 Chinese Medicine, 2010, 1, 75-83 doi:10.4236/cm.2010.13015 Published Online December 2010 (http://www.SciRP.org/journal/cm) Copyright © 2010 SciRes. CM Inhibitory Actions of Tetrandrine on Tumor Necrosis Factor -Induced NF-B Activation in Neovascularization of Cultured Choroidal Explants* Minoru Kikuchi1, Shusuke Kamimura1, Masaaki Nomura1, Tatsuo Takahashi1, Nobuyoshi Hagino2, Shinjiro Kobayashi1 1Department of Clinical Pharmacy, Faculty of Pharmaceutical Sciences, Hokuriku University, 3-Ho Kanagawa-Machi, Kanazawa, Japan 2Laboratory of Integrative Medicine, US-Japan Cooperative Biomedical Research Laboratories, Tulane University Herbert Research Center and Department of Medicine, Tulane University Health Science Center, School of Medicine, Main Street, Belle Chasse, USA. Email: s-kobayashi@hokuriku-u.ac.jp Received October 13, 2010; revised November 30, 2010; accepted December 2, 2010 Abstract Tetrandrine (1 μM), a bis-benzylisoquinoline alkaloid isolated from Stephania tetrandra S Moore, signifi- cantly decreased tumor necrosis factor alpha (TNFα; 10 ng/ml)-induced increase in the number of micro vessels that budded from cultured rat choroidal explants. Tetrandrine also decreased the TNFα-induced in- crease in the number of cells composing the microvessels. Ammonium pyrrolidine dithiocarbamate (APDC; 0.1-0.3 μM), an inhibitor of nuclear factor-B (NF-κB), decreased the TNFα-induced increase in the number of microvessels in a concentration-dependent manner. TNF increased the phosphorylation and degradation of inhibitor of NF-κB (IκBα), as well as increasing the DNA-binding activity of NF-B in choroidal explants. TNF induced an increase of vascular endothelial growth factor (VEGF)-A mRNA, but not VEGF-C mRNA or VEGF-D mRNA. TNF-induced angiogenic action was inhibited by treatment of VEGF-A antibody in cultured choroidal capillaries. Tetrandrine inhibited the TNFα-induced increases of phosphorylation and degradation of IκBα, and reduced the TNFα-induced increase of DNA-binding activity of NF-κB in chor- oidal explants. In conclusion, tetrandrine inhibits TNF-induced activation of NF-B in the choroidal capil- laries via inhibition of TNFα-induced phosphorylation of IκBα. Keywords: Choroidal Neovascularization, Anti-angiogenesis, Tetrandrine, Tumor Necrosis Factor α, NF-κB Activity, Phosphorylation of IκBα 1. Introduction The bis-benzylisoquinoline alkaloid tetrandrine is a component of Stephania tetrandra S. Moore, which is a herb used in traditional Chinese medicine to treat pa- tients with silicosis, asthma, hypertension and arthritis [1-4]. This alkaloid has been shown to be effective in experimental models of allergic encephalitis, airway mi- crovascular leakage, subcutaneous air pouch inflamma- tion, granuloma angiogenesis in chronic inflammation and proliferation of synovial cells [5-8]. Tetrandrine in- hibits the production and release of a broad range of in lammatory mediators and cytokines [9]. It has been re- ported to block voltage-dependent L-type and T-type Ca2+ channels in various cells, including retinal cells [10], and to inhibit nitric oxide (NO) production in endothelial cells by blocking the Ca2+ release-activated Ca2+ channel [11]. Tetrandrine also inhibits hypoxia-induced neovas- cularization of the small pulmonary artery [12], protects islet beta cells from alloxan-induced injury [13], and prevents spontaneous development of diabetes mellitus in BB rats [14]. Moreover, it has been proposed that Ca2+ flux and NO production in endothelial cells are associ- *A grant (to SK) for the “Academic Frontier” Project for Private Uni- versities (2005-2009) from the Ministry of Education, Culture, Sports, Science and Technology of Japan.
