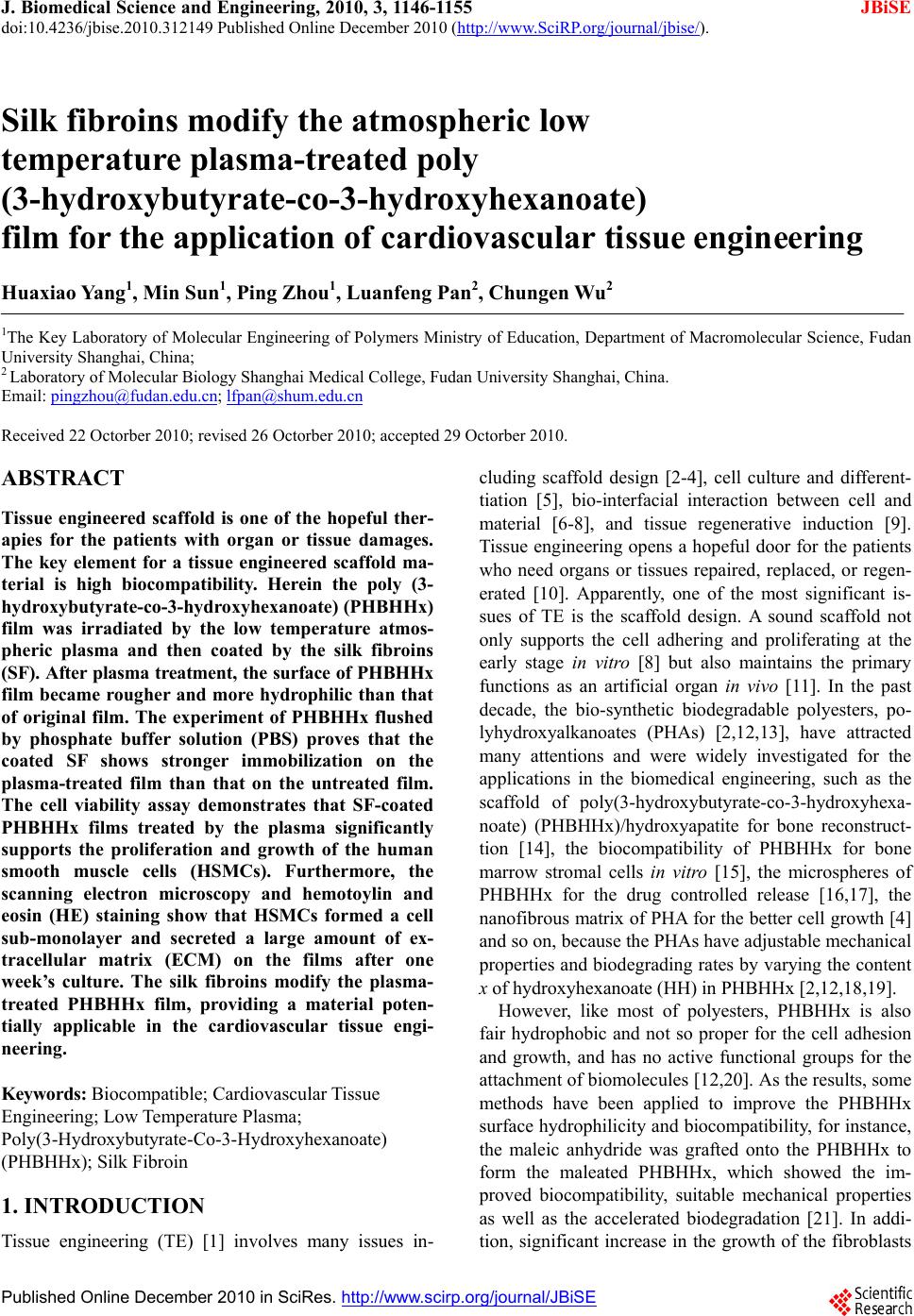 J. Biomedical Science and Engineering, 2010, 3, 1146-1155 doi:10.4236/jbise.2010.312149 Published Online December 2010 (http://www.SciRP.org/journal/jbise/ JBiSE ). Published Online December 2010 in SciRes. http://www.scirp.org/journal/JBiSE Silk fibroins modify the atmospheric low temperature plasma-treated poly (3-hydroxybutyrate-co-3-hydroxyhexanoate) film for the application of cardiovascular tissue engineering Huaxiao Yang1, Min Sun1, Ping Zhou1, Luanfeng Pan2, Chungen Wu2 1The Key Laboratory of Molecular Engineering of Polymers Ministry of Education, Department of Macromolecular Science, Fudan University Shanghai, China; 2 Laboratory of Molecular Biology Shanghai Medical College, Fudan University Shanghai, China. Email: pingzhou@fudan.edu.cn; lfpan@shum.edu.cn Received 22 Octorber 2010; revised 26 Octorber 2010; accepted 29 Octorber 2010. ABSTRACT Tissue engineered scaffold is one of the hopeful ther- apies for the patients with organ or tissue damages. The key element for a tissue engineered scaffold ma- terial is high biocompatibility. Herein the poly (3- hydroxybutyrate-co-3-hyd roxyhexanoate) (PHBHHx) film was irradiated by the low temperature atmos- pheric plasma and then coated by the silk fibroins (SF). After plasma treatment, the surface of PHBHHx film became rougher and more hydrophilic than that of original film. The experiment of PHBHHx flushed by phosphate buffer solution (PBS) proves that the coated SF shows stronger immobilization on the plasma-treated film than that on the untreated film. The cell viability assay demonstrates that SF-coated PHBHHx films treated by the plasma significantly supports the proliferation and growth of the human smooth muscle cells (HSMCs). Furthermore, the scanning electron microscopy and hemotoylin and eosin (HE) staining show that HSMCs formed a cell sub-monolayer and secreted a large amount of ex- tracellular matrix (ECM) on the films after one week’s culture. The silk fibroins modify the plasma- treated PHBHHx film, providing a material poten- tially applicable in the cardiovascular tissue engi- neering. Keywords: Biocompatible; Cardiovascular Tissue Engineering; Low Temperature Plasma; Poly(3-Hydroxybutyrate-Co-3-Hydroxyhexanoate) (PHBHHx); Silk Fibroin 1. INTRODUCTION Tissue engineering (TE) [1] involves many issues in- cluding scaffold design [2-4], cell culture and different- tiation [5], bio-interfacial interaction between cell and material [6-8], and tissue regenerative induction [9]. Tissue engineering opens a hopeful door for the patients who need organs or tissues repaired, replaced, or regen- erated [10]. Apparently, one of the most significant is- sues of TE is the scaffold design. A sound scaffold not only supports the cell adhering and proliferating at the early stage in vitro [8] but also maintains the primary functions as an artificial organ in vivo [11]. In the past decade, the bio-synthetic biodegradable polyesters, po- lyhydroxyalkanoates (PHAs) [2,12,13], have attracted many attentions and were widely investigated for the applications in the biomedical engineering, such as the scaffold of poly(3-hydroxybutyrate-co-3-hydroxyhexa- noate) (PHBHHx)/hydroxyapatite for bone reconstruct- tion [14], the biocompatibility of PHBHHx for bone marrow stromal cells in vitro [15], the microspheres of PHBHHx for the drug controlled release [16,17], the nanofibrous matrix of PHA for the better cell grow th [4] and so on, because the PHAs have adjustable mechanical properties and biodegrading rates by varying the content x of hydroxyhexanoate (HH) in PHBHHx [2,12,18,19]. However, like most of polyesters, PHBHHx is also fair hydrophobic and not so proper for the cell adhesion and growth, and has no active functional groups for the attachment of biomolecules [12,20]. As the results, some methods have been applied to improve the PHBHHx surface hydrophilicity and biocompatibility, for instance, the maleic anhydride was grafted onto the PHBHHx to form the maleated PHBHHx, which showed the im- proved biocompatibility, suitable mechanical properties as well as the accelerated biodegradation [21]. In addi- tion, significant increase in the growth of the fibroblasts
