 World Journal of Cardiovascular Diseases, 2013, 3, 8-16 WJCD http://dx.doi.org/10.4236/wjcd.2013.35A002 Published Online August 2013 (http://www.scirp.org/journal/wjcd/) The myocardial microcirculation: A key target for salvaging ischemic myocardium? John G. Kingma Département de Médecine, Pavillon Ferdinand-Vandry 1050, Université Laval Québec, Québec, Canada Email: john.kingma@fmed.ulaval.ca Received 27 June 2013; revised 27 July 2013; accepted 30 July 2013 Copyright © 2013 John G. Kingma. This is an open access article distributed under the Creative Commons Attribution License, which permits unrestricted use, distribution, and reproduction in any medium, provided the original work is properly cited. ABSTRACT Clinical management of patients with acute myocar- dial infarction for the most part involves re-opening of an infarct-related coronary vessel by the use of clot-busting pharmacologic treatment or percutane- ous coronary interventions. While blood flow in the epicardial coronary vessel is restored downstream, ef- fects remain largely unexplored; progressive injury at the microvessel level has significant repercussions on restoration of cardiocyte viability and the ventricular blood flow and contractile function relationship. This review focuses on the cardiac microcirculation and the fact that it should be a principle target of future studies to permit improvement of clinical outcomes in patients presenting with evolving myocardial infarc- tion. Keywords: Microcirculation; Ischemia; Reperfusion; Blood Flow; Cardioprotection 1. INTRODUCTION Cardiovascular disease currently generates billions of dol- lars in healthcare costs globally and accounts for the ma- jority of deaths and disability worldwide. Principle com- plications of cardiovascular diseases include myocardial infarction and subsequent ventricular contractile failure. Acute myocardial infarction occurs subsequent to sudden obstruction of coronary blood flow (i.e. ischemia due to coronary thrombus or embolus) to a specific region of the heart muscle. The duration of blood flow deficit ul- timately determines the overall level of cellular injury and the potential for recovery of function of affected my- ocardium. With prolonged ischemia a “wavefront” of cell death, commencing from the innermost (endocardium) to the outermost (epicardium) layer of the ventricular wall develops until a fully transmural infarct is produced [1]. Irreversible damage also occurs to components of the myocardial microvasculature but it is not clear that da- mage at this level occurs prior to onset of cardiocyte ne- crosis [2]. Finally, damage also occurs at the level of in- tramyocardial nerves [3]; however, few studies have fo- cused on this aspect of post -i s c hemic myocardial injury. For patients presenting with an acute myocardial in- farction, various reperfusion strategies have been devel- oped in an attempt to delay progression of or to reduce ultimate infarct size, improve recovery of ventricular con- tractile function, limit onset of heart failure and improve clinical outcomes. Paradoxically, restoration of blood flow to the infarct-related coronary artery, though critical for myocardial salvage, could produce further injury to al- ready damaged (and even previously undamaged) cardio- cytes and thereby mitigate the potential benefits of “re- perfusion therapy”; this phenomenon is more widely re- ferred to as myocardial reperfusion injury [4-7]. Within this context various phenomenon including, reperfusion- induced arrhythmias, myocardial stunning, microvessel obstruction and lethal reperfusion injury are currently ac- knowledged [8]. Compro mised blood flow at the level of the microvasculature is generally associated with larger infarcts, reduced cardiac contractile performance, adverse LV remodelling and poor clinical outcomes [9,10]. This review focuses on the cardiac microcirculation and whe- ther it should be targeted to permit improvement of cli- nical outcomes in patients presenting with evolving myo- cardial infarction. 2. PHYSIOPATHOLOGY OF THE MICROCIRCULATION Delivery of oxygen and nutrients to tissues in each organ is the ultimate function of th e card iovascu lar syste m. The architecture of the microcirculation includes arterioles, capillaries and venules [11,12]. Crucial exchange proc- esses for oxygen, nutrients and hormones all occur at the level of the microcirculation; metabolic catabolites are also removed here. In addition, during inflammation an- OPEN ACCESS
 J. G. Kingma / World Journal of Cardiovascular Diseases 3 (2013) 8-16 9 tibodies, fibrinogen, elements of the complement system and inflammatory cells all enter injured tissues at the level of the microcirculation. Chemical and physical factors that regulate microvessel functions have been widely investigated; a specific focal point has been the production of endogenously produced compounds that could affect endothelial cells or underlying smooth mus- cle cells in different disease processes [13-15]. In the heart, the microcirculation comprises a dense in- tramyocardia l network of micro vessels that originat e from a proximal arterial system [16]. The latter is divided into three compartments: 1) epicardial coronary (conductive) arteries, 2) pre-arterioles and 3) intramural arterioles; each of these compartments has the capacity to modulate capacitance and tone so that blood flow is matched to oxygen requirements [17,18]. Krogh first described the regulation of the capillary circulation in relation to tissue oxygen supply [19], and showed in the heart that during exercise (with an increase in oxygen demand) capillary vessels could be recruited to enable adequate distribution of blood flow. A small number of coronary capillaries are open under resting conditions in the heart; when oxy- gen demand is higher additional cap illaries are recruited. In the setting of coronary artery disease vasodilatory ca- pacity of the microvasculature is reduced and aerobic threshold (i.e. critical equilibrium between oxygen sup- ply and demand) of ischemic myocardium is reached much sooner. Metabolic, neural and myogenic mechani- sms regulate blood flow within the vascular network; higher blood flows require a corresponding increase in vessel diameter particularly at the level of the microvas- culature. Cardiocyte viability post-ischemia is integrally linked to the ability of the microvasculature to deliver oxygen and nutrients either via pre-existing coronary or collat- eral networks or promotion of new