1 30.59306 0.002649** pH (Q) 104066.3 1 104066.3 22.23800 0.005263** 1 × 3 10770.3 1 10770.3 2.30151 0.189697 Pure error 23398.3 5 4679.7 Total 298306.9 10 SQ: Some of Square; GL: Liberty degree; QM: Medium square. R2: 0.92; *α = 0.1; **α = 0.05 Temp.: temperature; 1 × 3: Temp.(L) × pH(L). (a) (b) Figure 3. Surface response for the influence of independent variables, temperature and pH on extracellular invertase activities from (a) A. nidulans and (b) E. nidulans. of 4.8 - 5.6 for both enzymes. The range of temperature obtained is in agreement with other observations as, for example, 60˚C for enzymes from A. niger [20] and A. niveus [24], among others. However, the pH value for A. niger invertase activity was lower than that observed for both E. nidulans and A. nidulans enzymes [19]. The extracellular invertases from E. nidulans and A. nidulans were stable at 40˚C and 50˚C for 1 h, while at 60˚C, 22-minute half-life (T50) was observed for the for- mer and 30 minutes for the latter (Figure 4). At 70˚C, the stability was drastically reduced. The thermal stability observed for both enzymes was higher than that observed for the invertases from A. flavus [21] and A. caespitosus [1]. The extracellular enzyme produced by E. nidulans was fully stable at all pH analyzed for 1 h, differing from the results observed for extracellular enzyme from A. nidulans, which was stable only at pH 3.0 and from pH 7.0 to 10.0. The pH stability of invertases produced by different strains of A. niger has been described. The en- zyme produced by the strain PSSF21 was stable at a pH range from 3.5 to 4.5 [20]. For strain IMI 303386, it was stable from pH 4.0 to 8.0 and for strain AS0023, the en- zyme was stable from pH 4.0 to 11.0 [25]. 3.3. Influence of Different Compounds on Invertase Activity The influence of different salts on extracellular invertases from E. nidulans and A. nidulans was analyzed (Table 4). Among all salts used, the MnCl2 promoted the highest activation for both enzymes from E. nidulans (+36%) and A. nidulans (+80%). The Zn2+ and K+ were also good stimulators of A. nidulans enzyme activity, while Ca2+ and Ba2+ were the best stimulators for E. nidulans en- zyme. The enzyme activity was drastically inhibited by Hg2+ and Pb2+ considering the anamorph, while NH4+ reduced drastically the enzyme activity from the teleo- morph. The MgCl2, CuCl2, NH4Cl and Ag2SO4 reduced Copyright © 2013 SciRes. AiM
 J. N. OLIVEIRA ALVES ET AL. 427 Table 4. Effect of different salts and compounds on the ex- tracellular invertases activities from A. nidulans (anamorph) and E. nidulans (teleomorph). Relative invertase activity (%) Salts and compounds (1 mM) Anamorph Teleomorph Without 100 100 Ag2SO4 64.8 ± 14.1 94.3 ± 4.2 AlCl3 99.7 ± 0.1 100.4 ± 20.8 BaCl2 106.7 ± 13.7 122.1 ± 24.4 CaCl2 108.0 ± 12.1 132.8 ± 17.6 CoCl2 110.0 ± 15.2 112.5 ± 21.1 CuCl2 89.4 ± 45.3 80.5 ± 23.7 FeCl3 106.5 ± 47.9 109.3 ± 20.2 HgCl2 2.3 ± 0.7 82.2 ± 1.2 KCl 128.5 ± 31.0 77.0 ± 13.2 MgCl2 92.2 ± 6.4 74.0 ± 13.5 MnCl2 180.0 ± 57.3 136.4 ± 16.4 NaCl 110.1 ± 33.7 109.6 ± 16.6 NH4Cl 85.0 ± 14.4 20.0 ± 1.1 Pb(C2H3O3)2 23.5 ± 8.4 65.0 ± 9.0 ZnCl2 120.2 ± 29.7 102.3 ± 5.5 β-mercaptoethanol 107.0 ± 40.5 123.4 ± 24.5 EDTA 100.5 ± 53.6 111.9 ± 24.8 the activity of both enzymes. The β-mercaptoethanol promoted an activation of 6% - 23% in the enzyme ac- tivities. The invertases from both strains showed differ- ent behaviors in the presence of the salt solutions used, despite the activation by Mn2+, but at a different propor- tion. The best enzyme activation by Mn2+ was observed when we used 25 mM and 10 mM of MnCl2 for inverta- ses from A. nidulans and E. nidulans, respectively (data not shown). These data reinforce that the enzymes have different properties according to the period of life cycle. Activation of the enzyme activity by Mn2+ was also ob- served for invertases from Aspergillus niveus [24], As- pergillus phoenicis [26] and Aspergillus ochraceus [27]. Metallic ions can affect the aminoacid residues through the induction of charge modifications promoting an in- crease or decrease in the enzyme activity (sometimes due to structural distortion). 3.4. Hydrolysis of Substrates and Kinetic Parameters The extracellular enzymes from anamorph and teleo- morph fungi were able to catalyze the hydrolysis of all substrates analyzed and their combinations (Table 5). The high hydrolytic activity using only one substrate for Table 5. Hydrolysis of different substrates by the extracel- lular invertases from A. nidulans (anamorph) and E. nidu- lans (teleomorph). Specific activities (U·mg−1 of protein) Substrates Anamorph Teleomorph Inulin 14.5 ± 3.5 7.3 ± 3.6 Raffinose 138.8 ± 0.7 51.0 ± 8.9 Sucrose 106.7 ± 7.8 193.0 ± 12.3 Inulin + sucrose 443.5 ± 7.8 232.3 ± 5.7 Raffinose + sucrose659.5 ± 9.9 256.6 ± 1.1 Inulin + raffinose 432.5 ± 2.1 51.2 ± 1.4 0 102030405060 0 20 40 60 80 100 120 A Extracellular residual activity (%) Time mi n (a) 0 102030405060 0 20 40 60 80 100 120 B Extracellular residual activity (%) Time (min) (b) Figure 4. Thermal stability at 40˚C (-■-), 50˚C (-●-), 60˚C (-▲-) and 70˚C (-▼-) for extracellular invertases produced by (a) E. nidulans and (b) A. nidulans. anamorph was observed with raffinose (138.8 U/mg of protein), and sucrose (193.0 U/mg of protein) for teleo- morph. However, the activities obtained with raffinose plus sucrose were 4.7 and 1.3-fold higher than that re- ported above, respectively. Additionaly, the S/I value was 7.3 and 26.5 for extracellular enzymes from A. nidulans and E. nidulans, respectively. The S/I value is used as a parameter to help determine the real nature of the enzymes, i.e., invertase or inulinase. Nevertheless, this parameter cannot be considered isolated and other aspects as kinetic parameters and structural characteriza- tion should be performed. One of the factors that can influence the S/I value is the inulin source, which can be Copyright © 2013 SciRes. AiM
