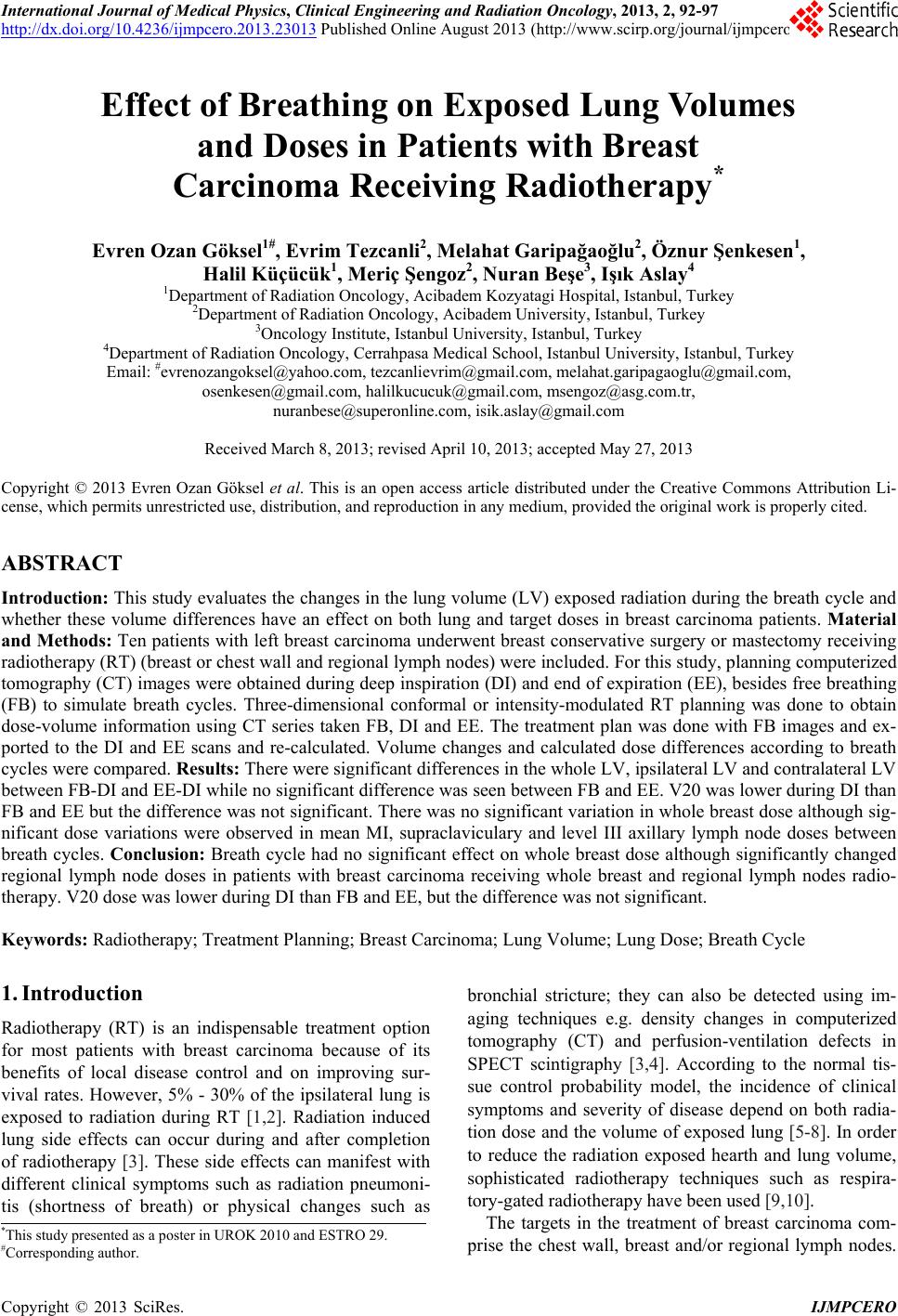 International Journal of Medical Physics, Clinical Engineering and Radiation Oncology, 2013, 2, 92-97 http://dx.doi.org/10.4236/ijmpcero.2013.23013 Published Online August 2013 (http://www.scirp.org/journal/ijmpcero) Effect of Breathing on Exposed Lung Volumes and Doses in Patients with Breast Carcinoma Receiving Radiotherapy* Evren Ozan Göksel1#, Evrim Tezcanli2, Melahat Garipağaoğlu2, Öznur Şenkesen1, Halil Küçücük1, Meriç Şengoz2, Nuran Beşe3, Işık Aslay4 1Department of Radiation Oncology, Acibadem Kozyatagi Hospital, Istanbul, Turkey 2Department of Radiation Oncology, Acibadem Univer sity, Istanbu l , T u r k ey 3Oncology Institute, Istanbul University, Istanbul, Turkey 4Department of Radiation Oncology, Cerrahpasa Medical School, Istanbul University, Istanbul, Turkey Email: #evrenozangoksel@yahoo.com, tezcanlievrim@gmail.com, melahat.garipagaoglu@gmail.com, osenkesen@gmail.com, halilkucucuk@gmail.com, msengoz@asg.com.tr, nuranbese@superonline.com, isik.aslay@gmail.com Received March 8, 2013; revised April 10, 2013; accepted May 27, 2013 Copyright © 2013 Evren Ozan Göksel et al. This is an open access article distributed under the Creative Commons Attribution Li- cense, which permits unrestricted use, distribution, and reproduction in any medium, provided the original work is properly cited. ABSTRACT Introduction: Th is study evaluates the changes in th e lung volume (LV) exposed radiation dur ing the breath cycle and whether these volume differences have an effect on both lung and target doses in breast carcinoma patients. Material and Methods: Ten patients with left breast carcinoma underwent breast conservative surgery or mastectomy receiving radiotherapy (RT) (breast or chest wall and regional lymph nodes) were included. For this study, planning computerized tomography (CT) images were obtained during deep inspiration (DI) and end of expiration (EE), besides free breathing (FB) to simulate breath cycles. Three-dimensional conformal or intensity-modulated RT planning was done to obtain dose-volume information using CT series taken FB, DI and EE. The treatment plan was done with FB images and ex- ported to the DI and EE scans and re-calculated. Volume changes and calculated dose differences according to breath cycles were compared. Results: There were significant differences in the whole LV, ipsilateral LV and contralateral LV between FB-DI and EE-DI while no significant difference was seen between FB and EE. V20 was lower during DI than FB and EE but the difference was not significant. There was no significant variation in whole breast dose although sig- nificant dose variations were observed in mean MI, supraclaviculary and level III axillary lymph node doses between breath cycles. Conclusion: Breath cycle had no significant effect on whole breast dose although significantly changed regional lymph node doses in patients with breast carcinoma receiving whole breast and regional lymph nodes radio- therapy. V20 dose was lower during DI than FB and EE, but the difference was not significant. Keywords: Radiotherapy; Treatment Planning; Breast Carcinoma; Lung Volume; Lung Dose; Breath Cycle 1. Introduction Radiotherapy (RT) is an indispensable treatment option for most patients with breast carcinoma because of its benefits of