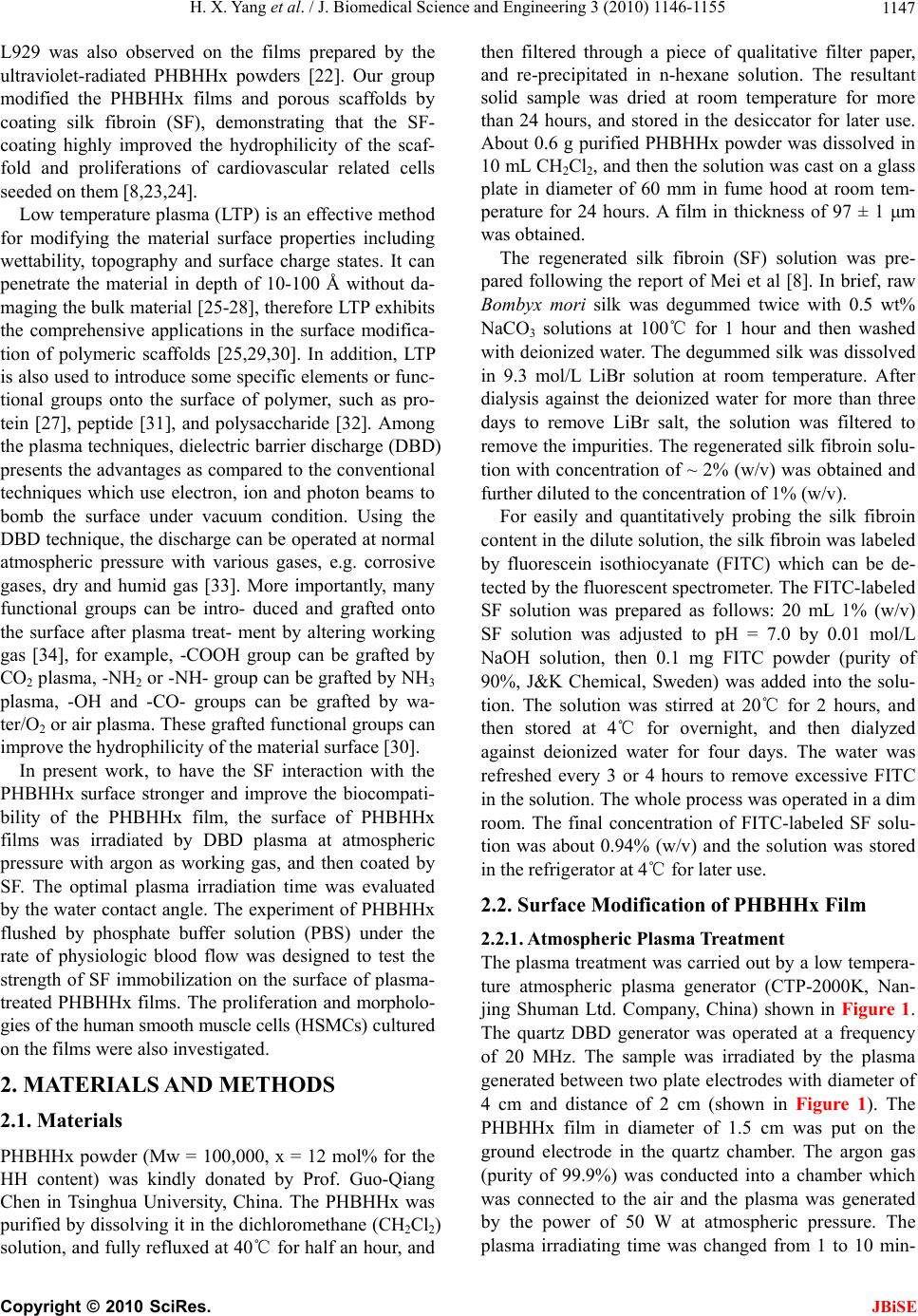 H. X. Yang et al. / J. Biomedical Science and Engineering 3 (2010) 1146-1155 1147 L929 was also observed on the films prepared by the ultraviolet-radiated PHBHHx powders [22]. Our group modified the PHBHHx films and porous scaffolds by coating silk fibroin (SF), demonstrating that the SF- coating highly improved the hydrophilicity of the scaf- fold and proliferations of cardiovascular related cells seeded on them [8,23,24]. Low temperature plasma (LTP) is an effective method for modifying the material surface properties including wettability, topography and surface charge states. It can penetrate the material in depth of 10-100 Å without da- maging the bulk material [25-28], therefore LTP exhibits the comprehensive applications in the surface modifica- tion of polymeric scaffolds [25,29,30]. In addition, LTP is also used to introduce some specific elements or func- tional groups onto the surface of polymer, such as pro- tein [27], peptide [31], and polysaccharide [32]. Among the plasma techniques, dielectric barrier discharge (DBD) presents the advantages as compared to the conventional techniques which use electron, ion and photon beams to bomb the surface under vacuum condition. Using the DBD technique, the discharge can be operated at normal atmospheric pressure with various gases, e.g. corrosive gases, dry and humid gas [33]. More importantly, many functional groups can be intro- duced and grafted onto the surface after plasma treat- ment by altering working gas [34], for example, -COOH group can be grafted by CO2 plasma, -NH2 or -NH- group can be grafted by NH3 plasma, -OH and -CO- groups can be grafted by wa- ter/O2 or air plasma. These grafted functional groups can improve the hydrophilicity of the material surface [30]. In present work, to have the SF interaction with the PHBHHx surface stronger and improve the biocompati- bility of the PHBHHx film, the surface of PHBHHx films was irradiated by DBD plasma at atmospheric pressure with argon as working gas, and then coated by SF. The optimal plasma irradiation time was evaluated by the water contact angle. The experiment of PHBHHx flushed by phosphate buffer solution (PBS) under the rate of physiologic blood flow was designed to test the strength of SF immobilization on the surface of plasma- treated PHBHH x films. The p roliferation and morpholo- gies of the human smooth muscle cells (HSMCs) cul tu r ed on the films were also investigated. 2. MATERIALS AND METHODS 2.1. Materials PHBHHx powder (Mw = 100,000, x = 12 mol% for the HH content) was kindly donated by Prof. Guo-Qiang Chen in Tsinghua University, China. The PHBHHx was purified by dissolving it in the dichloromethane (CH2Cl2) solution, and fu lly refluxed at 40℃ for half an hour, and then filtered through a piece of qualitative filter paper, and re-precipitated in n-hexane solution. The resultant solid sample was dried at room temperature for more than 24 hours, and stored in the desiccator for later use. About 0.6 g purified PHBHHx powder was dissolved in 10 mL CH2Cl2, and then th e solution was cast on a glass plate in diameter of 60 mm in fume hood at room tem- perature for 24 hours. A film in thickness of 97 ± 1 μm was obtained. The regenerated silk fibroin (SF) solution was pre- pared following the report of Mei et al [8]. In brief, raw Bombyx mori silk was degummed twice with 0.5 wt% NaCO3 solutions at 100℃ for 1 hour and then washed with deionized water. The degummed silk was dissolved in 9.3 mol/L LiBr solution at room temperature. After dialysis against the deionized water for more than three days to remove LiBr salt, the solution was filtered to remove the impurities. The regenerated silk fibroin so lu- tion with concentration of ~ 2% (w/v) was obtained and further diluted to the concentration of 1% (w/v). For easily and quantitatively probing the silk fibroin content in the dilute so lution, the silk fibroin was labeled by fluorescein isothiocyanate (FITC) which can be de- tected by the fluorescent spectrometer. The FITC-labeled SF solution was prepared as follows: 20 mL 1% (w/v) SF solution was adjusted to pH = 7.0 by 0.01 mol/L NaOH solution, then 0.1 mg FITC powder (purity of 90%, J&K Chemical, Sweden) was added into the solu- tion. The solution was stirred at 20℃ for 2 hours, and then stored at 4℃ for overnight, and then dialyzed against deionized water for four days. The water was refreshed every 3 or 4 hours to remove excessive FITC in the solution. The whole process was