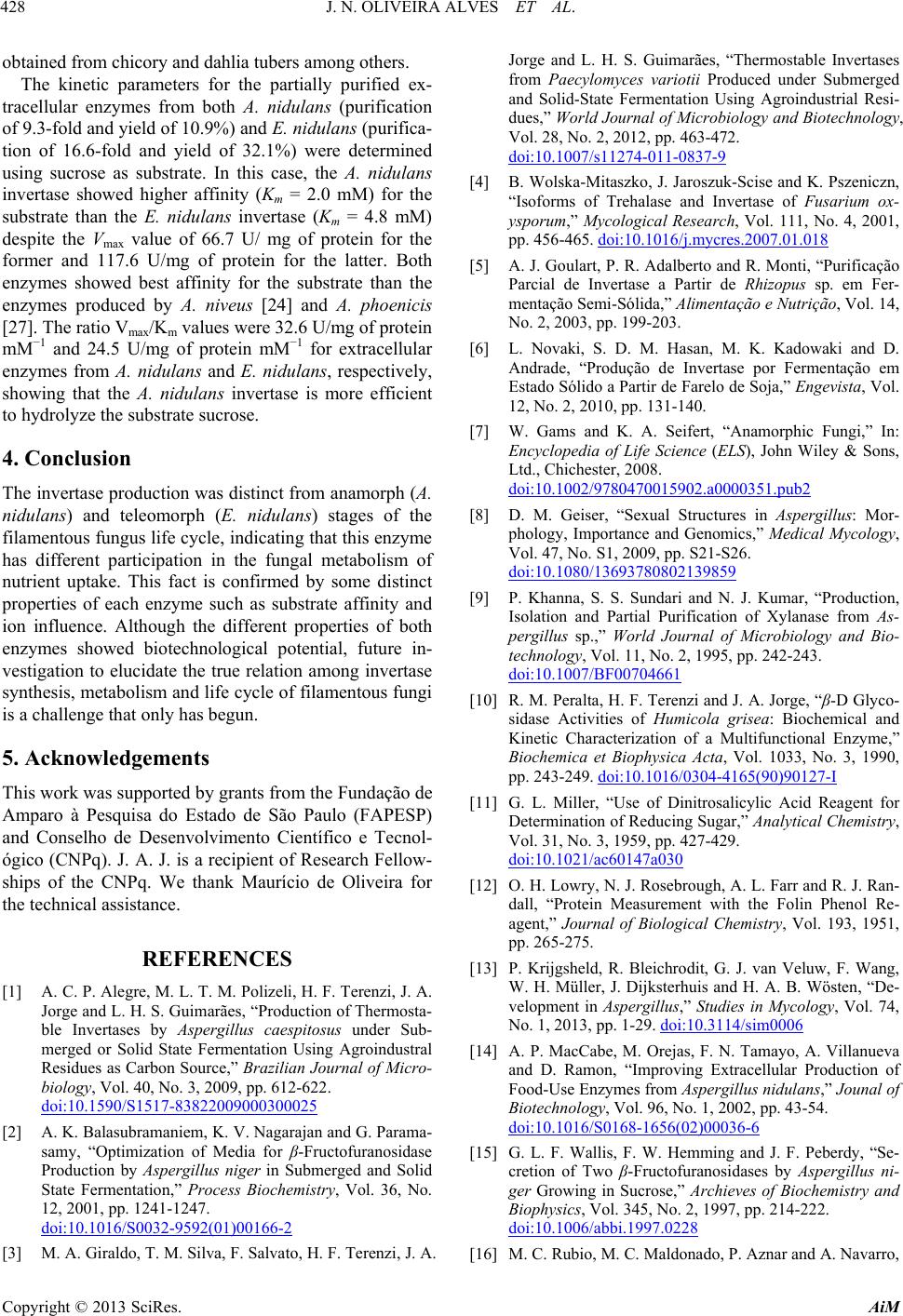 J. N. OLIVEIRA ALVES ET AL. 428 obtained from chicory and dahlia tubers among others. The kinetic parameters for the partially purified ex- tracellular enzymes from both A. nidulans (purification of 9.3-fold and yield of 10.9%) and E. nidulans (purifica- tion of 16.6-fold and yield of 32.1%) were determined using sucrose as substrate. In this case, the A. nidulans invertase showed higher affinity (Km = 2.0 mM) for the substrate than the E. nidulans invertase (Km = 4.8 mM) despite the Vmax value of 66.7 U/ mg of protein for the former and 117.6 U/mg of protein for the latter. Both enzymes showed best affinity for the substrate than the enzymes produced by A. niveus [24] and A. phoenicis [27]. The ratio Vmax/K m values were 32.6 U/mg of protein mM−1 and 24.5 U/mg of protein mM−1 for extracellular enzymes from A. nidulans and E. nidulans, respectively, showing that the A. nidulans invertase is more efficient to hydrolyze the substrate sucrose. 4. Conclusion The invertase production was distinct from anamorph (A. nidulans) and teleomorph (E. nidulans) stages of the filamentous fungus life cycle, indicating that this enzyme has different participation in the fungal metabolism of nutrient uptake. This fact is confirmed by some distinct properties of each enzyme such as substrate affinity and ion influence. Although the different properties of both enzymes showed biotechnological potential, future in- vestigation to elucidate the true relation among invertase synthesis, metabolism and life cycle of filamentous fungi is a challenge that only has begun. 5. Acknowledgements This work was supported by grants from the Fundação de Amparo à Pesquisa do Estado de São Paulo (FAPESP) and Conselho de Desenvolvimento Científico e Tecnol- ógico (CNPq). J. A. J. is a recipient of Research Fellow- ships of the CNPq. We thank Maurício de Oliveira for the technical assistance. REFERENCES [1] A. C. P. Alegre, M. L. T. M. Polizeli, H. F. Terenzi, J. A. Jorge and L. H. S. Guimarães, “Production of Thermosta- ble Invertases by Aspergillus caespitosus under Sub- merged or Solid State Fermentation Using Agroindustral Residues as Carbon Source,” Brazilian Journal of Micro- biology, Vol. 40, No. 3, 2009, pp. 612-622. doi:10.1590/S1517-83822009000300025 [2] A. K. Balasubramaniem, K. V. Nagarajan and G. Parama- samy, “Optimization of Media for β-Fructofuranosidase Production by Aspergillus niger in Submerged and Solid State Fermentation,” Process Biochemistry, Vol. 36, No. 12, 2001, pp. 1241-1247. doi:10.1016/S0032-9592(01)00166-2 [3] M. A. Giraldo, T. M. Silva, F. Salvato, H. F. Terenzi, J. A. Jorge and L. H. S. Guimarães, “Thermostable Invertases from Paecylomyces variotii Produced under Submerged and Solid-State Fermentation Using Agroindustrial Resi- dues,” World Journal of Microbiology and Biotechnology, Vol. 28, No. 2, 