 76 M. KIKUCHI ET AL. ated with angiogenesis induced by vascular endothelial growth factor (VEGF) [15-16]. Administration of tetran- drine suppresses neovascularization of the choroidal cap- illaries, which is facilitated by VEGF and platelet-de- rived growth factor (PDGF)-B in culture [17]. Choroidal tissue in streptozotocin (STZ)-induced diabetic rats ac- cumulates N-(carboxymethyl) lysine (CML), one of the advanced glycation end products (AGEs) (unpublished data). CML facilitates neovascularization of choroidal capillaries in culture [18-21]. Tetrandrine inhibits dia- betic state-induced overproduction of choroidal and reti- nal neovascularization in culture [17,22,23]. Tumor necrosis factor (TNF) is proinflammatory cytokine that has been implicated in the pathogenesis of diabetic retinopathy [24,25]. It is found in the extracellu- lar matrix, endothelium and vessel walls of fibrovascular tissue in the eyes of patients with proliferative diabetic retinopathy [25] and vitreous humor from eyes of pa- tients with diabetic complications [26]. This cytokine has been utilized as a potent promoting factor in in vivo and in vitro angiogenesis models [27-29]. TNF can induce the expression of many important immune-and angio- genesis-related genes through TNF receptors. TNF signaling is required for vessel development in ische- mia-induced neovascularization [30]. The increased ex- pression of many inflammatory proteins is mediated at the level of gene transcription through the activation of proinflammatory transcription factors, including nuclear factor kappa B (NF-B) [31]. In the present study, we investigated the effect of tetrandrine on TNF-induced cell signaling pathways in neovascularization of choroidal capillaries. 2. Materials and Methods 2.1. Materials The chemical structure of tetrandrine is shown in Figure 1. Tetrandrine, fibrinogen and thrombin were from Sigma (St. Louis, MO, USA); -aminocaproic acid, di- methyl sulfoxide, collagenase and ISOGEN were from Wako Pure Chemical Industries Ltd. (Osaka, Japan), dNTPs, RNase inhibitor, M-MLV reverse transcriptase, fetal bovine serum (FBS), oligo dT12-18 primer and Taq Figure 1. Chemical structure of tetrandrine. DNA polymerase were from Invitrogen (Carlsbad, CA, USA); anti-IB antibody and anti-phospho-IB anti- body were from Cell Signaling Technology, Inc. (Bev- erly, MA, USA); TNF and anti-VEGF were from R&D Systems (Minneapolis, MN, USA); ECL Plus western blotting detection system was from GE Health Biosci- ences (Piscataway, NJ, USA); probes for NF-B was from Promega K. K. (Tokyo, Japan); Dulbecco’s modi- fied Eagle’s medium (DMEM) was from Nissui (Tokyo); penicillin/streptomycin was from Lonza (Walkersville, MD, USA); qPCR Mastermix for SYBR® Green I was from Eurogentec (San Diego, CA, USA); ammonium pyrrolidine dithiocarbamate (APDC) was purchased from Dojin (Osaka). 2.2. Culture of Choroidal Tissues All of the animal experiments were performed in accor- dance with the guidelines of the Committee on Animal Experiments in Hokuriku University. Male Wistar rats (7-9 weeks of age) were purchased from Nippon SLC (Shizuoka, Japan). The rats were anaesthetized and killed, and eyeballs were rapidly isolated under aseptic condi- tions. Choroidal explants were isolated under an optical microscope as previously reported [18-20,32]. The size of isolated explants was approximately 0.16 mm2. The explants were plated on fibrin gels prepared by mixing 3 mg fibrinogen (0.3 ml), and 1 U thrombin per ml DMEM containing 30 U/ml penicillin/30 g/ml streptomycin in 24-well plates. Equal volumes of a mixture of the above concentrations of fibrinogen and thrombin were overlaid and allowed to solidify. The explants were cultured with 1% FBS-DMEM (0.5 ml) containing 30 U/ml penicillin/ 30 g/ml streptomycin and 300 g/ml -aminocaproic acid in a CO2 incubator at 37°C for 8 days. In some ex- periments, tetrandrine (0.1 and 1 M), APDC (0.1 and 0.3 M), anti-VEGF antibody (0.3 g/ml) or TNF (10 ng/ml) were added to tissue culture. 