operated in a d im room. The final concentration of FITC-labeled SF solu- tion was about 0.94% (w/v) and the solution was stored in the refrigerator at 4℃ for later use. 2.2. Surface Modification of PHBHHx Film 2.2.1. Atm ospheric Plasma Treatment The plasma treatment was carried out by a low tempera- ture atmospheric plasma generator (CTP-2000K, Nan- jing Shuman Ltd. Company, China) shown in Figure 1. The quartz DBD generator was operated at a frequency of 20 MHz. The sample was irradiated by the plasma generated between two plate electrodes with diameter of 4 cm and distance of 2 cm (shown in Figure 1). The PHBHHx film in diameter of 1.5 cm was put on the ground electrode in the quartz chamber. The argon gas (purity of 99.9%) was conducted into a chamber which was connected to the air and the plasma was generated by the power of 50 W at atmospheric pressure. The plasma irradiating time was changed from 1 to 10 min- Copyright © 2010 SciRes. JBiSE
 H. X. Yang et al. / J. Biomedical Science and Engineering 3 (2010) 1146-1155 1148 Figure 1. Schematic graph of low temperature atmospheric plasma generating device.© utes to determine an optimal irradiating time which was evaluated by the water contact angle of the film. The smaller water contact angle indicates the better wettabil- ity of the PHBHHx films. 2.2.2. Morphology measure m e nt by SEM The morphologies of pristine and plasma treated PHBHHx films were measured by the scanning electron microscopy (SEM). Briefly, sample was sputter-coated by an ultrathin gold layer for 60 seconds with current of 10 mA, and then observed by SEM (TS 5136MM, Tes- can vega, Czech) with an accelerating voltage of 20 kV. 2.2.3. Measurement of Water Contac t Angle The water contact angle was measured by OCA 15 plus equipment (Data Physics, Germany) packaged with SCA-20 software. A drop of 1.5 μL of distilled water was dropped onto the sample surface and its contact an- gle (θ) was measured within 10 seconds. The contact angle was mean of the ten values. 2.2.4. Silk Fibroins Immobilization The PHBHHx films were immediately immersed into 1% (w/v) SF solution fo r 30 min after plasma treatment, and then freeze-dried overnight under vacuum of 0.1 torr at -50℃. At the same time, PHBHHx film without plas- ma treatment was also immersed into 1% (w/v) SF solu- tion for 30 min and freeze-dried as control. 2.2.5. Test of the Strength of SF Immobilization on the Plasma Treated PHBHHx Film The strength of SF immobilization on the plasma treated PHBHHx film was tested by flushing the sample with PBS solution. In this experiment, both of the SF-coated PHBHHx films with and without plasma treatment were flushed by recycled 90 mL PBS buffer (pH = 7.4) for 30 min at the flow rate of 55 mL/min which mimicked the physiologic blood fluid in vivo. During flushing, 2 mL flushed solution was sampled every 10 min for the fluo- rescent measurement. The content of the flushed SF in the PBS solution was measured by the FITC fluorescent at 520 nm (FLS 920, Edinburgh Instrument, Switzerland) where FITC was labeled on the SF. 2.3. Human Smooth Muscle Cell (HSMCs) Isolation and Cells Culture in Vitro HSMCs were isolated from the human umbilical artery obtained from Shanghai No.1 Hospital for the Protection of Mother and Baby’s Health and puerperal informed consent, and the method of isolation was referred to the literature [24]. In brief, a 25 cm length of human um- bilical vein was cleaned with the sterile gauze to remove blood contaminations, and then the vascular tunica me- dia layer was prudently separated from the vein. The layer was thoroughly washed with serum free Dul- becco’s modified eagle medium (DMEM, Gibco, USA) to wipe off the portion of connective tissue, and then cut into ten pieces of tissues in 1 mm3. Those pieces of tis- sues were put on the botto m of culture flask (po lystyrene , Corning, USA) in a proper distance between each other and cultured in an incubator with saturated humid air and 5% CO2 for 12 hours at 37℃ to allow the cells ad- hering and migrating in the flask. After most of tissues firmly attached on the bottom of flask, the culture me- dium (DMEM with 10% (v/v) fetal bovine serum (FBS, Hyclone, USA), 100 U streptomycin and 100 μg/ml pe- nicillin) was added into the flask, just covering over the tissue pieces for continually culturing the cells in the incubator. The medium was refreshed every 2 days until the cells grew into the confluence by 80%. The cells were gently digested with 0.25% trypsinase–0.01% EDTA solution and passed into the next passage of cells for further culture. The cells within 5 to 8 passages were used in the study. 