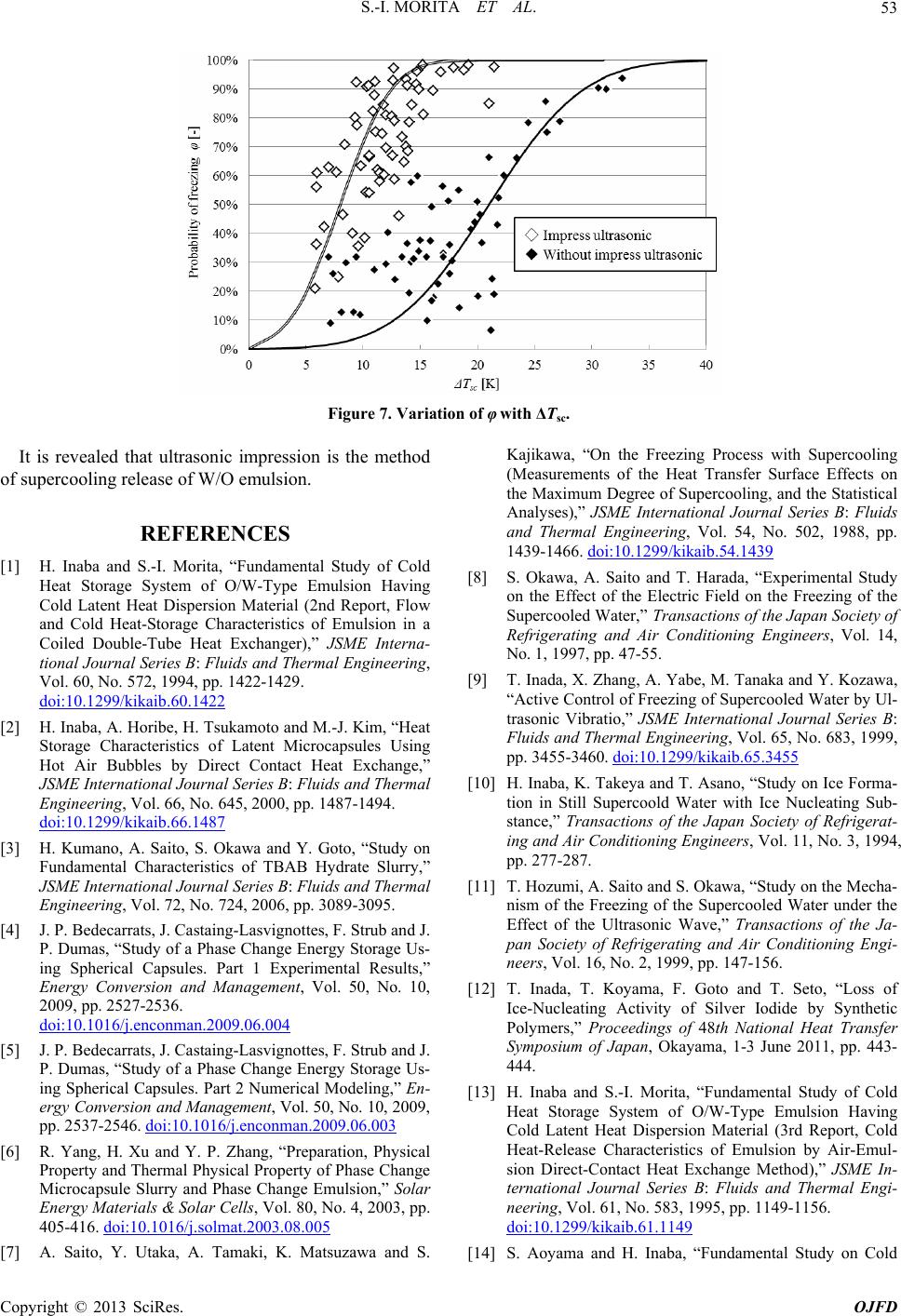
S.-I. MORITA ET AL. 53
Figure 7. Variation of φ with ΔTsc.
It is revealed that ultrasonic impression is the method
of supercooling release of W/O emulsion.