2.3. Assessment of Neovascularization The number of micro vessel-like structures newly bud- ded from choroidal explants cultured as described in the previous section was counted under a CKS microscope equipped with a camera (Olympus, Tokyo). The mi- crovessel-like structures have been demonstrated previ- ously to have the features of immature microvessels, consisting of vascular endothelial progenitor cells, such as angioblasts [18]. The number of microvessel-like structures per explant was used as an index of in vitro neovascularization. Choroidal tissue from eyeballs of male Wistar rats (7-9 weeks of age) was sampled with a biopsy punch (1 Copyright © 2010 SciRes. CM
 M. KIKUCHI ET AL. 77 mm; Kai Industries Co. Ltd., Gifu, Japan). The choroidal explants (0.785 mm2) were cultured in fibrin gel with 1% FBS-DMEM containing antibiotics and -aminocaproic acid in a CO2 incubator at 37°C for 8 days as described in the previous section. The primary choroidal explants were removed, and microvessel-like structures were di- gested with 0.75% collagenase (1 ml) in phosphate- buffered saline (PBS) in a CO2 incubator at 37°C for 30 min. The digested cells were transferred to 1.5 ml cen- trifuge tubes, and centrifuged at 16,000 xg for 5 min. Total cell number was counted using a Burker-Turk blood cell counting plate (Erma Inc., Tokyo) under a CKZ microscope (Olympus). 2.4. DNA Binding Activity of NF-κB Choroidal tissues isolated from eyeballs of male Wistar rats (7-9 weeks of age) were cut into pieces of approxi- mately 0.16 mm2, and incubated in 1% FBS-DMEM con- taining TNF (10 ng/ml) with or without tetrandrine (1 M) for 120 min in a CO2 incubator at 37°C. The chor- oidal tissues were lysed with 50 l of buffer A (10 mM HEPES; pH 7.9, 10 mM KCl, 0.1% Triton X-100, 50 M DTT, 1.5 mM MgCl2) for 15 min on ice. After centrifuga- tion at 250 xg for 5 min at 4°C, 20 l of buffer A was added to the pellets, and the mixture was incubated for 5 min on ice and centrifuged again at 8,000xg for 20 min at 4°C. The pellets were incubated in buffer B (20 mM HEPES; pH 7.9, 420 mM NaCl, 1% CA-630, 25% glyc- erol, 500 M DTT, 1.5 mM MgCl2, 0.2 mM EDTA) for 60 min on ice. After centrifugation at 16,000 xg for 5 min at 4°C, the supernatants were harvested as nuclear protein extracts and adjusted to 1 mg/ml proteins. The DNA binding reaction was performed with 3 g of nuclear pro- tein and 15,000 cpm of a 32P-labeled oligonucleotide con- taining NF-B-binding element (5’-AGT TGA GGG GAC TTT CCC AGC C-3’; Promega) as described previ- ously [33,34]. The samples were separated on 5% poly- acrylamide gels, which were analyzed with a Typhoon 9410 image analyzer (Amersham Biosciences). 2.5. Western Blotting Choroidal tissues isolated from eyeballs of male Wistar rats (7-9 weeks of age) were cut into pieces of ap- proximately 0.16 mm2, and incubated in 1% FBS-DMEM containing TNF (10 ng/ml) with or without tetrandrine (1 M) for 5 min in a CO2 incuba- tor at 37°C. The choroidal tissues were extracted with 200 l of triple-deter-gent buffer (50 mM Tris-HCl; pH 8.0, 150 mM NaCl, 0.02% sodium azide, 0.1% sodium dodecyl sulfate (SDS), 100 g/ml phenylmethylsul- fonyl fluoride (PMSF), 1 g/ml aprotinin, 1% Nonidet P-40, 0.5% sodium deoxycholate for 30 min on ice, and homogenized using an UltraS Homogenizer VP-5S (Taitec, Saitama, Japan). After centrifugation at 16,000 xg for 5 min at 4°C, the supernatants were harvested as samples for Western blotting; results were normalized with respect to untreated controls. Western blotting analysis was performed using antibodies against IB and its phosphorylated proteins as described previously [33,34]. Antibody-bound proteins were detected using an ECL-Plus Western Blotting Kit (GE Health Biosci- ences) and analyzed with a Typhoon 9410 image ana- lyzer. 