vessel growth (arteri- ogenesis). Transmural distribution of coronary collaterals varies considerably between species and is genetically determined [20,21]; consequently, post-ischemic devel- opment of cardiocyte injury is directly dependent on the location of functional collateral vessels across the ven- tricular wall. Development of functional collateral ves- sels cannot be predicted in advance of an acute corona- ry event; neither can we predict which patients have the ability to develop collateral vessels after an acute insult [22]. However, an extended time frame is necessary for new vessel growth [23,24]. Existing small vessels may also undergo a process of endogenous remodeling via sti- mulation of molecular and cellular processes [25]. There is ample reference in the scientific literature regarding recruitment of coronary collateral vessels at the onset of ischemia. It would seem more reasonable to use the ter- minology of microvessel recruitment as initially suggest- ed by Krogh [19]; questions as to whether arterial vessels recruited during the acute ischemic event are true collat- eral vessels or pre-existing arterioles/capillaries that op en because of local changes in external influences on the myocardial wall independent of the vessels themselves is not trivial and should be addressed. External factors such as intramyocardial tissue pressure, coronary perfusion pressure and location within the ventricular wall all in- fluence coronary collateral circulation. The functional ef- ficacy of coronary collaterals remains controversial; cli- nical evidence of improved ventricular function post-is- chemia remains anecdotal. On a similar note reduced infarct size, incidence of arrhythmias and mortality due to the presence of functional coronary collateral circula- tion remains speculative. 3. EFFECT OF ISCHEMIA Acute obstruction of a coronary vessel initiates profound pathological changes in cardiocytes (within the area of no blood flow or anatomic area at risk) due to abrupt stoppage of biochemical and metabolic pathways. Re- duced oxygen delivery halts oxidative phosphorylation at the mitochondrial level and lead s to mitochondrial mem- brane depolarization, depletion of intracellular energy phosphate stores and inhibition of myocyte contractile function. Ultrastructural changes at the level of cardio- cytes include cellular swelling, sub sarcolemmal blebbing, cytoplasmic membrane-bound vacuoles, swollen mito- chondria, nuclear chromatin clumping and margination [26]. Within the coronary vessels vascular endothelial cells become swollen and deformed with small intralu- minal protrusions; these cells also demonstrate nuclear chromatin clumping and margination, fewer pinocytotic vesicles and intercellular separation [27]. The described cellular injury represents a small portion of the ultra- structure changes that occur briefly after onset of coro- nary occlusion. Reversibility of these ultrastructure al- terations is possible but entirely dependent on duration of the ischemic insult. Mechanisms responsible for abnor- mal blood flow to damaged myocardium have not been clearly established; capillary damage and external capil- lary compression due to edema, micro-embolization of microthrombi from atherosclerotic plaque or platelet aggregation and neutrophil plugging remain potential can- didates for poor transmural distribution of blood flow [28-30]. For patients presenting with cardiac symptoms reduc- ing the time from chest pain onset to arriv al at the hospi- tal coronary intervention unit remains the first p riority. In the pre-hospital phase different ambulatory therapeutic strategies have been shown with varying degrees of suc- cess to delay development of cardiocyt e injury. M ost phar- macologic compounds have not yet shown consistent benefit with respect to reduction of ischemic injury, pre- serving cardiac function and improving patient outcomes; Copyright © 2013 SciRes. OPEN ACCESS
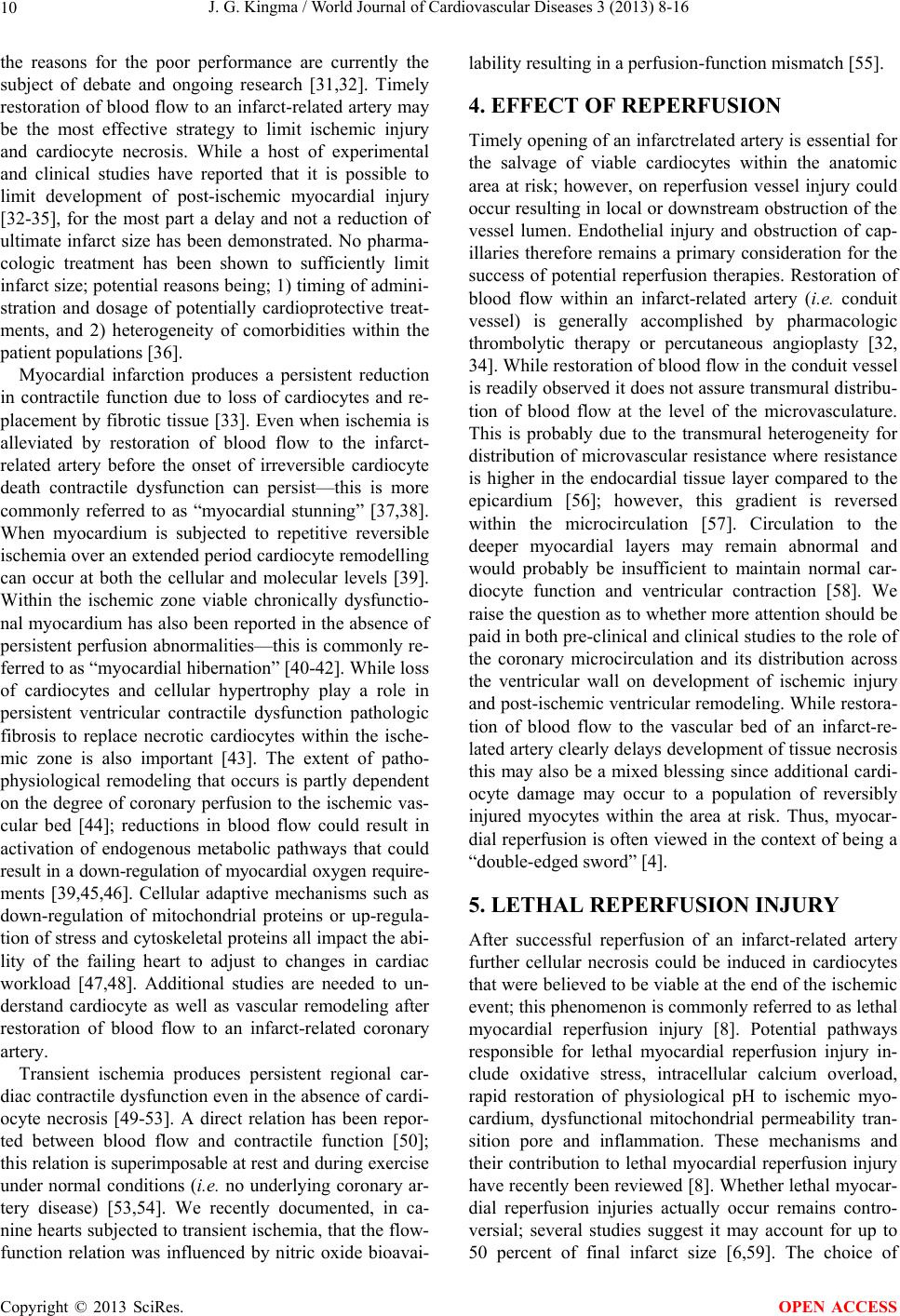 J. G. Kingma / World Journal of Cardiovascular Diseases 3 (2013) 8-16 10 the reasons for the poor performance are currently the subject of debate and ongoing research [31,32]. Timely restoration of blood flow to an infarct-related artery may be the most effective strategy to limit ischemic injury and cardiocyte necrosis. While a host of experimental and clinical studies have reported that it is possible to limit development of post-ischemic myocardial injury [32-35], for the most part a delay and not a reduction of ultimate infarct size has been demonstrated. No pharma- cologic treatment has been shown to sufficiently limit infarct size; potential reasons being; 1) timing of admini- stration and dosage of potentially cardioprotective treat- ments, and 2) heterogeneity of comorbidities within the patient populations [36]. Myocardial infarction produces a persistent reduction in contractile function due to loss of cardiocytes and re- placement by fibrotic tissue [33]. Even when ischemia is alleviated by restoration of blood flow to the infarct- related artery before the onset of irreversible cardiocyte death contractile dysfunction can persist—this is more commonly referred to as “myocardial stunning” [37,38]. When myocardium is subjected to repetitive reversible ischemia over an extended period card iocyte remodelling can occur at both the cellular and molecular levels [39]. Within the ischemic zone viable chronically dysfunctio- nal myocardium has also been reported in the absence of persistent perfusion abn ormalities—this is commonly re- ferred to as “myocardial hibernation” [40-42]. While loss of cardiocytes and cellular hypertrophy play a role in persistent ventricular contractile dysfunction pathologic fibrosis to replace necrotic cardiocytes within the ische- mic zone is also important [43]. The extent of patho- physiological remodeling that occurs is partly dependent on the degree of coronary perfusion to the ischemic vas- cular bed [44]; reductions in blood flow could result in activation of endogenous metabolic pathways that could result in a down-regulation of myocardial oxygen require- ments [39,45,46]. Cellular adaptive mechanisms such as down-regulation of mitochondrial proteins or up-regula- tion of stress and cytoskeletal proteins all impact the abi- lity of the failing heart to adjust to changes in cardiac workload [47,48]. Additional studies are needed to un- derstand cardiocyte as well as vascular remodeling after restoration of blood flow to an infarct-related coronary artery. Transient ischemia produces persistent regional car- diac contractile dysfunction even in the absence of cardi- ocyte necrosis [49-53]. A direct relation has been repor- ted between blood flow and contractile function [50]; this relation is superimposable at rest and during ex ercise under normal conditions (i.e. no underlying coronary ar- tery disease) [53,54]. We recently documented, in ca- nine hearts subjected to transient ischemia, that the flow- function relation was influenced by nitric oxide bioavai- lability resulting in a perfusion-function mismatch [55]. 