REFERENCES
[1] H. Inaba and S.-I. Morita, “Fundamental Study of Cold
Heat Storage System of O/W-Type Emulsion Having
Cold Latent Heat Dispersion Material (2nd Report, Flow
and Cold Heat-Storage Characteristics of Emulsion in a
Coiled Double-Tube Heat Exchanger),” JSME Interna-
tional Journal Series B: Fluids and Thermal Engineering,
Vol. 60, No. 572, 1994, pp. 1422-1429.
doi:10.1299/kikaib.60.1422
[2] H. Inaba, A. Horibe, H. Tsukamoto and M.-J. Kim, “Heat
Storage Characteristics of Latent Microcapsules Using
Hot Air Bubbles by Direct Contact Heat Exchange,”
JSME International Journal Series B: Fluids and Thermal
Engineering, Vol. 66, No. 645, 2000, pp. 1487-1494.
doi:10.1299/kikaib.66.1487
[3] H. Kumano, A. Saito, S. Okawa and Y. Goto, “Study on
Fundamental Characteristics of TBAB Hydrate Slurry,”
JSME International Journal Series B: Fluids and Thermal
Engineering, Vol. 72, No. 724, 2006, pp. 3089-3095.
[4] J. P. Bedecarrats, J. Castaing-Lasvignottes, F. Strub and J.
P. Dumas, “Study of a Phase Change Energy Storage Us-
ing Spherical Capsules. Part 1 Experimental Results,”
Energy Conversion and Management, Vol. 50, No. 10,
2009, pp. 2527-2536.
doi:10.1016/j.enconman.2009.06.004
[5] J. P. Bedecarrats, J. Castaing-Lasvignottes, F. Strub and J.
P. Dumas, “Study of a Phase Change Energy Storage Us-
ing Spherical Capsules. Part 2 Numerical Modeling,” En-
ergy Conversion and Management, Vol. 50, No. 10, 2009,
pp. 2537-2546. doi:10.1016/j.enconman.2009.06.003
[6] R. Yang, H. Xu and Y. P. Zhang, “Preparation, Physical
Property and Thermal Physical Property of Phase Change
Microcapsule Slurry and Phase Change Emulsion,” Solar
Energy Materials & Solar Cells, Vol. 80, No. 4, 2003, pp.
405-416. doi:10.1016/j.solmat.2003.08.005
[7] A. Saito, Y. Utaka, A. Tamaki, K. Matsuzawa and S.
Kajikawa, “On the Freezing Process with Supercooling
(Measurements of the Heat Transfer Surface Effects on
the Maximum Degree of Supercooling, and the Statistical
Analyses),” JSME International Journal Series B: Fluids
and Thermal Engineering, Vol. 54, No. 502, 1988, pp.
1439-1466. doi:10.1299/kikaib.54.1439
[8] S. Okawa, A. Saito and T. Harada, “Experimental Study
on the Effect of the Electric Field on the Freezing of the
Supercooled Water,” Transactions of the Japan Society of
Refrigerating and Air Conditioning Engineers, Vol. 14,
No. 1, 1997, pp. 47-55.
[9] T. Inada, X. Zhang, A. Yabe, M. Tanaka and Y. Kozawa,
“Active Control of Freezing of Supercooled Water by Ul-
trasonic Vibratio,” JSME International Journal Series B:
Fluids and Thermal Engineering, Vol. 65, No. 683, 1999,
pp. 3455-3460. doi:10.1299/kikaib.65.3455
[10] H. Inaba, K. Takeya and T. Asano, “Study on Ice Forma-
tion in Still Supercoold Water with Ice Nucleating Sub-
stance,” Transactions of the Japan Society of Refrigerat-
ing and Air Conditioning Engineers, Vol. 11, No. 3, 1994,
pp. 277-287.
[11] T. Hozumi, A. Saito and S. Okawa, “Study on the Mecha-
nism of the Freezing of the Supercooled Water under the
Effect of the Ultrasonic Wave,” Transactions of the Ja-
pan Society of Refrigerating and Air Conditioning Engi-
neers, Vol. 16, No. 2, 1999, pp. 147-156.
[12] T. Inada, T. Koyama, F. Goto and T. Seto, “Loss of
Ice-Nucleating Activity of Silver Iodide by Synthetic
Polymers,” Proceedings of 48th National Heat Transfer
Symposium of Japan, Okayama, 1-3 June 2011, pp. 443-
444.
[13] H. Inaba and S.-I. Morita, “Fundamental Study of Cold
Heat Storage System of O/W-Type Emulsion Having
Cold Latent Heat Dispersion Material (3rd Report, Cold
Heat-Release Characteristics of Emulsion by Air-Emul-
sion Direct-Contact Heat Exchange Method),” JSME In-
ternational Journal Series B: Fluids and Thermal Engi-
neering, Vol. 61, No. 583, 1995, pp. 1149-1156.
doi:10.1299/kikaib.61.1149
[14] S. Aoyama and H. Inaba, “Fundamental Study on Cold
Copyright © 2013 SciRes. OJFD