2.6. Real-Time PCR Total RNAs from the cultured choroidal explants were extracted using ISOGEN according to the manufac- turer’s instructions. Then, reverse transcription reaction was performed for 1 h at 37°C in a final volume of 20 l, containing 3 g of total RNA (previously denatured for 10 min at 65°C), 0.5 mM dNTPs, 25 ng/ml oligo dT12-18 primer and 200 units of M-MLV reverse transcriptase. Real-time PCR was optimized with each set of oligonu- cleotide primers, which were designed for rat VEGF-A (Gene Bank accession No. NM_031836; forward, 5’- GCA CTG GAC CCT GGC TTT ACT-3’; reverse, 5’- CGC TGG TAG ACG TCC ATG AA-3’; amplicon size 119 bp), rat VEGF-C (Gene Bank accession No. NM_053653; forward, 5’-CAC AAT CAG TTT TGC CAA TCA CA-3’; reverse, 5’-CAC ACG TAG TTT GCT GGA CAA GTC-3’; amplicon size 127 bp), rat VEGF-D (Gene Bank accession No. AY032728; forward, 5’-CAC CGA GCA GTG AAG GAT GTT-3’; reverse, 5’-CAG TCC TCA GAG TGT GCG ACT T-3’; ampli- con size 124 bp) and rat -actin (Gene Bank accession No. NM_031144; forward, 5’-AGG GAA ATC GTG CGT GAC AT-3’; reverse, 5’-GAA CCG CTC ATT GCC GAT AG-3’; amplicon size 149 bp). Primers were designed with the help of the software Primer Express Ver. 3.0 (Applied Biosystems Japan, Tokyo). The reac- tions were performed using qPCR Mastermix for SYBR® Green I in a 25 l reaction volume containing 10 l of cDNA, 10 pmol of each primer and 12.5 l of the 2x Mastermix in an ABI PRISM 7500 (Applied Biosystems Japan). The program profile used for ampli- fication was: 50°C for 2 min (stage 1), 95°C for 10 min (stage 2), 40 cycles of denaturation at 95°C for 15 sec and annealing at 65°C for 1 min (stage 3), 95°C for 15 sec (stage 4), 60°C for 1 min (stage 5), and 95°C for 15 sec (stage 6). The real-time amplified PCR products were checked on polyacrylamide gel to demonstrate that, un- der the specified real-time conditions, the expected am- p licon sizes are produced. Copyright © 2010 SciRes. CM
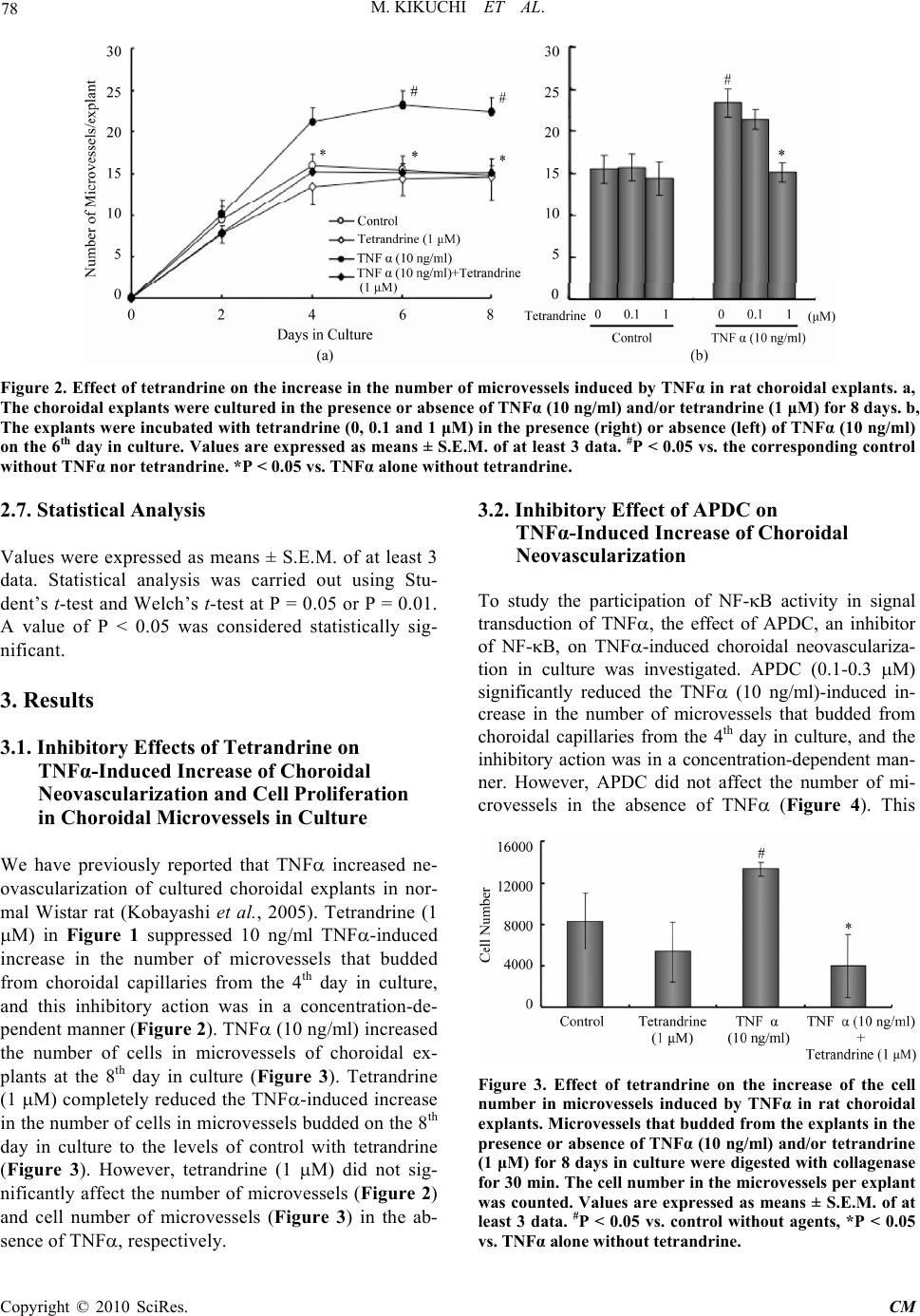 M. KIKUCHI ET AL. Copyright © 2010 SciRes. CM 78 Figure 2. Effect of tetrandrine on the increase in the number of microvessels induced by TNFα in rat choroidal explants. a, The choroidal explants were cultured in the presence or absence of TNFα (10 ng/ml) and/or tetrandrine (1 μM) for 8 days. b, The explants were incubated with tetrandrine (0, 0.1 and 1 μM) in the presence (right) or absence (left) of TNFα (10 ng/ml) on the 6th day in culture. Values are expressed as means ± S.E.M. of at least 3 data. #P < 0.05 vs. the corresponding control without TNFα nor tetrandrine. *P < 0.05 vs. TNFα alone without tetrandrine. 3.2. Inhibitory Effect of APDC on TNFα-Induced Increase of Choroidal Neovascularization 2.7. Statistical Analysis Values were expressed as means ± S.E.M. of at least 3 data. Statistical analysis was carried out using Stu- dent’s t-test and Welch’s t-test at P = 0.05 or P = 0.01. A value of P < 0.05 was considered statistically sig- nificant. To study the participation of NF-B activity in signal transduction of TNF, the effect of APDC, an inhibitor of NF-B, on TNF-induced choroidal neovasculariza- tion in culture was investigated. APDC (0.1-0.3 M) significantly reduced the TNF (10 ng/ml)-induced in- crease in the number of microvessels that budded from choroidal capillaries from the 4th day in culture, and the inhibitory action was in a concentration-dependent man- ner. However, APDC did not affect the number of mi- crovessels in the absence of TNF (Figure 4). This 3. Results 3.1. Inhibitory Effects of Tetrandrine on TNFα-Induced Increase of Choroidal Neovascularization and Cell Proliferation in Choroidal Microvessels in Culture We have previously reported that TNF increased ne- ovascularization of cultured choroidal explants in nor- mal Wistar rat (Kobayashi et al., 2005). Tetrandrine (1 M) in Figure 1 suppressed 10 ng/ml TNF-induced increase in the number of microvessels that budded from choroidal capillaries from the 4th day in