local disease control and on improving sur- vival rates. However, 5% - 30% of the ipsilateral lung is exposed to radiation during RT [1,2]. Radiation induced lung side effects can occur during and after completion of radiotherapy [3]. These side effects can manifest with different clinical symptoms such as radiation pneumoni- tis (shortness of breath) or physical changes such as bronchial stricture; they can also be detected using im- aging techniques e.g. density changes in computerized tomography (CT) and perfusion-ventilation defects in SPECT scintigraphy [3,4]. According to the normal tis- sue control probability model, the incidence of clinical symptoms and severity of disease depend on both radia- tion dose and the volume of ex posed lung [5-8]. In order to reduce the radiation exposed hearth and lung volume, sophisticated radiotherapy techniques such as respira- tory-gate d r adiotherap y have been us ed [9,10]. The targets in the treatment of breast carcinoma com- prise the chest wall, breast and/or regional lymph nodes. *This study presen ted as a poster in UROK 2010 and ESTRO 29. #Corresponding author. C opyright © 2013 SciRes. IJMPCERO
 E. O. GÖKSEL ET AL. 93 Tangential fields are often used to irradiate the breast and/or chest wall in order to protect the lungs and heart. Tangential beam axis passes through target-lung-target. A decrease in the lung tissue density results in an in- crease in electron scattering, which subsequently leads to an increase in the radiation dose in areas beyond and in front of the lung [11,12]. Therefore, tissue density het- erogeneity correction is considered during RT planning in clinical practice. RT planning is based on a series of CT images obtained without breath control (FB), which might not include all anatomical changes during a whole breath cycle. In other words, this CT series may not in- clude peak phases of breathing, namely the end of expi- ration (EE) and deep inspiration (DI). To our knowledge, there is only one study examined whether organ-tissue motion during breathing has an effect on the target dose in patients with breast carcinoma. They reported target dose was not significantly affected by breath cycles. However they looked for whole breast dose. Their study did not include regional lymph node doses [13]. This study evaluates the changes in the lung volume exposed radiation during the breath cycle and whether these volume differences have an effect on both lung and target doses in breast carcinoma patien ts. 2. Patients and Methods Ten consecutive patients with left breast carcinoma who underwent breast conservative surgery (BCS) or mastec- tomy (M), receiving radiotherapy (breast, chest wall and regional lymph nodes) were included. All patients were positioned supine on a carbon fiber breast board having a fixed base with adjustable tilting, and a body cast was fabricated to immobilize the pa- tient’s shoulder, in order to en sure daily set-up accuracy. Planning CT images were obtained from the upper neck to the upper abdomen at 3 mm intervals with a multi- detector 16 slices CT (Siemens Sensation 16 Erlangen, Germany), while the patient was in the treatment position on a flat tabletop. Because lung volumes vary with brea- thing and planning images taken during one particular moment of breath cycle such