4. EFFECT OF REPERFUSION Timely opening of an infarctrelated artery is essential for the salvage of viable cardiocytes within the anatomic area at risk; however, on reperfusion vessel injury could occur resulting in local or downstream obstruction of the vessel lumen. Endothelial injury and obstruction of cap- illaries therefore remains a primary consideration for the success of potential reperfusion therapies. Restoration of blood flow within an infarct-related artery (i.e. conduit vessel) is generally accomplished by pharmacologic thrombolytic therapy or percutaneous angioplasty [32, 34]. While restoration of blood flow in the conduit vessel is readily observed it do es not assure transmural distribu- tion of blood flow at the level of the microvasculature. This is probably due to the transmural heterogeneity for distribution of microvascular resistance where resistance is higher in the endocardial tissue layer compared to the epicardium [56]; however, this gradient is reversed within the microcirculation [57]. Circulation to the deeper myocardial layers may remain abnormal and would probably be insufficient to maintain normal car- diocyte function and ventricular contraction [58]. We raise the question as to whether more attention should be paid in both pre-clinical and clin ical studies to the role of the coronary microcirculation and its distribution across the ventricular wall on development of ischemic injury and post-ischemic ventricular remodeling. While restora- tion of blood flow to the vascular bed of an infarct-re- lated artery clearly delays development of tissu e necrosis this may also be a mixed blessing since additional cardi- ocyte damage may occur to a population of reversibly injured myocytes within the area at risk. Thus, myocar- dial reperfusion is often viewed in the context of being a “double-e dged sword” [4]. 5. LETHAL REPERFUSION INJURY After successful reperfusion of an infarct-related artery further cellular necrosis could be induced in cardiocytes that were believed to be viable at the end of the ischemic event; this phenomenon is commonly referred to as lethal myocardial reperfusion injury [8]. Potential pathways responsible for lethal myocardial reperfusion injury in- clude oxidative stress, intracellular calcium overload, rapid restoration of physiological pH to ischemic myo- cardium, dysfunctional mitochondrial permeability tran- sition pore and inflammation. These mechanisms and their contribution to lethal myocardial reperfusion injury have recently been reviewed [8]. Whether lethal myocar- dial reperfusion injuries actually occur remains contro- versial; several studies suggest it may account for up to 50 percent of final infarct size [6,59]. The choice of Copyright © 2013 SciRes. OPEN ACCESS
 J. G. Kingma / World Journal of Cardiovascular Diseases 3 (2013) 8-16 11 reperfusion strategy may therefore impact on the overall severity of lethal reperfusion injury inasmuch as one adheres to the dogma that reperfusion can actually be detrimental to post-ischemia cardiocyte survival. 6. NO-REFLOW More attention is being paid in the clinical setting to the phenomenon of “no-reflow” which results from endothe- lial cell injury during ischemia and develops within the ischemic vascular bed after opening an infarct-related artery. Interacting factors that contribute to no-reflow include ischemic injury, reperfusion injury, distal vessel embolization and microvessel susceptibility to injury [60]; all of these elements are associated with profound disturbances of vasoregulatory pathways [61]. Ischemia- reperfusion damage is central to the physiopathology of no-reflow which results in impaired LV remodeling, ven- tricular dysfunction and clearly impacts survival. After opening of the infarct-related vessel regional blood flow is initially hyperemic and then progressively declines [27,62-66]; the area of no-reflow progresses across the LV wall from the endocardium, is constrained to the ischemic area [2,26,58], and may depend on degree of collateral blood flow to ischemic region during coronary occlusion [66]. The causal link between microvascular and myocardial damage remains to be established [62,63, 67]; reduction of no-reflow and tissue necrosis has been documented with pharmacologic interventions given at the time of reperfusion [68]. Mechanisms responsible for no-reflow are probably quite similar across species in- cluding humans and include endothelial injury, accumu- lation of inflammatory cells, reactive oxygen intermedi- ates and the coagulation cascade. While no-reflow may not produce cardiocyte necrosis the overall consensus is that improvements in coronary collateral flow to the ischemic vascular bed will produce less ventricular re- modeling; in a retrospective clinical study of cardiovas- cular disease Rezkalla et al. reported no-reflow in more than a third of patients [69]. Intracoronary pharmaco- logic treatment in these patients resulted in normalization of flow to ischemic myocardium and, more importantly, reduced mortality. 