2.4. Cells Seeded on the Studied Films HSMCs (passage of 5-8) were harvested by 0.25% tryp- sinase—0.01% EDTA and diluted to the concentration of 4.0 × 105 cells/mL. The cells were seeded on four types of PHBHHx films including PHBHHx film (P), plasma treated PHBHHx film (PP), SF-coated PHBHHx film (SP), and plasma treated PHBHHx film coated by SF (SPP). Those films were carefully put into 24-well cul- ture plates (CosterTM) and sterilized in 75% (v/v) ethanol solution overnight, and then rinsed with PBS buffer to remove the ethanol and then irradiated by ultraviolet for 30 minutes for each side of the film. 100 μL 4 × 105 cells/mL suspended cell solution was diluted in 300 μL culture medium, and dropped onto each film. After the cells adhered on the films for 30 min, extra 600 μL c ul- ture medium was supplemented into each well. The cul- Copyright © 2010 SciRes. JBiSE
 H. X. Yang et al. / J. Biomedical Science and Engineering 3 (2010) 1146-1155 1149 ture medium was refreshed every 2 days. 2.5. Proliferation Analysis of HSMCs on the Studied Films 2.5.1. Mitoch o ndri al Met ab o l i c Activity of HSMCs on the Studied Films Mitochondrial metabolic activities of the HSMCs on the different types of films were evaluated by the MTT assay. MTT (3-(4, 5)-dimethylthiah iazo (-z-y1)-3, 5-diphen yte- trazoliumromide (Merk, USA)) can be reduced by the succinate dehydrogenase when the mitochondrial of liv- ing cells interact with the MTT and form the purple for- mazan deposits. MTT assay can quantitatively assess the metabolic activity of cells when the HSMCs ar e cultured on the studied films. Briefly, after the culture medium in the plate well was removed, the cells on the films were rinsed by serum free DMEM medium three times and then incubated continu ally with 400 μL DM E M med i u m and 40 μL MTT solution (5 mg/ml in PBS) for 4 h under the humid conditio n at 37℃, and then th e insoluble pur- ple formazan crystals were formed. The crystal was dis- solved by 400 μL dimethylsulfoxide (DMSO, Sigma, USA) and 200 μL of the solution was transformed into a 96-well plate for the optical density (OD) measurement by a spectrophotometer (ELx800, BioTek, USA) at 565 nm. The 200 μL pure DMSO was used as blank control. 2.5.2. HE Staining of HSMCs on the Studied Films Hemotoylin and eosin (HE) staining is a traditional, fast and reliable histological staining method to observe the cells. In this study, the HSMCs cultured on the studied films for 3, 5 and 7 days were rinsed with PBS buffer, and fixed by 4% polyformaldehyde buffer solution (pH = 7.4) overnight at 4℃, and then dehydrated by the etha- nol with gradually increased concentration of 50, 60, 70, 80, 90, 95 and 100% (v/v). The cells were stained with hemotoylin and eosin for 1 min respectively, and then gently washed with distilled water, and sealed with liq- uid paraffin. The cells were observed under the inverted light microscope (XDS-1B, Chongqing Optical Co., China) and recorded by a digital camera (Nikon D60). 2.6. Morphology Observation of HSMCs Growing on the Studied Films Morphologies of the HSMCs cultured on the studied films for 3 and 7 days were observed by SEM. The HSMCs were fixed by 4% polyformaldehyde buffer so- lution (pH = 7.4) overnight at 4℃, subsequently, dehy- drated by the ethanol with gradually increased concen- tration of 50, 60, 70, 80, 90, 95 and 100% (v/v), and then lyophilized overnight under vacuum of 0.1 torr at -50℃. Morphologies of the HSMCs cultured on the studied films were observed by SEM. 2.7. Statistical Analysis Data were presented as means ± SD (standard deviation). Three values were measured for every sample. Statistical comparisons were performed using ANOVA one-way method (OriginTM). P < 0.05 was considered statistically significant differenc e betwee n two data gr oups. 3. RESULTS AND DISCUSSION 3.1. Influence of Atmospheric Plasma Treatment on the Films In this study, the PHBHHx films were irradiated by low temperature atmospheric argon/air plasma for time of 1, 2, 4, 6, 8 and 10 min. Plasma irradiation can break down the chemical bonds of the