as expirium, inspirium, and these images do not represent whole breath cycles. Therefore the estimation of lung side effects based on DVH parameters is not precise. In this study, to simulate the lung volumes changes during the respiratory cycle, DI and EE series were also acquired, apart from FB im- age series, in same sequence to obtain unchanged DICOM coordinates as used in previous studies [14,15]. DI and EE image series were registered to FB, according to DICOM coordin ates using ECLIPSE version 8.6 (Varian, Palo Alto, USA) radiotherapy planning system (Figure 1). The target and organs at risk (OAR) volumes were contoured by primary Radiation Oncologist; on 3 differ- ent sets of images taken, according to RTOG breast con- Figure 1. FB and DI CT images registration according to DICOM coordinates. Lung volume changes and targets re- placement between FB and DI. touring atlas [16]. The target volumes were the breast or chest wall, lymph nodes namely level I, II, III axillaries, supraclaviculary and internal mammary (MI). Lung (whole, contralateral and ipsilateral), heart, left anterior descending artery (LAD) and contralateral breast were OAR. The lung volumes were automatically contoured by ECLIPSE using Hounsfield unit data and were manu- ally corrected. Target prescription dose was 4600 - 5000 cGy for this dosimetric study. Further planning requirements: 95% of the target volume receiving 95% of the prescription dose (4350 - 4750 cGy) and 110% of pr escription do se ( 5060 - 5500 cGy) should not exceed 5% of CTV volume. The dose constraints for OAR were: volume receiving 20 Gy (V20) < 30% for the ipsilateral lung, mean dose 3.5 Gy and V20 < 1% for heart and mean dose should not ex- ceed 1 Gy, for contralateral breast V3.5 < 1%. Primarily, three-dimensional conformal planning was used for all patients using CT slices were taken during FB series for each patient. However planning requirements were not achieved for 4 patients. Consequently IMRT planning was done for these particular 4 patients. Then, all plans were exported to DI and EE image series to obtain that dose-volume information changes depending on respira- tory motion. Any parameter difference such as beam an- gles, wedges, field size, etc. of FB pla n was not al lowed in planning for DI and EE series. In order to avoid the effect of heterogeneity difference during recalculation, MU values from FB plan were entered for each field, in DI and EE plan. Modified Batho heterogeneity correction algo- rithm was on during all calculations. The absolute and percentages of whole, ipsilateral and contralateral lung V5, V20 and prescription dose (PD) volumes belong to different breath cycle namely FB, DI Copyright © 2013 SciRes. IJMPCERO
 E. O. GÖKSEL ET AL. 94 and EE were calculated and compared each other. Whe- ther there was a difference between V20 among breath cycles was studied. Furthermore, target coverage, min, mean and max targets doses for different breath cycles namely FB, DI and EE were calculated and compared each other. Parts of this study examining hearth and con- tralateral breast volume-dose changes during breath cycle were publ ishe d and bein g pu bli shed el sewhe re sepa rate ly. The significance of dose and volume changes was inves- tigated using Wilcoxon test (PASW statistics 18) [13]. 