7. CARDIAC CONDITIONING AND ISCHEMIC INJURY Cardiac conditioning represents a potential breakthrough for protection of the ischemic heart. Murry et al. initially described “ischemic preconditioning” in dogs exposed to intermittent cycles of nonlethal coronary occlusion and reperfusion prior to a period of sustained coronary occlu- sion [70]. In this study infarct size was consistently smaller in hearts pretreated by the conditioning stimulus prior to index ischemia; however, cardioprotection was not sustained when the duration of coronary occlusion was extended to 3 hours. Since the publication of this landmark paper a host of studies have attempted to eluci- date underlying mechanisms responsible for this endo- genous cellular protective phenomenon with the hope of identifying mediators amenable to pharmacologic ma- nipulation for clinical utilisation [71-73]. In the current paradigm the conditioning stimulus generates endoge- nous ligands including adenosine, opioids and catecho- lamines that trigger cellular transduction pathways and mediate protective signals from the cell membrane to mi- tochondria where end-effectors induce protection [72, 74]. Although most studies have focused on pro tection in the heart conditioning pre-treatment protection has been reported for all organs studied [75-78]. To date, cardiac conditioning has been achieved using anesthetic, phar- macological and even remote interventions; the similar- ity of mechanisms forwarded for the different condition- ing stimuli suggest the existence of a cross-tolerance phenomenon [79,80]. Even though significant progress has been made in the identification of innate protective pathways involved translation of the overall benefits of conditioning into clinical practice remains a challenge. The key requirement for organ conditioning is reper- fusion of the ischemic tissues. In humans, protection of the coronary vasculature by various conditioning mano- euvers has not been clearly established but in several reports better myocardial perfusion (higher TIMI score or myocardial blush grade and coronary flow reserve) has been reported [81,82]. Prevention of vessel dysfunc- tion by cardiac conditioning remains controversial; in animal models cardiac conditioning has been shown to conserve endothelial function and increase regional myo- cardial blood flow [62,83-87]. Vascular injury produced by myocardial ischemia-reperfusion ranges from mild functional impairment of endothelium-dependent vasodi- latation, to increased permeability and severe structural alterations to no-reflow. A recent elegant study by Sky- schally et al. examined the impact of microembolization at the onset of coronary reperfusion on myocardial in- farction in a porcine experimental preparation of ische- mia-reperfusion [88]. They showed that no-reflow and tissue necrosis were significantly attenuated in post-con- ditioned animals and suggested that embolization of mi- crovessels located primarily at the border zone (i.e. be- tween ischemic and non-ischemic myocardium) prevent- ed an increase in cardiocyte necrosis. Whittaker and Pr- zyklenk previously hypothesized that the border zone was most susceptible to further damage during post-is- chemic reperfusion of the infarct-related artery [89]. On the other hand, several recent clinical studies using post- conditioning failed to show cardioprotection thus confir- ming the need for further study [90,91]. Copyright © 2013 SciRes. OPEN ACCESS
 J. G. Kingma / World Journal of Cardiovascular Diseases 3 (2013) 8-16 12 8. SUMMARY AND CONCLUSIONS Progress has been substantial over the past several dec- ades regarding angioplasty technologies for rapid resto- ration of blood flow in infarct-related conduit vessels in humans. Keeping afflicted vessels open by implantation of a coronary stent is also effective; however, consider- able efforts are ongoing to limit problems associated with their use. While it is clear that these interventions are successful in the majority of patients to restore/main- tain patency of conductive arteries and trigger myocar- dial salvage much less is known about the recovery of cardiocyte viability and distribu tion of blood flow within the infarct core (i.e. deeper myocardial tissue layers). In the clinical setting direct anatomical quantification of blood flow in the myocardial microcirculation remains elusive. However, coronary microvascular abnormalities could explain clinical signs of myocardial isch emia often observed in patients with normal coronary angiograms (cf. Herrmann et al. for recent review [92]). Microvessel dysfunction in patients is generally estimated by the use of vasodilator agents in conjunction with positron emis- sion tomography or cardiovascular magnetic resonance techniques [16]. Myocardial contrast echocardiography is also used to assess microvessel perfusion [93,94]; us- ing this technique it has been reported that microvessel obstruction occurs in 30 - 40 percent of patients with re- perfused ST-elevation acute myocardial infarction in which optimal TIMI (Thrombolysis in Myocardial In- farction) flow was achieved [10]. The consensus of clini- cal findings with myocardial contrast echocardiography is that poor perfusion of the deeper myocardium with adequate restoration of epicardial blood flow is a primary risk factor for ventricular remodelling and major adverse cardia c ev en ts [95 ,96 ]. In ad ditio n , co ron a r y flow re se rv e can be directly measured the catheterisation laboratory; Posa et al. recently documented the occurrence of mi- crovessel obstruction immediately post-opening of the infarct-related artery in a collateral circulation poor por- cine model of ischemia-reperfusion [97]. Coronary re- serve is spatially variable [98-101]; myocardial regions with reduced intrinsic coronary reserve could be most vulnerable to transient, repetitive ischemic events that culminate in microareas of cardiocyte necrosis. In pre- clinical studies organ blood flow under diverse experi- mental conditions can be more readily evaluated by the use of microspher es [102]. Marked pro gressive r eduction of blood flow and capillary filling in the canine heart following acute myocardial ischemia has been reported even after initial demonstration of adequate blood flow in epicardial coronary arteries [66,103]. Poor blood flow at the level of the microvessels could be due to increased microcirculatory resistance distal to the site of conduit vessel occlusion [104]; clinical studies have not ye t ev a lu - ated microvessel responses or ultrastructural changes in viable but dysfunctional myocardium. It is suggested that progressive vascular remodeling of coronary resistance vessels could adversely influence acute metabolic and autoregulatory adjustments and thereby contribute to poor ventric ul ar function. In conclusion, protection against post-ischemic injury remains an important goal in patients with coronary ar- tery disease. The majority of pharmacologic compounds developed to date to delay progression of ischemic injury have not shown great promise against no-flow and its consequences—this is probably due to progressive loss of microvessel function post-ischemia. Therefore apprai- sal of changes in the coronary microcirculation within the ischemic vascular bed is absolutely central to the maintenance of cellular viability (including cardiocytes, coronary vascular cells and