material to form free radicals, leading to the chemical composition rearranging and the surface roughening of polymeric matrix [33,35]. After the plasma treatment, the surface morphology of PHBHHx film became rougher than that of pristine film shown in Figure 2. The similar rough ‘zones’ were also observed when the PHBHHx film was irradiated by the ion implantation with fluence of 1×1015 ions/cm2 [20]. The hydrophilicity of the treated material surface was characterized by the water contact angles shown in Fig- ure 3. The results indicates that the water contact angles decreased from 90.3° to 70.4° when the films were irra- diated by plasma from 1 min to 6 min, and reached a constant valu e around 72° when the irradiation time was longer. The decreases in the water contact angle are con- tributed to the plenty of hydrophilic groups, such as hy- droxyl and carboxyl groups [25] formed on the surface of PHBHHx film after plasma treatment. The constant value of water contact angle is due to the hydrophilic functional groups saturated on the surfaces of films [27]. Considering the efficient treatment and no destruction for the bulk polymer, 6 min of the plasma irradiation time was selected as an optimum treating time in our experiment. The following reports will no longer men- tion this treating time any more. 3.2. Strength of SF Immobilization on the Plasma Treated PHBHHx Films The SF-coated PHBHHx films that with and without plasma treatment were flushed by PBS buffer for 30 min, and the FITC-labeled SF in the eluate was detected by the FITC fluorescence. The results are shown in Figure 4. The fluorescence increases as the flushing time increases for the SF-coated PHBHHx film without plasma treat- ment, indicating that the SF are easily desorbed from the SF-coated PHBHHx film, whilst very low fluorescence intensity and nearly no significant fluorescence change are found for the SF-coated PHBHHx film with plasma treatment, indicating that the SF were firmly adsorbed Copyright © 2010 SciRes. JBiSE
 H. X. Yang et al. / J. Biomedical Science and Engineering 3 (2010) 1146-1155 Copyright © 2010 SciRes. 1150 (a) (b) Figure 2. Comparison of the surface morphologies between pristine PHBHHx film (a) and plasma treated PHBHHx film for 6 min irradiation (b). Figure 3. The water contact angles versus the plasma irradia- tion time for the PHBHHx film. Figure 4. The fluorescents of FTIC-labeled SF in the PBS buffer solutions which were used to flush the SF-coated PHBHHx films with (in solid line) and without (in dash dot line) plasma treatment, respectively. on the plasma-treated PHBHHx film even though under the physiologic recycle-flow flushing condition, possibly due to the hydrophilic ch emical groups newly grafted on the surface. Therefore, the plasma-treated PHBHHx film is an ideal substrate for SF immobilization, and would be as a potential candidate material for the artificial blood vein to bear the high she er force of b lood flow in vivo. studied PHBHHx films up to 7 days were evaluated by MTT assay and HE staining method. The MTT assay results are shown in Figure 5 which demonstrates the relative optical densities (OD) of four types of the studied films. The high OD value indicates the high cell viability. Fro m the Figure 5, HSMCs seeded on the SF-coated PHBHHx film (SP) have the better cell viability than that on the PHBHHx film (P) after cul- tured for 7 days, which is consistent with our previous 3.3. Growth and Proliferation of HSMCs on the Studied Films The growth and proliferation of HSMCs cultured on the JBiSE
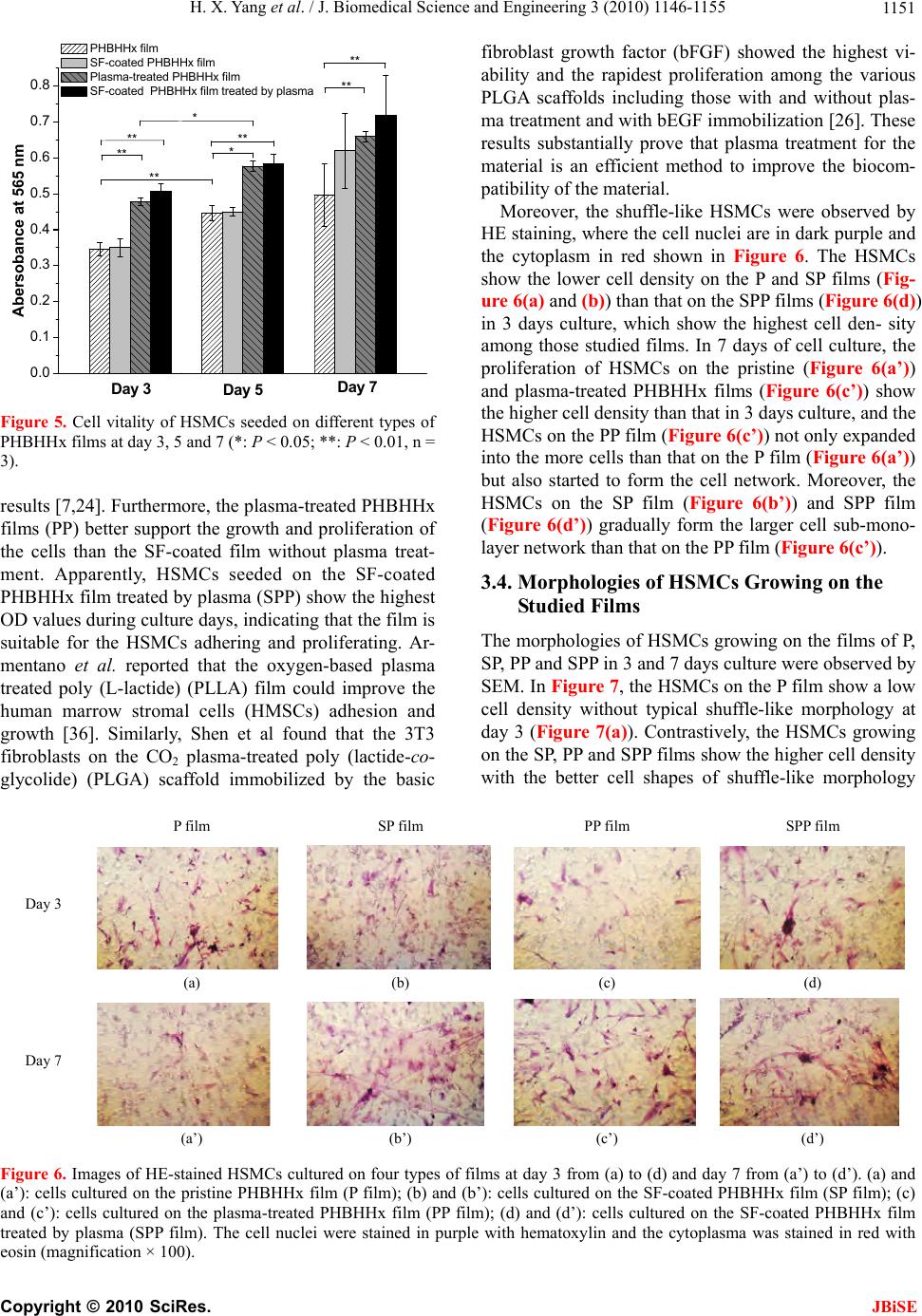 H. X. Yang et al. / J. Biomedical Science and Engineering 3 (2010) 1146-1155 1151 0.0 0.1 0.2 0.3 0.4 0.5 0.6 0.7 0.8 * ** PHBHHx film SF-coated PHBHHx film Plasma-treated PHBHHx film SF-coated PHBHHx film treated by plasma Abersobance at 565 nm Day 3Day 5Day 7 ** ** ** ** ** * Figure 5. Cell vitality of HSMCs seeded on different types of PHBHHx films at day 3, 5 and 7 (*: P < 0.05; **: P < 0.01, n = 3). results [7,24]. Furthermore, the plasma-treated PHBHHx films (PP) better support the growth and proliferation of the cells than the SF-coated film without plasma treat- ment. Apparently, HSMCs seeded on the SF-coated PHBHHx film treated by plasma (SPP) show the highest OD values during culture days, indicating that the film is suitable for the HSMCs