3. Results The absolute and perce ntages of V5, V20 and PD volum es of whole, ipsilateral and contralateral lung during FB, DI and EE are listed in Table 1. The differences between FB-DI were found significant for criteria namely absolute whole, ipsilateral and contralateral lung volumes, whole lung V5, ipsilateral lung V5, whole lung V20, lung vol- ume receiving PD, ipsilateral lung volume receiving PD, % lung volume receiving PD and % ipsilateral lung vol- ume receiving PD. However, contralateral lung volume was the only significant factor between FB-EE (Figure 2). Calculated min, max and mean target namely whole breast, axillary level I-III, supraclaviculary, infraclavicu- lary and M I for diffe rent breat h cycle and a re shown i n the Table 2. Average targets coverage was adequate for all breath cycle while target coverage was not adequate for 6 out of 10 patie nts. No significant difference was foun d be- tween whole breast doses. However there was a signifi- cant differences between mean MI and level III axillary lymph node doses belo ngs to FB and DI a nd mean l evel II axillary doses belongs to FB and EE (Figure 3). 4. Discussion In the present study, there were significant differences between FB-DI for absolute whole, ipsilateral, contralat- eral, V5, V20 lung volumes and volume exposed PD as reported previously [13,14]. On the other hand, absolute lung volumes were not significantly differ between FB and EE except contralateral lung volume. As known, absolute lung volume increases during inspiration in comparison to FB, because lung inflates. Percent lung volume is better than absolute volume to estimate radia- tion related side effects. Both exposed dose and % vol- ume are determine radiation side effects [17,18]. Lung functions permanently damage when exposed radiation doses greater than 20 Gy which is accepted as tolerance dose for lung [16]. Recently, Stranzl et al. reported sig- nificant dose decrease in both exposed heart and lung in their study examining benefit of radiotherapy during deep inspirium breath-hold in patients with breast carci- (a) (b) Data are shown as median values; 1) Whole lung volume (ml); 2) Ipsilateral lung volume (ml); 3) Contralateral lung volume (ml); 4) Lung volume re- ceiving 5 Gy (ml); 5) Ipsilateral lung volume receiving 5 Gy (ml); 6) Lung volume receiving 20 Gy (ml); 7) Lung volume receiving prescription dose (ml); 8) % Lung volume receiving prescription dose; 9) % Ipsilateral lung volume receiving prescription dose. Figure 2. (a) Absolute lung volume and exposed absolute lung volume; (b) Percentage of exposed lung volume dif- ferences during breath cycle. Dat a a re sh o wn a s m ed i an va lu e s; 1) Mean axillary (Level III) dose (cGy); 2) Mean (Supraclaviculary) dose (cGy); 3) Mean (MI) dose. Figure 3. Target (lymph nodes) dose differences according to breath cycle. noma receiving RT [19]. They reported significant de- crease in % ipsilateral lung V20 during deep inspirium breath-hold, average ipsilateral V20 values changes % .5 for whole group. However decrease were seen in 8 2 Copyright © 2013 SciRes. IJMPCERO