culture, and this inhibitory action was in a concentration-de- pendent manner (Figure 2). TNF (10 ng/ml) increased the number of cells in microvessels of choroidal ex- plants at the 8th day in culture (Figure 3). Tetrandrine (1 M) completely reduced the TNF-induced increase in the number of cells in microvessels budded on the 8th day in culture to the levels of control with tetrandrine (Figure 3). However, tetrandrine (1 M) did not sig- nificantly affect the number of microvessels (Figure 2) and cell number of microvessels (Figure 3) in the ab- sence of TNF, respectively. Figure 3. Effect of tetrandrine on the increase of the cell number in microvessels induced by TNFα in rat choroidal explants. Microvessels that budded from the explants in the presence or absence of TNFα (10 ng/ml) and/or tetrandrine (1 μM) for 8 days in culture were digested with collagenase for 30 min. The cell number in the microvessels per explant was counted. Values are expressed as means ± S.E.M. of at least 3 data. #P < 0.05 vs. control without agents, *P < 0.05 s. TNFα alone without tetrandrine. v
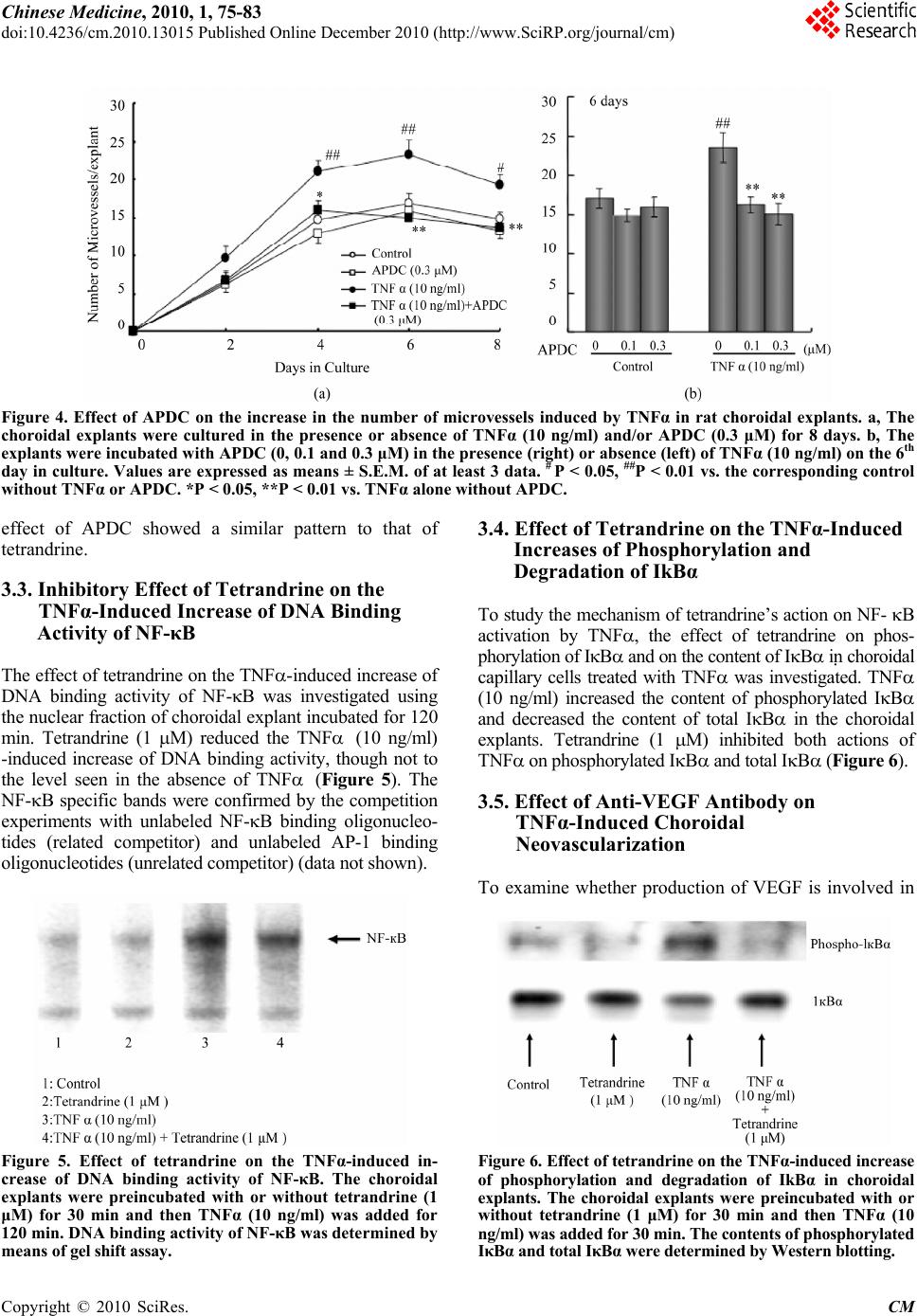 Chinese Medicine, 2010, 1, 75-83 doi:10.4236/cm.2010.13015 Published Online December 2010 (http://www.SciRP.org/journal/cm) Copyright © 2010 SciRes. CM Figure 4. Effect of APDC on the increase in the number of microvessels induced by TNFα in rat choroidal explants. a, The choroidal explants were cultured in the presence or absence of TNFα (10 ng/ml) and/or APDC (0.3 μM) for 8 days. b, The explants were incubated with APDC (0, 0.1 and 0.3 μM) in the presence (right) or absence (left) of TNFα (10 ng/ml) on the 6th day in culture. Values are expressed as means ± S.E.M. of at least 3 data. # P < 0.05, ##P < 0.01 vs. the corresponding control without TNFαor APDC. *P < 0.05, **P < 0.01 vs. TNFα alone without APDC. effect of APDC showed a similar pattern to that of tetrandrine. 3.3. Inhibitory Effect of Tetrandrine on the TNFα-Induced Increase of DNA Binding Activity of NF-κB The effect of tetrandrine on the TNF-induced increase of DNA binding activity of NF-B was investigated using the nuclear fraction of choroidal explant incubated for 120 min. Tetrandrine (1 M) reduced the TNF (10 ng/ml) -induced increase of DNA binding activity, though not to the level seen in the absence of TNF (Figure 5). The NF-B specific bands were confirmed by the competition experiments with unlabeled NF-B binding oligonucleo- tides (related competitor) and unlabeled AP-1 binding oligonucleotides (unrelated competitor) (data not shown). Figure 5. Effect of tetrandrine on the TNFα-induced in- crease of DNA binding activity of NF-κB. The choroidal explants were preincubated with or without tetrandrine (1 μM) for 30 min and then TNFα (10 ng/ml) was added for 120 min. DNA binding activity of NF-κB was determined by means of gel shift assay. 3.4. Effect of Tetrandrine on the TNFα-Induced Increases of Phosphorylation and Degradation of IkBα To study the mechanism of tetrandrine’s action on NF- B activation by TNF, the effect of tetrandrine on phos- phorylation of IB and on the content of IB in choroidal capillary cells treated with TNF was investigated. TNF (10 ng/ml) increased the content of phosphorylated IB and decreased the content of total IB in the choroidal explants. Tetrandrine (1 M) inhibited both actions of TNF on phosphorylated IB and total IB (Figure 6). 3.5. Effect of Anti-VEGF Antibody on TNFα-Induced Choroidal Neovascularization To examine whether production of VEGF is involved in Figure 6. Effect of tetrandrine on the TNFα-induced increase of phosphorylation and degradation of IkBα in choroidal explants. The choroidal explants were preincubated with or without tetrandrine (1 μM) for 30 min and then TNFα (10 ng/ml) was added for 30 min. The contents of phosphorylated IκBα and total IκBα were determined by Western blotting.