adhering and proliferating. Ar- mentano et al. reported that the oxygen-based plasma treated poly (L-lactide) (PLLA) film could improve the human marrow stromal cells (HMSCs) adhesion and growth [36]. Similarly, Shen et al found that the 3T3 fibroblasts on the CO2 plasma-treated poly (lactide-co- glycolide) (PLGA) scaffold immobilized by the basic fibroblast growth factor (bFGF) showed the highest vi- ability and the rapidest proliferation among the various PLGA scaffolds including those with and without plas- ma treatment and with bEGF immobilization [26]. These results substantially prove that plasma treatment for the material is an efficient method to improve the biocom- patibility of the material. Moreover, the shuffle-like HSMCs were observed by HE staining, where the cell nuclei are in dark purple and the cytoplasm in red shown in Figure 6. The HSMCs show the lower cell density on the P and SP films (Fig- ure 6(a) and (b)) than that on the SPP films (Figure 6(d)) in 3 days culture, which show the highest cell den- sity among those studied films. In 7 days of cell culture, the proliferation of HSMCs on the pristine (Figure 6(a’)) and plasma-treated PHBHHx films (Figure 6(c’)) show the higher cell density than that in 3 days culture, and the HSMCs on the PP film (Figure 6(c’)) not only expanded into the more cells than that on the P film (Figure 6(a’)) but also started to form the cell network. Moreover, the HSMCs on the SP film (Figure 6(b’)) and SPP film (Figure 6(d’)) gradually form the larger cell sub-mono- layer network than that on the PP film (Figure 6(c’)). 3.4. Morphologies of HSMCs Growing on the Studied Films The morphologies of HSMCs growing on the films of P, SP, PP and SPP in 3 and 7 days culture were observed by SEM. In Figure 7, the HSMCs on the P film show a low cell density without typical shuffle-like morphology at day 3 (Figure 7(a)). Contrastively, the HSMCs growing on the SP, PP and SPP films show the h igher cell density with the better cell shapes of shuffle-like morphology P film SP film PP film SPP film Day 3 (a) (b) (c) (d) Day 7 (a’) (b’) (c’) (d’) Figure 6. Images of HE-stained HSMCs cultured on four types of films at day 3 from (a) to (d) and day 7 from (a’) to (d’). (a) and (a’): cells cultured on the pristine PHBHHx film (P film); (b) and (b’): cells cultured on the SF-coated PHBHHx film (SP film); (c) and (c’): cells cultured on the plasma-treated PHBHHx film (PP film); (d) and (d’): cells cultured on the SF-coated PHBHHx film treated by plasma (SPP film). The cell nuclei were stained in purple with hematoxylin and the cytoplasma was stained in red with osin (magnification × 100). e Copyright © 2010 SciRes. JBiSE
 H. X. Yang et al. / J. Biomedical Science and Engineering 3 (2010) 1146-1155 1152 (Figure 7 (b), (c) and (d)). At day 7, HSMCs grew into cell sub-monolayer on the surface of PP and SPP films and secreted the extracellular matrix (ECM) to fill up the holes present in the PHBHHx films (Figure 7 (c’) and (d’)), while the HSMCs on the P and SP films show a lower cell confluence and less ECM secretion (Figure 7 (a’) and (b’)). Similarly, Kim et al found that the human bone marrow stromal cells proliferated well to a larger area when attached to th e hydrophilic surfaces than those of the hydrophobic surfaces, resulting in the formation of a more flatten morphology [30]. Also Lampin et al. [29] reported that the increase in the surface roughness of poly (methyl methacrylate) film in extent allowed the expansion of the migration of the chick embryo vascular and corneal cells and triggered the subconfluent cells to secrete the extracellular proteins. Accordingly, in our work, the plasma-treated PHBHHx film improves the roughness (shown in Figure 2) and hydrophilicity (sho- wn in Figure 3), leading to the larger amount of ECM secretion and the better cell migration. 4. CONCLUSION In this work, PHBHHx films were irradiated by the low temperature atmospheric plasma for 6 min, resulting in Day 3 Day 7 PHBHHx (P) (a) (a’) SF-coated PHBHHx (SP) (b) (b’) Copyright © 2010 SciRes. JBiSE