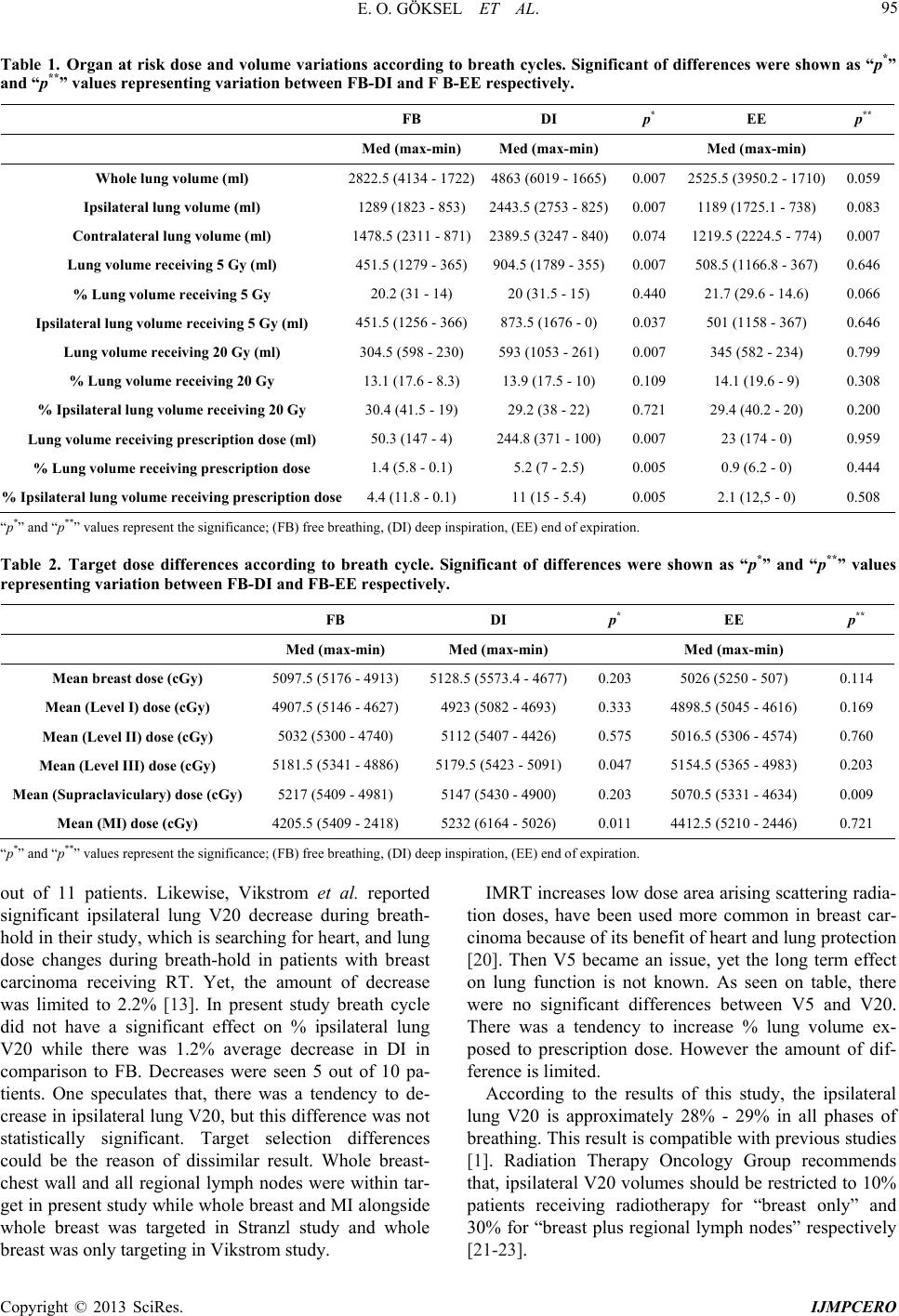 E. O. GÖKSEL ET AL. Copyright © 2013 SciRes. IJMPCERO 95 Table 1. Organ at risk dose and volume variations according to breath cycles. Significant of differences were shown as “p*” and “p**” values representing variation between FB-DI and F B-EE respectively. FB DI p* EE p** Med (max-min) Med (max-min) Med (max-min) Whole lung volume (ml) 2822.5 (4134 - 17 22)4863 (6019 - 1665)0.007 2525.5 (3950.2 - 1710)0.059 Ipsilateral lung volume (ml) 1289 (1823 - 853) 2443.5 (2753 - 82 5)0.007 1189 (1725.1 - 73 8) 0.083 Contralateral lung volume (ml) 1478.5 (2311 - 87 1)2389.5 (3247 - 840)0.074 1219.5 (2224.5 - 774)0.007 Lung volume receiving 5 Gy (ml) 451.5 (1279 - 365 )904.5 (1789 - 355)0.007 508.5 (1166.8 - 367)0.646 % Lung volume receiving 5 Gy 20.2 (31 - 14) 20 (31.5 - 15) 0.440 21.7 (29.6 - 14.6) 0.066 Ipsilateral lung volume receiving 5 Gy (ml) 451.5 (1256 - 366 )873.5 (1676 - 0) 0.037 501 (1158 - 367) 0.646 Lung volume receiving 20 Gy (ml) 304.5 (598 - 230) 593 (1053 - 261) 0.007 345 (582 - 234) 0.799 % Lung volume receiving 20 Gy 13.1 (17.6 - 8.3) 13.9 (17.5 - 10) 0.109 14.1 (19.6 - 9) 0.308 % Ipsilateral lung volume receiving 20 Gy 30.4 (41.5 - 19) 29.2 (38 - 22) 0.721 29.4 (40.2 - 20) 0.200 Lung volume receiving prescription dose (ml) 50.3 (147 - 4) 244.8 (371 - 100) 0.007 23 (174 - 0) 0.959 % Lung volume receiving prescription dose 1.4 (5.8 - 0.1) 5.2 (7 - 2.5) 0.005 0.9 (6.2 - 0) 0.444 % Ipsilateral lung volume receiving prescription dose 4.4 (11.8 - 0.1) 11 (15 - 5.4) 0.005 2.1 (12,5 - 0) 0. 508 “p*” and “p**” values represent the significance; (FB) free breathing, (DI) deep inspiration, (EE) end of expiration. Table 2. Target dose differences according to breath cycle. Significant of differences were shown as “p*” and “p**” values representing variation between FB-DI and FB-EE respectively. FB DI p* EE p** Med (max-min) Med (max-min) Med (max-min) Mean breast dose (cGy) 5097.5 (5176 - 4913) 5128.5 (5573.4 - 4677)0.203 5026 (5250 - 507) 0.114 Mean (Level I) dose (cGy) 4907.5 (5146 - 46 27) 4923 (5082 - 469 3) 0.333 4898.5 (5045 - 4616) 0.169 Mean (Level II) dose (cGy) 5032 (5300 - 4740) 5112 (5407 - 4426) 0.575 5016.5 (5306 - 4574) 0.760 Mean (Level III) dose (cGy) 5181.5 (5341 - 48 86) 5179.5 (5423 - 5091) 0.047 5154.5 (5365 - 4983) 0.203 Mean (Supraclaviculary) dose (cGy) 5217 (5409 - 4981) 5147 (5430 - 4900) 0.203 5070.5 (53 31 - 4634) 0.009 Mean (MI) dose (cGy) 4205.5 (5409 - 24 18) 5232 (6164 - 502 6) 0.011 4412.5 (5210 - 2446) 0.721 “p*” and “p**” values represent the significance; (FB) free breathing, (DI) deep inspiration, (EE) end of expiration. out of 11 patients. Likewise, Vikstrom et al. reported significant ipsilateral lung V20 decrease during breath- hold in their study, which is searching for heart, and lung dose changes during breath-hold in patients with breast carcinoma receiving RT. Yet, the amount of decrease was limited to 2.2% [13]. In present study breath cycle did not have a significant effect on % ipsilateral lung V20 while there was 1.2% average decrease in DI in comparison to FB. Decreases were seen 5 out of 10 pa- tients. One speculates that, there was a tendency to de- crease in ipsilateral lung V20, but this differen ce was not statistically significant. Target selection differences could be the reason of dissimilar result. Whole breast- chest wall and all regional lymph nodes were within tar- get in present study while whole breast and MI alongside whole breast was targeted in Stranzl study and whole breast