cardiac nerves) and restora- tion of ventricular contractile function. Future pre-clini- cal and clinical studies must take into account post-ische- mic changes occurring at the level of the myocardial mi- crovessel network and probably most-importantly within the deeper layers of the ventricular wall. Failure to do so will undoubtedly reduce th e therapeutic potential that fu- ture interventions might have for patients with acute my- ocardial infarction. REFERENCES [1] Reimer, K.A., Lowe, J.E., Rasmussen, M.M. and Jenn- ings, R.B. (1977) The wavefront phenomenon of ische- mic cell death: I. Myocardial infarct size vs. duration of coronary occlusion in dogs. Circulation, 56, 786-794. doi:10.1161/01.CIR.56.5.786 [2] Kloner, R.A., Rude, R.E., Carlson, N., Maroko, P.R., De- Boer, L.W.V. and Braunwald, E. (1980) Ultrastructural evidence of microvascular damage and myocardial cell injury after coronary artery occlusion: Which comes first? Circulation, 62, 945-952. doi:10.1161/01.CIR.62.5.945 [3] Matsunari, I., Schricke, U., Bengel, F.M., Haase, H.U., Barthel, P., Schmidt, G., Nekolla, S.G., Schoemig, A. and Schwaiger, M. (2000) Extent of cardiac sympathetic neu- ronal damage is determined by the area of ischemia in pa- tients with acute coronary syndromes. Circ ulation, 101, 2579-2585. doi:10.1161/01.CIR.101.22.2579 [4] Braunwald, E. and Kloner, R.A. (1985) Myocardial reper- fusion: A double-edged sword? The Journal of Clinical Investigation, 76, 1713-1719. doi:10.1172/JCI112160 [5] Piper, H.M., Garcia-Dorado, D. and Ovize, M. (1998) A fresh look at reperfusion injury. Cardiovascular Research, 38, 291-300. doi:10.1016/S0008-6363(98)00033-9 [6] Yellon, D.M. and Hausenloy, D.J. (2007) Myocardial re- perfusion injury. The New England Journal of Medicine, 357, 1121-1135. doi:10.1056/NEJMra071667 [7] Hearse, D.J. (1977) Reperfusion of the ischemic myocar- dium. Journal of Molecular and Cellular Cardiology, 9, 605-616. doi:10.1016/S0022-2828(77)80357-X Copyright © 2013 SciRes. OPEN ACCESS
 J. G. Kingma / World Journal of Cardiovascular Diseases 3 (2013) 8-16 13 [8] Hausenloy, D.J. and Yellon, D.M. (2013) Myocardial is- chemia-reperfusion injury: A neglected therapeutic target. The Journal of Clinical Investigation, 123, 92-100. doi:10.1172/JCI62874 [9] Ito, H., Okamura, A., Iwakura, K., Masuyama, T., Hori, M., Takiuchi, S., Negoro, S., Nakatsuchi, Y., Taniyama, Y., Higashino, Y., Fujii, K. and Minamino, T. (1996) Myocar- dial perfusion patterns related to thrombolysis in myocar- dial infarction perfusion grades after coronary angiopla- sty in patients with acute anterior wall myocardial infarc- tion. Circulation, 93, 1993-1999. doi:10.1161/01.CIR.93.11.1993 [10] Ito, H., Maruyama, A., Iwakura, K., Takiuchi, S., Masuya- ma, T., Hori, M., Higashino, Y., Fujii, K. and Minamino, T. (1996) Clinical implications of the “no reflow” phe- nomenon. A predictor of complications and left ventricu- lar remodeling in reperfused anterior wall myocardial in- farction. Circulation, 93, 223-228. doi:10.1161/01.CIR.93.2.223 [11] Palade, G.E., Simionescu, M. and Simionescu, N. (1979) Structural aspects of the permeability of the microvascu- lar endothelium. Acta Physiologica Scandinavica, S463, 11-32. [12] Granger, D.N. (1998) Physiology and pathophysiology of the microcirculation. Dialogues in Cardiovascular Medi- cine, 3, 123-140. [13] Granger, D.N. and Korthuis, R.J. (1995) Physiologic me- chanisms of postischemic tissue injury. Annual Review of Physiology, 57, 311-332. doi:10.1146/annurev.ph.57.030195.001523 [14] Harrison, D.G. (1997) Cellular and molecular mechani- sms of endothelial cell dysfunction. The Journal of Cli- nical Investigation , 100, 2153-2157. doi:10.1172/JCI119751 [15] Drexler, H. and Hornig, B. (1996) Importance of endothe- lial function in chronic heart failure. Journal of Cardi- ovascular Pharmacology, 27, S9-S12. doi:10.1097/00005344-199600002-00003 [16] White, S.K., Hausenloy, D.J. and Moon, J.C. (2012) Ima- ging the myocardial microcirculation post-myocardial in- farction. Current Heart Failure Reports, 9, 282-292. doi:10.1007/s11897-012-0111-y [17] Camici, P.G. and Crea, F. (2007) Coronary microvascular dysfunction. The New England Journal of Medicine, 356, 830-840. doi:10.1056/NEJMra061889 [18] Chilian, W.M. (1997) Coronary microcirculation in health and disease. Summary of an NHLBI workshop. Circula- tion, 95, 522-528. doi:10.1161/01.CIR.95.2.522 [19] Krogh, A. (1919) The supply of oxygen to the tissues and the regulation of the capillary circulation. The Journal of Physiology, 52, 457-474. [20] James, T.N. (1970) The delivery and distribution of coro- nary collateral circulation. Chest, 58, 183-203. doi:10.1378/chest.58.3.183 [21] De, B.M. and Schaper, W. (1971) Quantitative histology of the canine coronary collateral circulation in localized myocardial ischemia. Life Science, 10, 857-868. doi:10.1016/0024-3205(71)90157-3 [22] Newman, P.E. (1981) The coronary collateral circulation: Determinants and functional significance in ischemic heart disease. American Heart Journal, 102, 431-445. doi:10.1016/0002-8703(81)90318-5 [23] Kersten, J.R., Pagel, P.S., Chilian, W.M. and Warltier, D.C. (1999) Multifactorial basis for coronary collaterali- zation: A complex adaptive response to ischemia. Cardio- vascular Research, 43, 44-57. doi:10.1016/S0008-6363(99)00077-2 [24] Kersten, J.R. and Warltier, D.C. (1999) Modulation of the adaptive response to myocardial ischemia by coexisting disease. American Journal of Physiology, 276, H2268- H2270. [25] Heil, M. and Schaper, W. (2004) Influence of mechanical, cellular, and molecular factors on collateral artery growth (arteriogenesis). Circulation Research, 95, 449-458. doi:10.1161/01.RES.0000141145.78900.44 [26] Kloner, R.A., Ganote, C.E. and Jennings, R.B. (1974) The “no-reflow” phenomenon after temporary coronary occlu- sion in the dog. The Journal of Clinical Investigation, 54, 1496-1508. doi:10.1172/JCI107898 [27] Schwartz, B.G. and Kloner, R.A. (2012) Coronary no re- flow. Journal of Molecular and Cellular Cardiology, 52, 873-882. doi:10.1016/j.yjmcc.2011.06.009 [28] Ito, H., Okamura, A., Iwakura, K., Masuyama, T., Hori, M., Takiuchi, S., Negoro, S., Nakatsuchi, Y., Taniyama, Y., Higashino, Y., Fujii, K. and Minamino, T. (1996) Myo- cardial perfusion patterns related to thrombolysis in my- ocardial infarction perfusion grades after coronary angio- plasty in patients with acute anterior wall myocardial infarction. Circulation, 93, 1993-1999. doi:10.1161/01.CIR.93.11.1993 [29] Heusch, G., Kleinbongard, P., Bose, D., Levkau, B., Hau- de, M., Schulz, R. and Erbel, R. (2009) Coronary micro- embolization: from bedside to bench and back to bedside. Circulation, 120, 1822-1836. doi:10.1161/CIRCULATIONAHA.109.888784 [30] Luo, A.K. and Wu, K.C. (2006) Imaging microvascular obstruction and its clinical significance following acute myocardial infarction. Heart Failure Reviews, 11, 305- 312. doi:10.1007/s10741-006-0231-0 [31] Hausenloy, D.J., Baxter, G., Bell, R., Botker, H.E., Da- vidson, S.M., Downey, J., Heusch, G., Kitakaze, M., Le- cour, S., Mentzer, R., Mocanu, M.M., Ovize, M., Schulz, R., Shannon, R., Walker, M., Walkinshaw, G. and Yellon, D.M. (2010) Translating novel strategies for cardiopro- tection: The Hatter Workshop Recommendations. Basic Research in Cardiology, 105, 677-686. doi:10.1007/s00395-010-0121-4 [32] Schwartz, L.L., Kloner, R.A., Arai, A.E., Baines, C.P., Bolli, R., Braunwald, E., Downey, J., Gibbons, R.J., Got- tlieb, R.A., Heusch, G., Jennings, R.B., Lefer, D.J., Ment- zer, R.M., Murphy, E., Ovi ze, M., Ping, P., Przykle nk, K., Sack, M.N., Vander Heide, R.S., Vinten-Johansen, J. and Yellon, D.M. (2011) New horizons in cardioprotection: Recommendations from the 2010 National Heart, Lung, and Blood Institute Workshop. Circulation, 124, 1172- 1179. doi:10.1161/CIRCULATIONAHA.111.032698 [33] Kloner, R.A. and Jennings, R.B. (2001) Consequences of brief ischemia: Stunning, preconditioning, and their clini- cal implications: Part 1. Circulation, 104, 2981-2989. Copyright © 2013 SciRes. OPEN ACCESS
 J. G. Kingma / World Journal of Cardiovascular Diseases 3 (2013) 8-16 14 doi:10.1161/hc4801.100038 [34] Hausenloy, D.J. and Yellon, D.M. (2009) Myocardial pro- tection: Is primary PCI enough? Nature Clinical Practice Cardiovascular Medicine, 6, 12-13. doi:10.1038/ncpcardio1371 [35] Reimer, K.A., Jennings, R.B., Cobb, F.R., Murdock, R.H., Greenfield, J.C., Becker, L.C., Bulkley, B.H., Hutchins, G.M. , Schwartz Jr., R.P., Bailey, K.R. and Passamani, E.R. (1985) Animal models for protecting ischemic myocar- dium (AMPIM): Results of the NHLBI cooperative study. Comparison of the unconscious and conscious dog mo- dels. Circulation Research, 56, 651-665. doi:10.1161/01.RES.56.5.651 [36] Sharma, V., Bell, R.M. and Yellon, D.M. (2012) Targeting reperfusion injury in acute myocardial infarction: A re- view of reperfusion injury pharmacotherapy. Expert Opi- nion on Pharmacotherapy, 13, 1153-1175. doi:10.1517/14656566.2012.685163 [37] Bolli, R. (1992) Postischemic myocardial stunning: Pa- thogenesis, pathophysiology, and clinical relevance. In: Yellon, D.M. and Jennings, R.B., Eds., Myocardial Pro- tection: The Pathophysiology of Reperfusion and Reper- fusion Injury, Raven Press, New York, 1992, 105-149. [38] Bolli, R. (1992) Myocardial ‘stunning’ in man. Circu- lation, 86, 1671-1691. doi:10.1161/01.CIR.86.6.1671 [39] Canty Jr., J.M. and Fallavollita, J.A. (2005) Hibernating myocardium. Journal of Nuclear Cardiology, 12, 104-119. doi:10.1016/j.nuclcard.2004.11.003 [40] Tubau, J.F. and Rahimtoola, S.H. (1992) Hibernating myocardium: A historical perspective. Cardiovascular Drugs and Therapy, 6, 267-271. doi:10.1007/BF00051149 [41] Heusch, G. (1998) Hibernating myocardium. Physiolo- gical Reviews, 78, 1055-1085. [42] Heusch, G., Schulz, R. and Rahimtoola, S.H. (2005) Myocardial hibernation: A delicate balance. American Journal of Physiology: Heart and Circulatory Physiology, 288, H984-H999. doi:10.1152/ajpheart.01109.2004 [43] Beltrami, C.A., Finato, N., Rocco, M., Feruglio, G.A., Puricelli, C., Cigola, E., Quaini, F., Sonnenblick, E.H., Olivetti, G. and Anversa, P. (1994) Structural basis of end-stage failure in ischemic cardiomyopathy in humans. Circulation, 89, 151-163. doi:10.1161/01.CIR.89.1.151 [44] Canty Jr., J.M. and Suzuki, G. (2012) Myocardial per- fusion and contraction in acute ischemia and chronic ischemic heart disease. Journal of Molecular and Cellu- lar Cardiology, 52, 822-831. doi:10.1016/j.yjmcc.2011.08.019 [45] Kelly, R.F., Cabrera, J.A., Ziemba, E.A., Crampton, M., Anderson, L.B., McFalls, E.O. and Ward, H.B. (2011) Continued depression of maximal oxygen consumption and mitochondrial proteomic expression despite suc- cessful coronary artery bypass grafting in a swine model of hibernation. The Journal of Thoracic and Cardiovas- cular Surgery, 141, 261-268. doi:10.1016/j.jtcvs.2010.08.061 [46] Liem, D.A., Manintveld, O.C., Schoonderwoerd, K., McFalls, E.O., Heinen, A., Verdouw, P.D., Sluiter, W. and Duncker, D.J. (2008) Ischemic preconditioning modulates mitochondrial respiration, irrespective of the employed signal transduction pathway. Translational Research, 151, 17-26. doi:10.1016/j.trsl.2007.09.007 [47] Page, B., Young, R., Iyer, V., Suzuki, G., Lis, M., Koro- tchkina, L., Patel, M.S., Blumenthal, K.M., Fallavollita, J.A. and Canty Jr., J.M. (2008) Persistent regional down- regulation in mitochondrial enzymes and upregulation of stress proteins in swine with chronic hibernating myocar- dium. Circulation Research, 102, 103-112. doi:10.1161/CIRCRESAHA.107.155895 [48] Hu, Q., Suzuki, G., Young, R.F., Page, B.J., Fallavollita, J.A. and Canty Jr., J.M. (2009) Reductions in mitochon- drial O2 consumption and preservation of high-energy phosphate levels after simulated ischemia in chronic hibernating myocardium. American Journal of Physio- logy: Heart and Circulatory Physiology, 297, H223-H232. doi:10.1152/ajpheart.00992.2008 [49] Gall Jr., S.A., Maier, G.W., Glower, D.D., Gaynor, J.W., Cobb, F.R., Sabiston Jr., D.C. and Rankin, J.S. (1993) Recovery of myocardial function after repetitive episodes of reversible ischemia. American Journal of Physiology: Heart and Circulatory Physiology, 264, H1130-H1138. [50] Gallagher, K.P., Matsuzaki, M., Koziol, J.A., Kemper, W.S. and Ross Jr., J. (1984) Regional myocardial per- fusion and wall thickening during ischemia in conscious dogs. American Journal of Physiology: Heart and Cir- culatory Physiology, 247, H727-H738. [51] Heyndrickx, G.R., Baig, H., Nellens, P., Leusen, I., Fish- bein, M.C. and Vatner, S.F. (1978) Depression of regional blood flow and wall thickening after brief coronary occlusions. American Journal of Physiology: Heart and Circulatory Physiology, 234, H653-H659. [52] Vatner, S.F. (1980) Correlation between acute reductions in myocardial blood flow and function in conscious dogs. Circulation Research, 47, 201-207. doi:10.1161/01.RES.47.2.201 [53] Heusch, G. (2008) Heart rate in the pathophysiology of coronary blood flow and