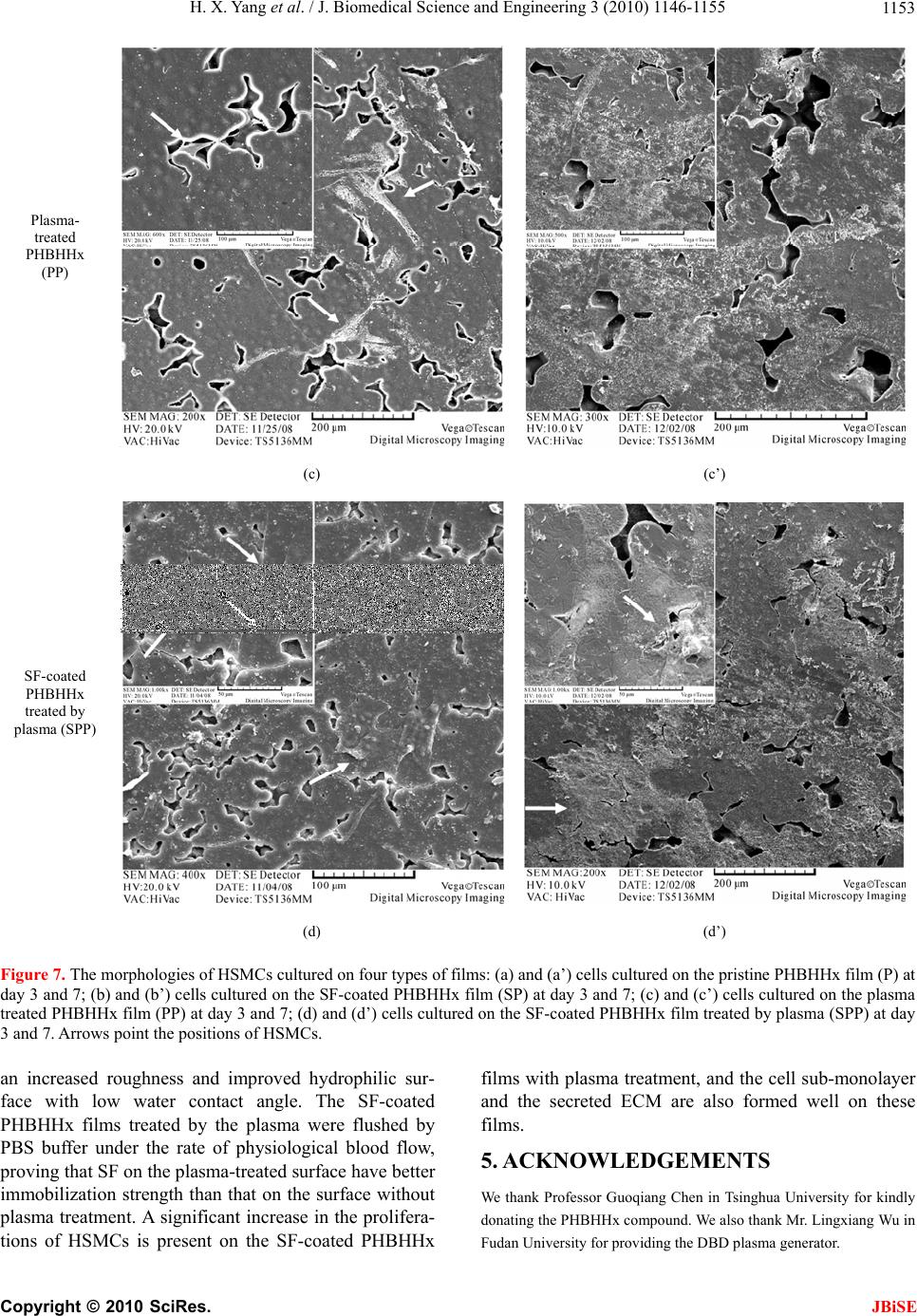 H. X. Yang et al. / J. Biomedical Science and Engineering 3 (2010) 1146-1155 1153 Plasma- treated PHBHHx (PP) (c) (c’) SF-coated PHBHHx treated by plasma (SPP) (d) (d’) Figure 7. The morphologies of HSMCs cultured on four types of films: (a) and (a’) cells cultured on the pristine PHBHHx film (P) at day 3 and 7; (b) and (b’) cells cultured on the SF-coated PHBHHx film (SP) at day 3 and 7; (c) and (c’) cells cultured on the plasma treated PHBHHx film (PP) at day 3 and 7; (d) a nd (d’) cells cultured on the SF-coated PHBHHx film treated by plasma (SPP) at day 3 and 7. Arrows point the positions of HSMCs. an increased roughness and improved hydrophilic sur- face with low water contact angle. The SF-coated PHBHHx films treated by the plasma were flushed by PBS buffer under the rate of physiological blood flow, proving that SF on the plasma-treated surface have better immobilization strength than that on the surface without plasma treatment. A significant increase in the prolifera- tions of HSMCs is present on the SF-coated PHBHHx films with plasma treatment, and the cell sub-monolayer and the secreted ECM are also formed well on these films. 5. ACKNOWLEDGEMENTS We thank Professor Guoqiang Chen in Tsinghua University for kindly donating the PHBHHx compound. We also thank Mr. Lingxiang Wu in Fudan University for providing the DBD plasma generator. Copyright © 2010 SciRes. JBiSE
 H. X. Yang et al. / J. Biomedical Science and Engineering 3 (2010) 1146-1155 1154 REFERENCES [1] Armentano, I. (2009) Role of PLLA plasma surface modification in the interaction with human marrow stro- mal cells. Journal of Applied Polymer Science, 114, 3602-3611. [2] Chen, G., Zhou, P., Mei, N., Chen, X., Shao, Z.Z., Pan, L.F. and Wu, C.G. (2004) Silk fibroin modified porous poly (E-caprolactone) scaffold for human fibroblast cul- ture in vitro. Journal of Materials Science-Materials in Medicine, 15, 671-677. [3] Chen, G.Q. and Wu, Q. (2005) The application of polyhy- droxyalkanoates as tissue engineering materials. Bioma- terials, 26, 6565-6578. [4] Dumitrascu, N., Borcia C. and Borcia G. (2008) Control of the blood-polymer interface by plasma treatment. Journal of Biomedical Materials Research Part B-Ap- plied Biomaterials, 87B, 364-373. [5] Dumitrascu, N., Borcia, G., Apetroaei, N. and Popa, G. (2002) Roughness modification of surfaces treated by a pulsed dielectric barrier discharge. Plasma Sources Sci- ence & Technology, 11, 127-134. [6] Hersel, U., Dahmen, C. and Kessler, H. (2003) RGD modified polymers: biomaterials for stimulated cell ad- hesion and beyond. Biomaterials, 24, 4385-4415. [7] Hoerstrup, S.P. (2000) Functional living trileaflet heart valves grown in vitro. Circulation, 102, 44-49. [8] Khang, G., Kim, S.W., Cho, J.C., Rhee, J.M., Yoon, S.C. and Lee, H.B. (2001) Preparation and characterization of poly (3-hy-droxybutyrate-co-3-hydroxyvalerate) micro- spheres for the sustained release of 5-fluorouracil. Bio- Medical Materials and Engineering, 11, 89-103. [9] Kim, M.S., Shin, Y.N., Cho, M.H., Kim, S.H., Kim, S.K., Cho, Y.H., Khang, G., Lee, I.W. and Lee, H.B. (2007) Adhesion behavior of human bone marrow stromal cells on differentially wettable polymer surfaces. Tissue Engi- neering, 13, 2095-2103. [10] Lampin, M., WarocquierClerout, R., Legris, C, Degrange, M. and SigotLuizard, M.F. (1997) Correlation between substratum roughness and wettability, cell adhesion, and cell migration. Journal of Biomedical Materials Research, 36, 99-108. [11] Langer, R. and Vacanti, J.P. (1993) Tissue Engineering. Science, 260, 920-926. [12] Lee, S.Y. (1996) Bacterial polyhydroxyalkanoates. Bio- technology and Bioengineering, 49, 1-14. [13] Li, X.T., Zhang, Y. and Chen, G.Q. (2008a) Nanofibrous polyhy- droxyalkanoate matrices as cell growth support- ing materials. Biomaterials, 29, 3720-3728. [14] Li, X.T., Sun, J., Chen, S. and Chen, G.Q. (2008b) In vitro investigation of maleated poly (3-hydroxybutyrate- co-3-hydroxyhexanoate) for its biocompatibility to mou- se fibroblast L929 and human microvascular endothelial cells. Journal of Biomedical Materials Research Part A, 87A, 832-842. [15] Li, Z.G., Lin, H., Ishii, N., Chen, G.Q. and Inoue, Y. (2007) Study of enzymatic degradation of microbial co- polyesters consisting of 3-hydroxybutyrate and mdium- chain-length 3-hydroxyalkanoates. Polymer Degradation and Stability, 92, 1708-1714. [16] Mei, N., Chen, G., Zhou, P., Chen, X., Shao, Z.Z., Pan, L.F. and Wu C.G. (2005) Biocompatibility of poly (epsi- lon-caprolac-tone) scaffold modified by chitosan - The fibroblasts proliferation in vitro. Journal of Biomaterials Applications, 