 80 M. KIKUCHI ET AL. the TNF-induced increase of choroidal neovasculariza- tion, the effect of anti-VEGF antibody on the neovascu- larization was investigated in culture. Simultaneous treatment of anti-VEGF antibody (0.3 g/ml) signifi- cantly reduced the TNF (10 ng/ml) - induced increase of choroidal neovascularization on the 6th and 8th days in culture (Figure 7). 3.6. Expression of VEGF mRNA during TNFα- Induced Choroidal Neovascularization The levels of mRNAs of VEGF-A, VEGF-C and VEGF- D in choroidal explants were directly measured on the 6th day in culture by means of real-time PCR and nor- malized by -actin mRNA levels. Expression of nor- malized VEGF-A mRNA in choroidal explants was al- most 5-time greater than those of VEGF-C and VEGF-D. TNF (10 ng/ml) significantly increased expression of VEGF-A mRNA 3-time greater. However, TNF unaf- fected VEGF-C mRNA expression and decreased VEGF-D mRNA expression in choroidal explants, re- spectively (Table 1). 4. Discussion The present study was designed to investigate the action of tetrandrine on TNF-induced cell signaling associated with neovascularization of choroidal capillaries in cul- ture. Tetrandrine inhibited 1) the TNF-induced increase of choroidal neovascularization (Figures 2 and 3), 2) the TNF-induced increase of DNA binding activity of Figure 7. Effect of anti-VEGF antibody on the TNFα-in- duced increase in the number of microvessels in choroidal explants in culture. The choroidal explants were cultured in the presence or absence of TNFα (10 ng/ml) and/or anti- VEGF antibody (0.3 μg/ml) for 8 days. Values are expressed as means ± S.E.M. of at least 3 data. ##P < 0.01 vs. the cor- responding control without TNFα or tetrandrine. *P < 0.05, **P < 0.01 vs. TNFα alone without anti-VEGF antibody at the corresponding day. Table 1. mRNA Levels of VEGF-A, VEGF-C and VEGF-D in choroidal explants cultured in the presence of TNFα. VEGF-A VEGF-C VEGF -D TNF 0.1664 ± 0.0237*0.0018 ± 0.0010 0.0008 ± 0.0005* Control0.0531 ± 0.01130.0023 ± 0.0005 0.0025 ± 0.0014 The choroidal explants were cultured in fibrin gel with 1% FBS-DMEM with or without TNFα (10 ng/ml) for 6 days. VEGF-A, VEGF-C and VEGF-D mRNA levels ( △ C) in choroidal explants were determined by means of the protocol for real-time reverse transcription- polymerase chain reaction and normalized by β-actin mRNA levels. Values were expressed as means ± S.E.M. of at least 3 data. *P < 0.05 vs. the corresponding control without TNFα. NF-B (Figure 5), 3) the TNF-induced increase of phosphorylation of IB(Figure 6) and 4) the TNF- induced increase of degradation of IB in choroidal explants (Figure 6). These results indicate that tetran- drine inhibits TNF-induced neovascularization through NF-B inactivation in choroid. The site of action of tetrandrine is upstream of TNF-induced phosphoryla- tionin the process of NF-B activation. Therefore, tetran- drinedecreases translocation of NF-B heterodimers into the nucleus of choroidal capillary cells. This inhibitory action of tetrandrine is similar to that of APDC in chor- oidal neovascularization. APDC prevented release of IB from NF-B in intact cells, but did not inhibit the DNA binding activity in the nucleus in vitro or the inducibility of AP-1 by PMA. APDC has at least two chemical prop- erties that may be related to the inhibition of NF-B ac- tivity: one is a chelating activity for heavy metals, and the second is an antioxidant activity [35]. We have previously reported that nifedipine, an L-type Ca2+ channel blocker, inhibits TNF-induced neovascu- larization of choroidal capillaries [32]. Nifedipine also inhibits basic fibroblast growth factor-induced prolifera- tion of endothelial cells by causing cell-cycle arrest at G0/G1 through blocking the Ca2+ channel [36]. There are also evidences that nifedipine results in increased release of NO [37-38]. It has been proposed that Ca2+ flux and NO production in endothelial cells are associated with angiogenesis induced by VEGF [15-16]. Tetrandrine also blocks voltage-dependent L-type and T-type Ca2+ chan- nels in various cells, including retinal cells [10], and in- hibits NO production in endothelial cells by blocking Ca2+ release-activated Ca2+ channels [11]. The action of tetrandrine on TNF-induced cell signaling in neovas- cularization may therefore be associated with the inhibi- tion of NF-B activity through reducing Ca2+ flux and NO production in choroidal capillaries. Angiogenic ac- tion of TNF has been reported to be associated with Copyright © 2010 SciRes. CM
 M. KIKUCHI ET AL. 81 increased expression of many immune-and angiogene- sis-related genes, mediated by TNF receptors. TNF signaling induces NF-B activation and regulates VEGF expression and consequently VEGF- mediated neovas- cularization [30,39,40]. On the contrary NF-B also in- duces formation of vascular endothelial growth inhibitor (VEGI), which induces apoptosis to proliferating cells while enforcing a growth arrest in quiescent cells [41]. In the present study, TNF accelerated cell proliferation in newly formed microvessels, as well as number of mi- crovessels budded from cultured choroidal capillaries (Figures 2 and 3), indicating that TNF may induce ex- pression of VEGF rather than expression of VEGI in our angiogenesis model of choroidal explants. We have addi- tional data that TNF (10 ng/ml) inhibits cleavage of poly (ADP-ribose) polymerase by caspase-3 (data not