2012, pp. 463-472. doi:10.1007/s11274-011-0837-9 [4] B. Wolska-Mitaszko, J. Jaroszuk-Scise and K. Pszeniczn, “Isoforms of Trehalase and Invertase of Fusarium ox- ysporum,” Mycological Research, Vol. 111, No. 4, 2001, pp. 456-465. doi:10.1016/j.mycres.2007.01.018 [5] A. J. Goulart, P. R. Adalberto and R. Monti, “Purificação Parcial de Invertase a Partir de Rhizopus sp. em Fer- mentação Semi-Sólida,” Alimentação e Nutrição, Vol. 14, No. 2, 2003, pp. 199-203. [6] L. Novaki, S. D. M. Hasan, M. K. Kadowaki and D. Andrade, “Produção de Invertase por Fermentação em Estado Sólido a Partir de Farelo de Soja,” Engevista, Vol. 12, No. 2, 2010, pp. 131-140. [7] W. Gams and K. A. Seifert, “Anamorphic Fungi,” In: Encyclopedia of Life Science (ELS), John Wiley & Sons, Ltd., Chichester, 2008. doi:10.1002/9780470015902.a0000351.pub2 [8] D. M. Geiser, “Sexual Structures in Aspergillus: Mor- phology, Importance and Genomics,” Medical Mycology, Vol. 47, No. S1, 2009, pp. S21-S26. doi:10.1080/13693780802139859 [9] P. Khanna, S. S. Sundari and N. J. Kumar, “Production, Isolation and Partial Purification of Xylanase from As- pergillus sp.,” World Journal of Microbiology and Bio- technology, Vol. 11, No. 2, 1995, pp. 242-243. doi:10.1007/BF00704661 [10] R. M. Peralta, H. F. Terenzi and J. A. Jorge, “β-D Glyco- sidase Activities of Humicola grisea: Biochemical and Kinetic Characterization of a Multifunctional Enzyme,” Biochemica et Biophysica Acta, Vol. 1033, No. 3, 1990, pp. 243-249. doi:10.1016/0304-4165(90)90127-I [11] G. L. Miller, “Use of Dinitrosalicylic Acid Reagent for Determination of Reducing Sugar,” Analytical Chemistry, Vol. 31, No. 3, 1959, pp. 427-429. doi:10.1021/ac60147a030 [12] O. H. Lowry, N. J. Rosebrough, A. L. Farr and R. J. Ran- dall, “Protein Measurement with the Folin Phenol Re- agent,” Journal of Biological Chemistry, Vol. 193, 1951, pp. 265-275. [13] P. Krijgsheld, R. Bleichrodit, G. J. van Veluw, F. Wang, W. H. Müller, J. Dijksterhuis and H. A. B. Wösten, “De- velopment in Aspergillus,” Studies in Mycology, Vol. 74, No. 1, 2013, pp. 1-29. doi:10.3114/sim0006 [14] A. P. MacCabe, M. Orejas, F. N. Tamayo, A. Villanueva and D. Ramon, “Improving Extracellular Production of Food-Use Enzymes from Aspergillus nidulans,” Jounal of Biotechnology, Vol. 96, No. 1, 2002, pp. 43-54. doi:10.1016/S0168-1656(02)00036-6 [15] G. L. F. Wallis, F. W. Hemming and J. F. Peberdy, “Se- cretion of Two β-Fructofuranosidases by Aspergillus ni- ger Growing in Sucrose,” Archieves of Biochemistry and Biophysics, Vol. 345, No. 2, 1997, pp. 214-222. doi:10.1006/abbi.1997.0228 [16] M. C. Rubio, M. C. Maldonado, P. Aznar and A. Navarro, Copyright © 2013 SciRes. AiM