19, 323-339. [17] Mei, N., Zhou, P., Pan, L.F., Chen, G., Wu, C.G., Chen, X., Shao, Z.Z. and Chen, G.Q. (2006) Biocompatibility of poly (3-hy-droxybutyrate-co-3-hydroxyhexanoate) modi- fied by silk fibroin. Journal of Materials Science- Mate- rials in Medicine, 17, 749-758. [18] Niklason, L.E., Gao, J., Abbott, W.M., Hirschi, K.K., Houser, S., Marini, R. and Langer, R. (1999) Functional arteries grown in vitro. Science, 284, 489-493. [19] Ostrikov, K. and Murphy, A.B. (2007) Plasma-aided nanofabrication: Where is the cutting edge? Journal of Physics D-Applied Physics, 40, 2223-2241. [20] Robinson, D.E., Marson, A., Short, R.D., Buttle, D.J., Day, A.J., Parry, K.L., Wiles, M., Highfield, P., Mistry, A. and Whittle, J.D. (2008) Surface gradient of functional heparin. Advanced Materials, 20, 1166-1169. [21] Shangguan, Y.Y., Wang, Y.W., Wu, Q. and Chen, G.Q. (2006) The mechanical properties and in vitro biodegra- dation and biocompatibility of UV-treated poly (3-hy- droxybutyrate-co-3-hydroxyhexanoate). Biomaterials, 27, 2349-2357. [22] Shen, H., Hu, X.X., Bei, J.Z., Wang, S.G. (2008) The immobilization of basic fibroblast growth factor on plasma-treated poly (lactide-co-glycolide). Biomaterials, 29, 2388-2399. [23] Shen, H., Hu, X.X., Yang, F., Bel, J.Z. and Wang, S.G. (2007) Combining oxygen plasma treatment with an- chorage of cationized gelatin for enhancing cell affinity of poly (lactide-c o-glycolide) . Biomaterials, 28, 4219-4230. [24] Shishatskaya, E.I., Voinova, O.N., Goreva, A.V., Mogil- naya, O.A. and Volova, T.G. (2008) Biocompatibility of polyhydroxybutyrate microspheres: in vitro and in vivo evaluation. Journal of Materials Science-Materials in Medicine, 19, 2493-2502. [25] Siow, K.S., Britcher, L., Kumar, S. and Griesser, H.J. (2006) Plasma methods for the generation of chemically reactive surfaces for biomolecule immobilization and cell colonization — A review. Plasma Processes and Poly- mers, 3, 392-418. [26] Sodian, R., Hoerstrup, S.P., Sperling, J.S., Daebritz, S., Martin, D.P., Moran, A.M., Kim, B.S., Schoen, F.J., Va- canti, J.P. and Mayer, J.E. (2000) Early in vivo experi- ence with tissue-engineered trileaflet heart valves. Cir- culation, 102, 22-29. [27] Sun, M., Zhou, P., Pan, L.F., Liu, S. and Yang, H.X. (2009) Enhanced cell affinity of the silk fibroin-modified PHBHHx material. Journal of Materials Sc ie nc e -M a t er i al s in Medicine, 20, 1743-1751. [28] Tamada, Y. and Ikada, Y. (1993) Cell-adhesion to plasma-treated polymer surfaces. Polymer, 34, 2208-2212. [29] Wang, Y.W., Wu, Q., Chen, J.C. and Chen, G.Q. (2005) Evaluation of three-dimensional scaffolds made of blends of hydroxyapatite and poly (3-hydroxybutyrate-co-3- hydroxy-hexanoate) for bone reconstruction. Biomate- rials, 26, 899-904. [30] Wu, Q., Wang, Y. and Chen, G.Q. (2009) Medical Appli- cation of Microbial Biopolyesters Polyhydroxyalka- noates. Artificial Cells Blood Substitutes and Biotech- Copyright © 2010 SciRes. JBiSE
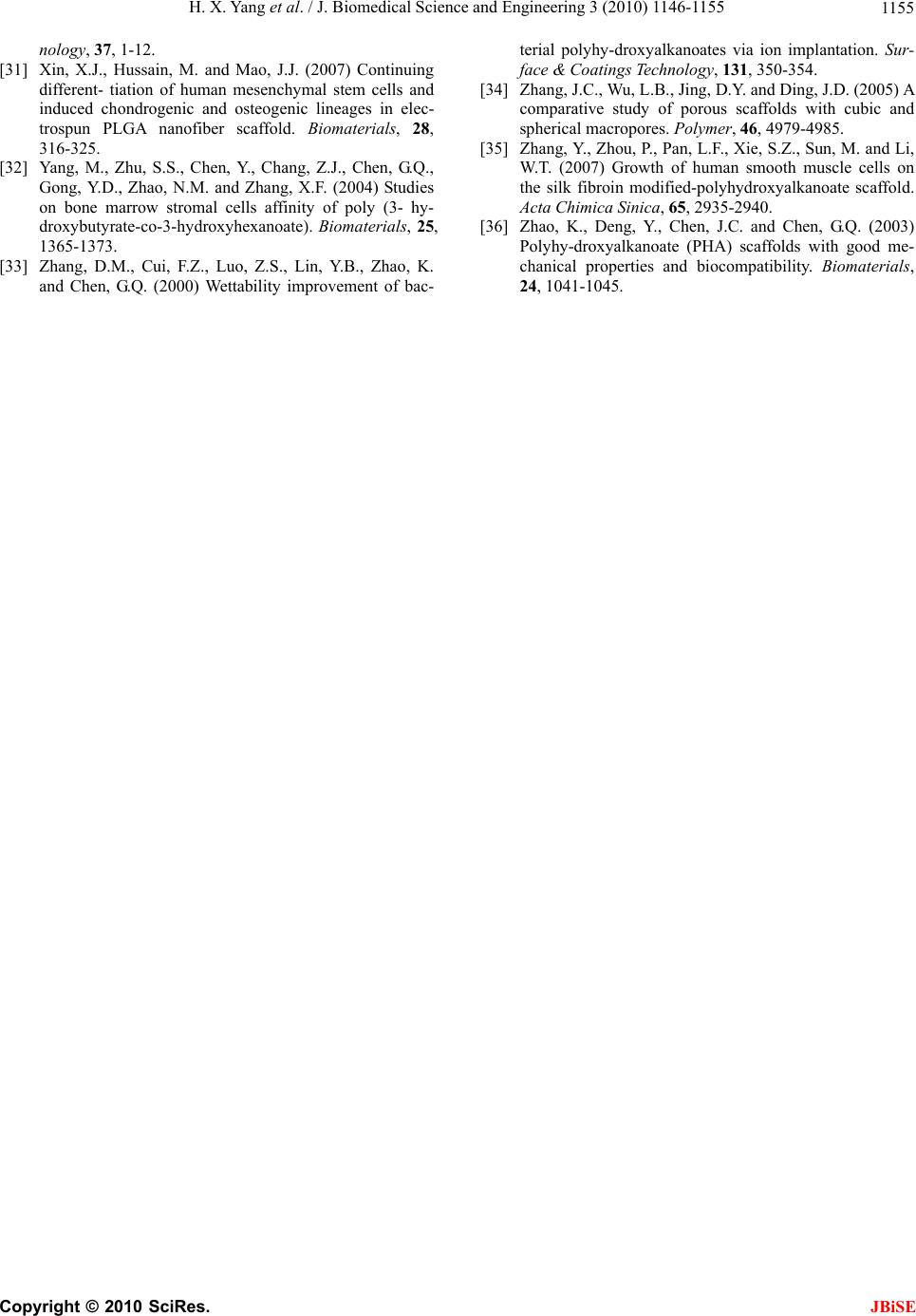 H. X. Yang et al. / J. Biomedical Science and Engineering 3 (2010) 1146-1155 Copyright © 2010 SciRes. 1155 JBiSE nology, 37, 1-12. [31] Xin, X.J., Hussain, M. and Mao, J.J. (2007) Continuing different- tiation of human mesenchymal stem cells and induced chondrogenic and osteogenic lineages in elec- trospun PLGA nanofiber scaffold. Biomaterials, 28, 316-325. [32] Yang, M., Zhu, S.S., Chen, Y., Chang, Z.J., Chen, G.Q., Gong, Y.D., Zhao, N.M. and Zhang, X.F. (2004) Studies on bone marrow stromal cells affinity of poly (3- hy- droxybutyrate-co-3-hydroxyhexanoate). Biomaterials, 25, 1365-1373. [33] Zhang, D.M., Cui, F.Z., Luo, Z.S., Lin, Y.B., Zhao, K. and Chen, G.Q. (2000) Wettability improvement of bac- terial polyhy-droxyalkanoates via ion implantation. Sur- face & Coatings Technology, 131, 350-354. [34] Zhang, J.C., Wu, L.B., Jin g , D.Y. and Ding, J.D. (2005) A comparative study of porous scaffolds with cubic and spherical macropores. Polymer, 46, 4979-4985. [35] Zhang, Y., Zhou, P., Pa n, L.F., Xie, S.Z. , Sun, M. and Li, W.T. (2007) Growth of human smooth muscle cells on the silk fibroin modified-polyhydroxyalkanoate scaffold. Acta Chimica Sinica, 65, 2935-2940. [36] Zhao, K., Deng, Y., Chen, J.C. and Chen, G.Q. (2003) Polyhy-droxyalkanoate (PHA) scaffolds with good me- chanical properties and biocompatibility. Biomaterials, 24, 1041-1045.
|