shown), supporting that TNF is angiogenic activator but not inducer of apoptosis in the choroidal explants. The angiogenic action of TNF on choroidal neovascu- larization was inhibited by APDC, an inhibitor of NF-B [35] (Figure 4), supporting that NF-B is involved in the mechanism of TNF’s action on choroidal neovascu- larization. The present investigations also indicated that TNF facilitated phosphorylation of IBand degrada- tion (decrease of content) of IB (Figure 6). The deg- radation of IB results from release of NF-B het- erodimers to translocate to the nucleus, where they bind to nuclear DNA (Figure 5), leading to activation of spe- cific subsets of angiogenic genes in the choroidal ex- plants. We have now plans to identify cellular sources of NF-B and I-B in our choroidal explant lysates. The present study directly demonstrates that TNF increases expression of mRNA of VEGF-A, but not VEGF-C or VEGF-D in choroid (Table 1). Angiogenic action of TNF was reduced by anti-VEGF-A antibody in choroidal explant (Figure 7). These results indicate that TNF stimulates the production and release of VEGF-A via activation of NF-B in choroidal explant and increases neovascularization. VEGF-C and VEGF-D interact with VEGF receptor (VEGFR)-2 and VEGFR-3 and have a role for the growth of lymphatic vessels [42]. TNF did not increase mRNA of VEGF-C and VEGF-D, suggesting that TNF does not increase lymph angio- genesis. Tetrandrine (1 M) inhibited TNF-induced cell signaling of neovascularization in cultured choroidal explant. However, this concentration of tetrandrine did not affect basal neovascularization or cell proliferation in the absence of TNF (Figures 2 and 3). Tetrandrine (1 M) inhibits VEGF-induced neovascularization in chor- oidal explant [17], indicating that tetrandrine reduces both of TNF-induced release of VEGF-A and the action of VEGF-A in neovascularization of choroidal explant. 5. Conclusion Tetrandrine inhibits TNF-induced activation of NF-B in the choroidal capillaries via inhibition of TNF-in- duced phosphorylation of IBin choroidal neovascu- larization. 6. Acknowledgements This work was supported in part by a grant (to SK) for the “Academic Frontier” Project for Private Universities (2005-2009) from the Ministry of Education, Culture, Sports, Science and Technology of Japan, Japan. 7. References [1] X. X. Yu, J. X. Dang, L. Shi, P. F. Gu, X. H. Xu and F. Z. Kong, “Clinical Observation of Tetrandrine Aero Sol on the Effect of Relieving Asthma,” Zhejiang Journal Tradi- tional Chinese Medicine, Vol. 27, 1992, pp. 98-99. [2] D. Li, H. Lu, X. Li, Q. Ouan, X. Li and W. Lu, “Calcium Channel Blockers in Cirrhotic Patients with Portal Hy- pertension,” Chinese Medicine Journal (Engl), Vol. 108, 1995, pp. 803-808. [3] D. H. Li, C. J. Zhang and Y. N. Zhong, “Clinical Study on Long-Term Therapeutic Effect of Combined Anti-Fibrosis, 2001,” Chin J Indus Hygiene Occup Dis , Vol. 19, 2001, pp. 3-6. [4] Q. M. Xie, H. F. Tang, J. Q. Chen and R. L. Bian, “Phar- macological Actions of Tetrandrine in Inflammatory Pul- monary Disease,” Acta Pharmacologica Sinica, Vol. 23, 2002, pp. 1107-1113. [5] C. W. Wong, W. K. Seow, J. W. O’Callaghan and Y. H. Thong, “Comparative effects of Tetrandrine and Berbamine on Subcutaneous Air Pouch Inflammation Induced by In- terleukin-1, Tumor Necrosis Factor and Platelet-Activating Factor,” Agents Actions, Vol. 36, 1992, pp. 112- 118. [6] C. W. Wong, Y. H. Thong and W. K. Seow, “Compara- tive Effects of Tetrandrine and Berbamine on Guinea Pig Airway Micro Vascular Leakage Induced by Plate- let-Activating Factor and Other Allergic Mediators,” In- ternational Journal of Immunopharmacology, Vol. 15, 1993, pp. 185-193. [7] K. Takahashi, H. Kobayashi, S. Kobayashi, I. Kimura, K. Terasawa and M. Kimura, “Antiproliferative Effects of Magnosalin Derived from ‘Shin’i’ (Flos Magoliae), a Japanese Sino-Medicine, on Cultured Synovial Cells of MRL/l and C57BL/6J Mice,” Phototherapy Research, Vol. 10, 1996, pp. 42-48. [8] S. Kobayashi, K. Inaba, I. Kimura and M. Kimura, “In- hibitory Effects of Tetrandrine on Angiogenesis in Adju- vant-Induced Chronic Inflammation and Tube Formation of Vascular Endothelial Cells,” Biological and Pharma- ceutical Bullet in, Vol. 21, 1998, pp. 346-349. [9] B. S. Teh, W. K. Seow, S. Y. Li and Y. H. Thong, “Inhi- bition of Prostaglandin and Leukotriene Generation by Copyright © 2010 SciRes. CM
 82 M. KIKUCHI ET AL. the Plant Alkaloids Tetrandrine and Berbamine,” Interna- tional Journal of Immunopharmacology Vol. 12, 1990, pp. 321- 326. [10] G. Wang and J. R. Lemos, “Tetrandrine: A New Ligand to Block Voltage-Dependent Ca2+ and Ca(2+)-Activated K+ Channels,” Life Sciences, Vol. 56, 1995, pp. 295-306. [11] C. Y. Kwan, F. M. Ma and S. C. Hui, “Inhibition of En- dothelium-Dependent Vascular Relaxation by Tetran- drine,” Life Science s, Vol. 64, 1999, pp. 2391-2400. [12] W. Li, W. Chen, S. Zhang and S. Lei, “Effect of Tetran- drine on Pulmonary Vascular Morphology in Rats with Hypoxia Oulmonary Hypertension,” Journal of West China University Medical Sciences, Vol. 28, 1997, pp. 389-391. [13] G. Sun, Y. Qi and Q. Pan, “Quantitative Analysis of Pre- vention Effect of Tetrandrine on Pancreatic Islet Beta Cells Injury in Rats,” Chinese Medical Journal, Vol. 77, 1997, pp. 270-277. [14] I. Lieberman, D. P. Lentz, G. A. Trucco, W. K. Seow and Y. H. Thong, “Prevention by Tetrandrine of Spontaneous Development of Diabetes