 J. N. OLIVEIRA ALVES ET AL. Copyright © 2013 SciRes. AiM 429 “Production y Caracterizacion de uma Invertasa Extra- cellular de Penicillium glabrum,” La Alimentacion Lati- noamerica, Vol. 247, 2003, pp. 40-45. [17] R. Trumbly, “Glucose Repression in the Yeast Saccha- romyces cerevisiae,” Molecular Microbiology, Vol. 6, No. 1, 1992, pp. 15-21. doi:10.1111/j.1365-2958.1992.tb00832.x [18] J. Dynesen, H. P. Smits, L. Olsson and J. Nielsen, “Car- bon Catabolite Repression of Invertase during Batch Cul- tivations of Saccharomyces cerevisiae: The Role of Glu- cose, Fructose and Mannose,” Applied Microbiology and Biotechnology, Vol. 50, No. 5, 1998, pp. 579-582. doi:10.1007/s002530051338 [19] M. C. Rubio and A. R. Navarro, “Regulation of Invertase Synthesis in Aspergillus niger,” Enzyme and Microbial Technology, Vol. 39, No. 4, 2006, pp. 601-606. doi:10.1016/j.enzmictec.2005.11.011 [20] P. P. Reddy, G. S. Reddy and M. B. Sulochana, “Highly Termostable β-Fructofuranosidase from Aspergillus niger PSSF21 and Its Application in the Synthesis of Fructoo- ligosacharides from Agro Industrial Residue,” Asian Jour- nal of Biotechnology, Vol. 2, No. 2, 2010, pp. 86-98. [21] C. Uma, D. Gomathi, C. Muthulakshmi and V. K. Gola- palakrishnnan, “Production, Purification and Characteri- zation of Invertases by Aspergillus flavus Using Fruit Peel Was as Substrate,” Advances in Biological Research, Vol. 4, No. 1, 2010, pp. 31-36. [22] H. N. Bhatti, A. Muhammad, A. Abbas, R. Nawaz and M. A. Sheikh, “Studies on Kinetics and Thermostability of Novel Acid Invertase from Fusarium solani,” Journal of Agriculture and Food Chemistry, Vol. 54, No. 13, 2006, pp. 4617-4623. doi:10.1021/jf053194g [23] K. Shafiq, S. Ali and I. Ul-Hag, “Effect of Different Min- eral Nutrients on Invertase Production by Saccharomyces cerevisiae GCB-K5,” Biotechnology, Vol. 1, 2002, pp. 40-44. doi:10.3923/biotech.2002.40.44 [24] L. H. S. Guimarães, A. F. Somera, H. F. Terenzi, M. L. T. M. Polizeli and J. A. Jorge, “Production of β-Fructofu- ranosidases by Aspergillus niveus Using Agroindustrial Residues as Carbon Sources: Characterization of an In- tracellular Enzyme Accumulated in the Presence of Glu- cose,” Process Biochemistry, Vol. 44, No. 2, 2009, pp. 237-241. doi:10.1016/j.procbio.2008.10.011 [25] Q. D. Nguyen, J. M. Rezessy-Szabó, M. K. Bhat and Á. Hoschke, “Purification and Some Properties of β-Fructo- furanosidase from Aspergillus niger IMI 303386,” Proc- ess Biochemistry, Vol. 40, No. 7, 2005, pp. 2461-2466. doi:10.1016/j.procbio.2004.09.012 [26] C. B. Rustiguel, A. H. C. Oliveira, H. F. Terenzi, J. A. Jorge and L. H. S. Guimarães, “Biochemical Properties of an Extracellular β-D-Fructofuranosidase II Produced by Aspergillus phoenicis under Solid-Sate Fermentation Us- ing Soy Bran as Substrate,” Electronic Journal of Bio- technology, Vol. 14, No. 2, 2011. [27] L. H. S. Guimarães, H. F. Terenzi, M. L. T. M. Polizeli and J. A. Jorge, “Production and Characterization of a Thermostable Extracellular β-D-Fructofuranosidase Pro- duced by Aspergillus ochraceus with Agroindustrial Resi- dues as Carbon Sources,” Enzyme and Microbial Tech- nology, Vol. 42, No. 1, 2007, pp. 52-57. doi:10.1016/j.enzmictec.2007.07.021
|