was only targeting in Vikstrom study. IMRT incr eases low dose area arising scattering radia- tion doses, have been used more common in breast car- cinoma because of its benefit of heart and lung protection [20]. Then V5 became an issue, yet the long term effect on lung function is not known. As seen on table, there were no significant differences between V5 and V20. There was a tendency to increase % lung volume ex- posed to prescription dose. However the amount of dif- ference is limited. According to the results of this study, the ipsilateral lung V20 is approximately 28% - 29% in all phases of breathing. This result is compatible with previous studies [1]. Radiation Therapy Oncology Group recommends that, ipsilateral V20 volumes should be restricted to 10% patients receiving radiotherapy for “breast only” and 30% for “breast plus regional lymph nodes” respectively [21-23].
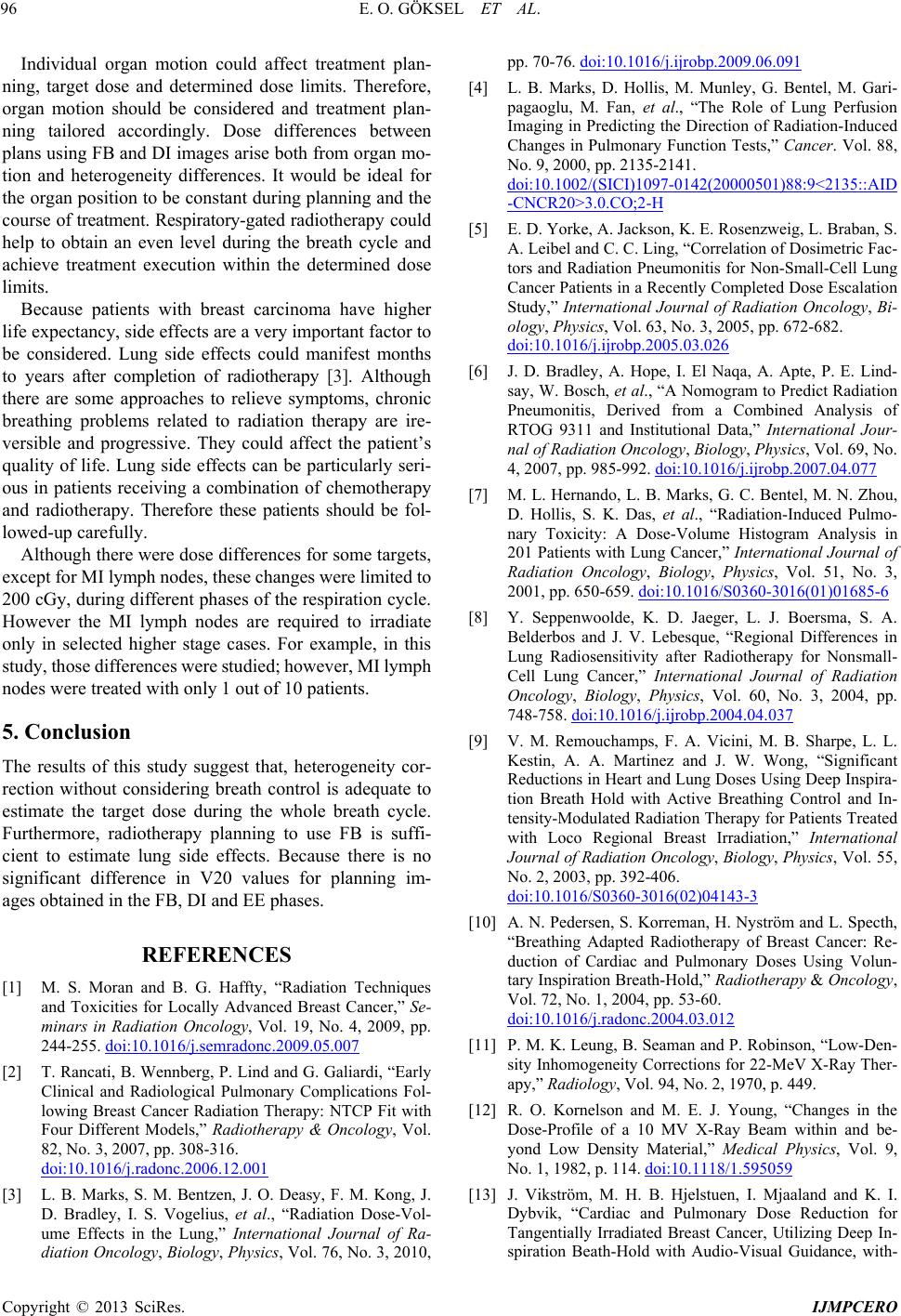 E. O. GÖKSEL ET AL. 96 Individual organ motion could affect treatment plan- ning, target dose and determined dose limits. Therefore, organ motion should be considered and treatment plan- ning tailored accordingly. Dose differences between plans using FB and DI images arise both from organ mo- tion and heterogeneity differences. It would be ideal for the organ position to be constant during planning and the course of treatment. Respiratory-gated radiotherapy could help to obtain an even level during the breath cycle and achieve treatment execution within the determined dose limits. Because patients with breast carcinoma have higher life expectancy, side effects are a very important factor to be considered. Lung side effects could manifest months to years after completion of radiotherapy [3]. Although there are some approaches to relieve symptoms, chronic breathing problems related to radiation therapy are ire- versible and progressive. They could affect the patient’s quality of life. Lung side effects can be particularly seri- ous in patients receiving a combination of chemotherapy and radiotherapy. Therefore these patients should be fol- lowed-up carefully. Although there were dose differences for some targets, except for MI lymph nodes, these changes we re lim ited t o 200 cGy, dur ing differen t phases of the respiration cycle. However the MI lymph nodes are required to irradiate only in selected higher stage cases. For example, in this study, t hose dif ferences wer e studi ed; howe ver, MI lymph nodes were treated with only 1 out of 10 patients. 5. Conclusion The results of this study suggest that, heterogeneity cor- rection without considering breath control is adequate to estimate the target dose during the whole breath cycle. Furthermore, radiotherapy planning to use FB is suffi- cient to estimate lung side effects. Because there is no significant difference in V20 values for planning im- ages obtained in the FB, DI and EE phases. REFERENCES [1] M. S. Moran and B. G. Haffty, “Radiation Techniques and Toxicities for Locally Advanced Breast Cancer,” Se- minars in Radiation Oncology, Vol. 19, No. 4, 2009, pp. 244-255. doi:10.1016/j.semradonc.2009.05.007 [2] T. Rancati, B. Wennberg, P. Lind and G. Galiardi, “Early Clinical and Radiological Pulmonary Complications Fol- lowing Breast Cancer Radiation Therapy: NTCP Fit with Four Different Models,” Radiotherapy & Oncology, Vol. 82, No. 3, 2007, pp. 308-316. doi:10.1016/j.radonc.2006.12.001 [3] L. B. Marks, S. M. Bentzen, J. O. Deasy, F. M. Kong, J. D. Bradley, I. S. Vogelius, et al., “Radiation Dose-Vol- ume Effects in the Lung,” International Journal of Ra- diation Oncology, Biology, Physics, Vol. 76, No. 3, 2010, pp. 70-76. doi:10.1016/j.ijrobp.2009.06.091 [4] L. B. Marks, D. Hollis, M. Munley, G. Bentel, M. Gari- pagaoglu, M. Fan, et al., “The Role of Lung Perfusion Imaging in Predicting the Direction of Radiation-Induced Changes in Pulmonary Function Tests,” Cancer. Vol. 88, No. 9, 2000, pp. 2135-2141. doi:10.1002/(SICI)1097-0142(20000501)88:9<2135::AID -CNCR20>3.0.CO;2-H [5] E. D. Yorke, A. Jackson, K. E. Rosenzweig, L. Braban, S. A. Leibel and C. C. Ling, “Correlation of Dosimetric Fac- tors and Radiation Pneumonitis for Non-Small-Cell Lung Cancer Patients in a Recently Completed Dose Escalation Study,” International Journal of Radiation Oncology, Bi- ology, Physics, Vol. 63, No. 3, 2005, pp. 672-682. doi:10.1016/j.ijrobp.2005.03.026 [6] J. D. Bradley, A. Hope, I. El Naqa, A. Apte, P. E. Lind- say, W. Bosch, et al., “A Nomogram to Predict Radiation Pneumonitis, Derived from a Combined Analysis of RTOG 9311 and Institutional Data,” International Jour- nal of Radiation Oncology, Biology, Physics, Vol. 69, No. 4, 2007, pp. 985-992. doi:10.1016/j.ijrobp.2007.04.077 [7] M. L. Hernando, L. B. Marks, G. C. Bentel, M. N. Zhou, D. Hollis, S. K. Das, et al., “Radiation-Induced Pulmo- nary Toxicity: A Dose-Volume Histogram Analysis in 201 Patients with Lung Cancer,” International Journal of Radiation Oncology, Biology, Physics, Vol. 51, No. 3, 2001, pp. 650-659. doi:10.1016/S0360-3016(01)01685-6 [8] Y. Seppenwoolde, K. D. Jaeger, L. J. Boersma, S. A. Belderbos and J. V. Lebesque, “Regional Differences in Lung Radiosensitivity after Radiotherapy for Nonsmall- Cell Lung Cancer,” International Journal of Radiation Oncology, Biology, Physics, Vol. 60, No. 3, 2004, pp. 748-758. doi:10.1016/j.ijrobp.2004.04.037 [9] V. M. Remouchamps, F. A. Vicini, M. B. Sharpe, L. L. Kestin, A. A. Martinez and J. W. Wong, “Significant Reductions in Heart and Lung Doses Using Deep Inspira- tion Breath Hold with Active Breathing Control and In- tensity-Modulated Radiation Therapy for Patients Treated with Loco Regional Breast Irradiation,” International Jou rna l of Radiation Oncology, Biology, Physics, Vol. 55, No. 2, 2003, pp. 392-406. doi:10.1016/S0360-3016(02)04143-3 [10] A. N. Pedersen, S. Korreman, H. Nyström and L. Specth, “Breathing Adapted Radiotherapy of Breast Cancer: Re- duction of Cardiac and Pulmonary Doses Using Volun- tary Inspiration Breath-Hold,” Radiotherapy & Oncology, Vol. 72, No. 1, 2004, pp. 53-60. doi:10.1016/j.radonc.2004.03.012 [11] P. M. K. Leung, B. Seaman and P. Robinson, “Low-Den- sity Inhomogeneity Corrections for 22-MeV X-Ray Ther- apy,” Radiology, Vol. 94, No. 2, 1970, p. 449. [12] R. O. Kornelson and M. E. J. Young, “Changes in the Dose-Profile of a 10 MV X-Ray Beam within and be- yond Low Density Material,” Medical Physics, Vol. 9, No. 1, 1982, p. 114. doi:10.1118/1.595059 [13] J. Vikström, M. H. B. Hjelstuen, I. Mjaaland and K. I. Dybvik, “Cardiac and Pulmonary Dose Reduction for Tangentially Irradiated Breast Cancer, Utilizing Deep In- spiration Beath-Hold with Audio-Visual Guidance, with- Copyright © 2013 SciRes. IJMPCERO