myocardial ischaemia: Benefit from selective bradycardic agents. British Journal of Pharmacology, 153, 1589-1601. doi:10.1038/sj.bjp.0707673 [54] Ross Jr., J. (1991) Myocardial perfusion-contraction ma- tching. Implications for coronary heart disease and hiber- nation. Circulation, 83, 1076-1083. doi:10.1161/01.CIR.83.3.1076 [55] Kingma, J.G., Simard, D. and Rouleau, J.R. (2011) Mo- dulation of nitric oxide affects myocardial perfusion-con- traction matching in anesthetised dogs with recurrent no- flow ischemia. Experimental Physiology, 96, 1293-1301. doi:10.1113/expphysiol.2011.060244 [56] DeFily, D.V. (1998) Control of microvascular resistance in physiological conditions and reperfusion. Journal of Molecular and Cellular Cardiology, 30, 2547-2554. doi:10.1006/jmcc.1998.0826 [57] Chilian, W.M. (1991) Microvascular pressures and resis- tances in the left ventricular subepicardium and suben- docardium. Circulation Research, 69, 561-570. doi:10.1161/01.RES.69.3.561 [58] Krug, A., Du Mesnil, D.R. and Korb, G. (1966) Blood Copyright © 2013 SciRes. OPEN ACCESS
 J. G. Kingma / World Journal of Cardiovascular Diseases 3 (2013) 8-16 15 supply of the myocardium after temporary coronary occlusion. Circula t io n R e se arch, 19, 57-62. doi:10.1161/01.RES.19.1.57 [59] Staat, P., Rioufol, G., Piot, C., Cottin, Y., Cung, T.T., L’Huillier, I., Aupetit, J.F., Bonnefoy, E., Finet, G., Andre- Fouet, X. and Ovize, M. (2005) Postconditioning the human heart. Circulation, 112, 2143-2148. doi:10.1161/CIRCULATIONAHA.105.558122 [60] Guo, A.Q., Sheng, L., Lei, X. and Shu, W. (2013) Phar- macological and physical prevention and treatment of no-reflow after primary percutaneous coronary inter- vention in ST-segment elevation myocardial infarction. Journal of International Medical Research, 41, 537-547. doi:10.1177/0300060513479859 [61] Niccoli, G., Burzotta, F., Galiuto, L. and Crea, F. (2009) Myocardial no-reflow in humans. Cardiology, 54, 281- 292. doi:10.1016/j.jacc.2009.03.054 [62] Reffelmann, T., Hale, S.L., Li, G. and Kloner, R.A. (2002) Relationship between no reflow and infarct size as in- fluenced by the duration of ischemia and reperfusion. American Journal of Physiology: Heart and Circulatory Physiology, 282, H766-H772. [63] Reffelmann, T. and Kloner, R.A. (2002) Microvascular reperfusion injury: Rapid expansion of anatomic no re- flow during reperfusion in the rabbit. American Journal of Physiology: Heart and Circulatory Physiology, 283, H1099-H1107. [64] Galiuto, L. and Iliceto, S. (1998) Myocardial contrast echocardiography in the evaluation of viable myocardium after acute myocardial infarction. American Journal of Cardiology, 81, 29G-32G. doi:10.1016/S0002-9149(98)00050-2 [65] Galiuto, L., DeMa ria, A.N., May -Newma n, K., Del, B.U., Ohmori, K., Bhargava, V., Flaim, S.F. and Iliceto, S. (1998) Evaluation of dynamic changes in microvascular flow during ischemia-reperfusion by myocardial contrast echocardiography. Cardiology, 32, 1096-1101. doi:10.1016/S0735-1097(98)00349-0 [66] Ambrosio, G., Weisman, H.F., Mannisi, J.A. and Becker, L.C. (1989) Progressive impairment of regional myocar- dial perfusion after initial restoration of postischemic blood flow. Circulation, 80, 1846-1861. doi:10.1161/01.CIR.80.6.1846 [67] Golino, P., Maroko, P.R. and Carew, T.E. (1987) The effect of acute hypercholesterolemia on myocardial infarct size and the no-reflow phenomenon during coro- nary occlusion-reperfusion. Circulation, 75, 292-298. doi:10.1161/01.CIR.75.1.292 [68] Matsumura, K., Jeremy, R.W., Schaper, J. and Becker, L.C. (1998) Progression of myocardial necrosis during re- perfusion of ischemic myocardium. Circulation, 97, 795- 804. doi:10.1161/01.CIR.97.8.795 [69] Rezkalla, S.H., Dharmashankar, K.C., Abdalrahman, I.B. and Kloner, R.A. (2010) No-reflow phenomenon fol- lowing percutaneous coronary intervention for acute my- ocardial infarction: Incidence, outcome, and effect of pharmacologic therapy. Journal of Interventional Car- diology, 23, 429-436. d oi: 10. 1111 /j .1540 - 8183.2010.00561.x [70] Murry, C.E., Jennings, R.B. and Reimer, K.A. (1986) Preconditioning with ischemia: A delay of lethal cell injury in ischemic myocardium. Circulation, 74, 1124- 1136. doi:10.1161/01.CIR.74.5.1124 [71] Ovize, M., Baxter, G.F., Di, L.F., Ferdinandy, P., Garcia- Dorado, D., Hausenloy, D.J., Heusch, G., Vinten-Jo- hansen, J., Yellon, D.M. and Schulz, R. (2010) Post-con- ditioning and protection from reperfusion injury: Where do we stand? Position paper from the Working Group of Cellular Biology of the Heart of the European Society of Cardiology. Card iovas cular Research, 87, 406-423. doi:10.1093/cvr/cvq129 [72] Yellon, D.M. and Downey, J.M. (2003) Preconditioning the myocardium: From cellular physiology to clinical cardiology. Physiological Review s, 83, 1113-1151. [73] Downey, J.M., Davis, A.M. and Cohen, M.V. (2007) Signaling pathways in ischemic preconditioning. Heart Failure Reviews, 12, 181-188. doi:10.1007/s10741-007-9025-2 [74] Hausenloy, D.J. and Yellon, D.M. (2009) Preconditioning and postconditioning: Underlying mechanisms and cli- nical application. Ath ero scl ero sis , 204, 334-341. doi:10.1016/j.atherosclerosis.2008.10.029 [75] Lu, Y.Z., Wu, C.C., Huang, Y.C., Huang, C.Y., Yang, C.Y., Lee, T.C., Chen, C.F. and Yu, L.C. (2012) Neutrophil priming by hypoxic preconditioning protects against epi- thelial barrier damage and enteric bacterial translocation in intestinal ischemia/reperfusion. Laboratory Investiga- tion, 92, 783-796. doi:10.1038/labinvest.2012.11 [76] Ko, J.K. and Cho, C.H. (2011) Adaptive cytoprotection and the brain-gut axis. Digestion, 83, 19-24. doi:10.1159/000323400 [77] Meng, R., Asmaro, K., Meng, L., Liu, Y., Ma, C., Xi, C., Li, G., Ren, C., Luo, Y., Ling, F., Jia, J., Hua, Y., Wang, X., Ding, Y., Lo, E.H. and Ji, X. (2012) Upper limb ischemic preconditioning prevents recurrent stroke in intracranial arterial stenosis. Neurology, 79, 1853-1861. doi:10.1212/WNL.0b013e318271f76a [78] Koch, S., Sacco, R.L. and Perez-Pinzon, M.A. (2012) Preconditioning the brain: Moving on to the next frontier of neurotherapeutics. Stroke, 43, 1455-1457. doi:10.1161/STROKEAHA.111.646919 [79] Lynch III, C. (1999) Anesthetic preconditioning: Not just for the heart? Anesthesiology, 91, 606-608. doi:10.1097/00000542-199909000-00007 [80] Minguet, G., Joris, J. and Lamy, M. (2007) Precondi- tioning and protection against ischaemia-reperfusion in non-cardiac organs: A place for volatile anaesthetics? Eu- ropean Journal of Anaesthesiology, 24, 733-745. doi:10.1017/S0265021507000531 [81] Ma, X.J., Zhang, X.H., Li, C.M. and Luo, M. (2006) Effect of postconditioning on coronary blood flow velo- city and endothelial function in patients with acute myo- cardial infarction. Scandinavian Cardiovascular Journal, 40, 327-333. doi:10.1080/14017430601047864 [82] Laskey, W.K., Yoon, S., Calzada, N. and Ricciardi, M.J. (2008) Concordant improvements in coronary flow re- serve and ST-segment resolution during percutaneous coronary intervention for acute myocardial infarction: A benefit of postconditioning. Catheterization and Cardio- Copyright © 2013 SciRes. OPEN ACCESS