 E. O. GÖKSEL ET AL. Copyright © 2013 SciRes. IJMPCERO 97 out Compromising Target Coverage,” Acta Oncologica, Vol. 50, No. 1, 2011, pp. 42-50. [14] R. C. Frazier, F. A. Vicini, M. B. Sharpe, D. Yan, J. Fayad, K. L. Baglan, et al., “Impact of Breathing Motion on Breast Radiotherapy: A Dosimetric Analysis Using Active Breathing Control,” International Journal of Ra- diation Oncology, Biology, Physics, Vol. 58, No. 4, 2004, pp. 1041-1047. doi:10.1016/j.ijrobp.2003.07.005 [15] E. Tezcanli, E. Goksel, E. Yildiz, M. Garipagaoglu, O. Senkesen, H. Kucucuk, et al., “Does Radiotherapy Plan- ning without Breath Control Compensate Intra-Fraction Heart and Its Compartments’ Movement?” Breast Cancer Research and Treatment, Vol. 126, No. 1, 2011, pp. 85- 92. doi:10.1007/s10549-010-1306-0 [16] X. A. Li, A. Tai, D. W. Arthur, T. A. Buchholz, S. Mac- Donald, L. B. Marks, et al., “Variability of Target and Normal Structure Delineation for Breast-Cancer Radio- therapy: A RTOG Multi-Institutional and Multi-Observer Study,” International Journal of Radiation Oncology, Bi- ology, Physics, Vol. 73, No. 3, 2009, pp. 944-951. doi:10.1016/j.ijrobp.2008.10.034 [17] T. I. Lingos, A. Recht, F. Vicini, A. Abner, B. Silver and J. R. Harris, “Radiation Pneumonitis in Breast Cancer Pa- tients Treated with Conservative Surgery and Radiation Therapy,” International Journal of Radiation Oncology, Biology, Physics, Vol. 21, No. 2, 1991, pp. 355-360. doi:10.1016/0360-3016(91)90782-Y [18] A. G. Taghian, S. I. Assaad, A. Niemierko, I. Kuter, J. Younger, R. Schoenthaler, et al., “Risk of Pneumonitis in Breast Cancer Patients Treated with Radiation Therapy and Combination Chemotherapy with Paclitaxel,” Jour- nal of the National Cancer Institute, Vol. 93, No. 23, 2001, pp. 1806-1811. doi:10.1093/jnci/93.23.1806 [19] H. Stranzl, B. Zurl, T. Langsenlehner, K. S. Kapp, “Wide- tangential Fields Including the Internal Mammary Lymph Nodes in Patients with Left-Sided Breast Cancer,” Strah- lentherapie und Onkologie, Vol. 185, No. 2, 2009, pp. 155-160. doi:10.1007/s00066-009-1939-2 [20] E. J. Hall, C. S. Wuu, “Radiation-Induced Second Can- cers: The Impact of 3D-CRT and IMRT,” International Jou rna l of Radiation Oncology, Biology, Physics, Vol. 56, No. 1, 2003, pp. 83-88. doi:10.1016/S0360-3016(03)00073-7 [21] G. Gagliardi, J. Bjohle, I. Lax, A. Ottolenghi, F. Eriksson, A. Liedberg, et al., “Radiation Pneumonitis after Breast Cancer Irradiation: Analysis of the Complication Prob- ability Using the Relative Seriality Model,” International Jou rna l of Radiation Oncology, Biology, Physics, Vol. 46, No. 2, 2000, pp. 373-381. doi:10.1016/S0360-3016(99)00420-4 [22] N. Wolmark, W. J. Curran, F. Vicini, J. White, J. P. Co- stantino, D. Arthur, et al., “Toxicity of Three-Dimen- sional Conformal Radiotherapy for Accelerated Partial Bre ast Irradiation,” International Journ al of Radiation On- cology, Biology, Physics, Vol. 75, No. 3, 2009, pp. 1290- 1296. [23] A. J. Hope, P. E. Lindsay, I. El Naqa, J. R. Alaly, M. Vicic, J. D. Breadley, et al., “Modeling Radiation Pneu- monitis Risk with Clinical, Dosimetric, and Spatial Pa- rameters,” International Journal of Radiation Oncology, Biology, Physics, Vol. 65, No. 1, 2006, pp. 112-124. doi:10.1016/j.ijrobp.2005.11.046
|