 J. G. Kingma / World Journal of Cardiovascular Diseases 3 (2013) 8-16 Copyright © 2013 SciRes. 16 OPEN ACCESS vascular Interventions, 72, 212-220. [83] Laude, K., Beauchamp, P., Thuillez, C. and Richard, V. (2002) Endothelial protective effects of preconditioning. Cardiovascular Research, 55, 466-473. doi:10.1016/S0008-6363(02)00277-8 [84] Reffelmann, T. and Kloner, R.A. (2002) Is microvascular protection by cariporide and ischemic preconditioning causally linked to myocardial salvage? American Journal of Physiology: Heart and Circulatory Physiology, 284, H1134-H1141. [85] Zhao, Z.Q., Corvera, J.S., Halkos, M.E., Kerendi, F., Wang, N.P., Guyton, R.A. and Vinten-Johansen, J. (2003) Inhibition of myocardial injury by ischemic postcondi- tioning during reperfusion: Comparison with ischemic preconditioning. American Journal of Physiology: Heart and Circulatory Physiology, 285, H579-H588. [86] Kaeffer, N., Richard, V., Francois, A., Lallemand, F., Henry, J.P. and Thuillez, C. (1996) Preconditioning pre- vents chronic reperfusion-induced coronary endothelial dysfunction in rats. American Journal of Physiology, 271, H842-H849. [87] Richard, V., Kaeffer, N., Tron, C. and Thuillez, C. (1994) Ischemic preconditioning protects against coronary endo- thelial dysfunction induced by ischemia and reperfusion. Circulation, 89, 1254-1261. doi:10.1161/01.CIR.89.3.1254 [88] Skyschally, A., Walter, B. and Heusch, G. (2012) Coro- nary microembolization during early reperfusion: Infarct extension, but protection by ischaemic postconditioning. European Heart Journal. doi:10.1093/eurheartj/ehs434 [89] Whittaker, P. and Przyklenk, K. (1994) Reduction of infarct size in vivo with ischemic preconditioning: Mathe- matical evidence for protection via non-ischemic tissue. Basic Research in Cardiology, 89, 6-15. doi:10.1007/BF00788673 [90] Freixa, X., Bellera, N., Ortiz-Perez, J.T., Jimenez, M., Pare, C., Bosch, X., De Caralt, T.M., Betriu, A. and Masotti, M. (2012) Ischaemic postconditioning revisited: Lack of effects on infarct size following primary percu- taneous coronary intervention. European Heart Journal, 33, 103-112. doi:10.1093/eurheartj/ehr297 [91] Sorensson, P. , Saleh, N., Bouvier, F., Bohm, F., Settergren, M., Caidahl, K., Tornvall, P., Arheden, H., Ryden, L. and Pernow, J. (2010) Effect of postconditioning on infarct size in patients with ST elevation myocardial infarction. Heart, 96, 1710-1715. doi:10.1136/hrt.2010.199430 [92] Herrmann, J., Kaski, J.C. and Lerman, A. (2012) Coro- nary microvascular dysfunction in the clinical setting: From mystery to reality. European Heart Journal, 33, 2771-2781. doi:10.1093/eurheartj/ehs246 [93] Dwivedi, G., Janardhanan, R., Hayat, S.A., Lim, T.K., Greaves, K. and Senior, R. (2010) Relationship between myocardial perfusion with myocardial contrast echocar- diography and function early after acute myocardial in- farction for the prediction of late recovery of function. International Journal of Cardiology, 140, 169-174. doi:10.1016/j.ijcard.2008.11.052 [94] Hayat, S.A. and Senior, R. (2008) Myocardial contrast echocardiography in ST elevation myocardial infarction: Ready for prime time? European Heart Journal, 29, 299- 314. doi:10.1093/eurheartj/ehm621 [95] Bolognese, L., Carrabba, N., Parodi, G., Santoro, G.M., Buonamici, P., Cerisano, G. and Antoniucci, D. (2004) Impact of microvascular dysfunction on left ventricular remodeling and long-term clinical outcome after primary coronary angioplasty for acute myocardial infarction. Cir- culation, 109, 1121-1126. doi:10.1161/01.CIR.0000118496.44135.A7 [96] Dwivedi, G., Janardhanan, R., Hayat, S.A., Swinburn, J.M. and Senior, R. (2007) Prognostic value of myocar- dial viability detected by myocardial contrast echocardi- ography early after acute myocardial infarction. Cardi- ology, 50, 327-334. doi:10.1016/j.jacc.2007.03.036 [97] Posa, A., Pavo, N., Hemetsberger, R., Csonka, C., Csont, T., Ferdinandy, P., Petrasi, Z., Varga, C., Pavo, I.J., Laszlo Jr., F., Huber, K. and Gyongyosi, M. (2010) Protective effect of ischaemic preconditioning on ischaemia/reper- fusion-induced microvascular obstruction determined by on-line measurements of coronary pressure and blood flow in pigs. Thrombosis and Haemostasis, 103, 450-460. doi:10.1160/TH09-03-0165 [98] Hoffman, J.I. (1995) Heterogeneity of myocardial blood flow. Basic Research in Cardiology, 90, 103-111. doi:10.1007/BF00789440 [99] Hoffman, J.I.E. (1987) A critical view of coronary reserve. Circulation, 75, 1-6. [100] Austin, R.E.J., Aldea, G.S., Coggins, D.L., Flynn, A.E. and Hoffman, J.I.E. (1990) Profound spatial hetero- geneity of coronary reserve. Discordance between pat- terns of resting and maximal myocardial blood flow. Circulation Research, 67, 319-331. doi:10.1161/01.RES.67.2.319 [101] Bassingthwaighte, J.B., King, R.B. and Roger, S.A. (1989) Fractal nature of regional myocardial blood flow hetero- geneity. Circulation Research, 65, 578-590. doi:10.1161/01.RES.65.3.578 [102] Kingma Jr., J.G., Simard, D. and Rouleau, J.R. (2005) Comparison of neutron activated and radiolabeled micro- sphere methods for measurement of transmural myocar- dial blood flow in dogs. Journal of Thromb osis and T hrom- bolysis, 19, 201-208. doi:10.1007/s11239-005-1201-4 [103] Alhaddad, I.A., Kloner, R.A., Hakim, I., Garno, J.L. and Brown Jr., E.J. (1996) Benefits of late coronary artery reperfusion on infarct expansion progressively diminish over time: relation to viable islets of myocytes within the scar. American Heart Journal, 131, 451-457. doi:10.1016/S0002-8703(96)90522-0 [104] Fearon, W.F., Aarnoudse, W., Pijls, N.H., De, B.B., Balsam, L.B., Cooke, D.T., Robbins, R.C., Fitzgerald, P.J., Yeung, A.C. and Yock, P.G. (2004) Microvascular resis- tance is not influenced by epicardial coronary artery ste- nosis severity: Experimental validation. Circulation, 109, 2269-2272. doi:10.1161/01.CIR.0000128669.99355.CB
|