Mellitus in BB Rats,” Diabetes, Vol. 41, 1992, pp. 616-619. [15] D. O. Bates and F. E. Curry, “Vascular Endothelial- growth Factor Increases Microvascular Permeability via A Ca(2+)-Dependent Pathway,” American Journal of Physiology Vol. 273, 1997, pp. H687-H694. [16] K. S. Bauer, K. J. Cude, S. C. Dixon, E. A. Kruger and W. D. Figg, “Carboxyamidotriazole Inhibits Angiogenesis by Blocking the Calcium-Mediated Nitric-Oxide Synthase- Vascular Endothelial Growth Factor Pathway,” The Jour- nal of Pharmacology and Experimental Therapeutics, Vol. 292, 2000, pp. 31-37. [17] S. Kobayashi, I. Kimura, M. Fukuta, H. Kontani, K. Inaba, M. Niwa, S. Mita and M. Kimura, “Inhibitory Ef- fects of Tetrandrine and Its Related Synthetic Compounds on Angiogenesis in Streptozotocin-Diabetic Rodent,” Biological and Pharmaceutical Bulletin, Vol. 22, 1999, pp. 360-365. [18] S. Kobayashi, H. Shinohara, H. Tsuneki, R. Nagai, and S. Horiuchi, “N-(Carboxymethyl) Lysine Proliferates CD34+ Cells from Rat Choroidal Explant in Culture,” Biological and Pharmaceutical Bulletin, Vol. 27, 2004, pp. 1382-1387. [19] S. Kobayashi, M. Suzuki, H. Tsuneki, R. Nagai, S. Horiu- chi and N. Hagino, “Overproduction of N- (Carboxy- methyl) Lysine-Induced Neovascularization in Cultured Choroidal Explant of Streptozotocin-Diabetic Rat,” Bio- logical and Pharmaceutical Bulletin, Vol. 27, 2004, pp. 1565-1571. [20] S. Kobayashi, M. Nomura, T. Nishioka, M. Kikuchi, A. Ishihara, R. Nagai and N. Hagino, “Overproduction of N-(carboxymethyl) Lysine-Induced Neovascularization in Cultured Choroidal Explant of Aged Rat,” Biological and Pharmaceutical Bulletin, Vol. 30, 2007, pp. 133-138. [21] S. Kobayashi, M. Nomura, T. Takahashi, M. Suzuki, R. Nagai and N. Hagino, “N-(Carboxymethyl) Lysine- In- duced Choroidal Angiogenic Potential Facilitates Retinal Neovascularization in Advanced-Diabetic Rat in Vitro,” the Open Pharmacology Journal, Vol. 2, 2008, pp. 79-86. [22] X- C. Liang, N. Hagino, S- S., Guo, T. Tsutsumi and S. Kobayashi, “Therapeutic Efficacy of Stephania tetrandra S. Moore for Treatment of Neovascularization of Retinal Capillary (Retinopathy) in Diabetes-in Vitro Study,” Phy- tomedicine, Vol. 9, 2002, pp. 377-384. [23] T. Tsutsumi, N. Hagino, X- C. Liang, S- S. Guo and S. Kobayashi, “Effects of Oral Administration of Stephania tetrandra S. Moore on Neovascularization of Retinal and Choroidal Capillaries of Diabetes in Rats,” Phototherapy Research, Vol. 22, 2008, pp 591-596. [24] K. Hawrami, G. A. Hitman, M. Rema, C. Snehalatha, M. Viswanathan, A. Ramachandran and V. Mohan, “An As- sociation in Non-Insulin-Dependent Diabetes Mellitus Subjects between Susceptibility,” Human Immunology, Vol. 46, 1996, pp. 49-54. [25] G. A. Limb, A. H. Chignell, W. Green, F. LeRoy and D. C. Dumonde, “Distribution of TNF Alpha and Its Reac- tive Vascular Adhesion Molecules in Fibrovascular,” British Journal of Ophthalmology, Vol. 80, 1996, pp. 168-173. [26] J. Spranger, R. Meyer-Schwickerath, M. Klein, H. Schatz and A. Pfeiffer, “TNF-Alpha Level in the Vitreous Body. Increase in Neovascular Eye Diseases and Proliferative Diabetic Retinopathy,” Medizinische Klinik (Munich, Germany), Vol. 90, 1995, pp. 134-137. [27] M. Frater-Schroder, W. Risau, R. Hallmann, P. Gautschi and P. Bohlen, “Tumor Necrosis Factor Type Alpha, A Potent Inhibitor of Endothelial Cell Growth in Vitro, is Angiogenic in Vivo,” Proceedings of National Academy of Sciences of the United States of Am erica, Vol. 84, 1987, pp. 5277-5281. [28] S. J. Leibovich, P. J. Polverini, H. M. Shepard, D. M. Wiseman, V. Shively, N. Nuseir, “Macrophage-Induced Angiogenesis is Mediated by Tumor Necrosis Fac- tor-Alpha,” Nature, Vol. 329, 1987, pp. 630-632. [29] A. Passaniti, R. M. Taylor, R. Pili, Y. Guo, P. V. Long, J. A. Haney, R. R. Pauly, D. S. Grant and G. R. Martin, “A Simple, Quantitative Method For Assessing Angiogenesis and Antiangiogenic Agents Using Reconstituted Base- ment Membrane, Heparin, and Fibroblast Growth Fac- tor,” Laboratory Investigation, Vol. 67, 1992, pp. 519-28. [30] D. V. Goukassian, G. Qin, C. Dolan, T. Murayama, M. Silver, C. Curry, E. Eaton, C. Luedemann, H. Ma, T. Asahara, V. Zak, S. Mehta, A. Burg, T. Thorne, R. Kishore and D. W. Losordo, “Tumor Necrosis Factor-α Receptor P75 is Required in Ischemia-Induced Neovascu- larization,” Circulation, Vol. 115, 2007, pp. 752-762. [31] C. Granet, W. Maslinski and P. Miossec, “Increased AP-1 and NF-κB Activation and Recruitment with the Combi- nation of the Proinflammatory Cytokines IL-17, Tumor Necrosis Factor Alpha and IL-17 in Rheumatoid Syno- viocytes,” Arthritis Research Therapy, Vol. 6, 2004, pp. Copyright © 2010 SciRes. CM
 M. KIKUCHI ET AL. Copyright © 2010 SciRes. CM 83 R190-R198. [32] S. Kobayashi, M. Fukuta, M. Suzuki, H. Tsuneki and I. Kimura, “Inhibitory Effect of Nifedipine on Tumor Ne- crosis Factor -Induced Neovascularization in Cultured Choroidal Explants of Streptozotocin-Diabetic Rat,” Bio- logical and Pharmaceutical Bulletin, Vol. 28, 2005, pp. 242-246. [33] M. Nomura, W. Ma, N. Chen, A. M. Bode and Z. Dong, “Inhibition of 12-O-Tetradecanoyl Phorbol-13-Acetate- Induced NF-Kappab Activation by Tea Polyphenols, (-)- Epigallocatechin Gallate and Theaflavins,” Carcinogene- sis, Vol. 21, 2000, pp 1885-1890. [34] D. Ichimatsu, M. Nomura, S. Nakamura, S. Moritani, K. Yokogawa, S. Kobayashi, T. Nishioka, and K. Miyamoto, “Structure-Activity Relationship of Flavonoids for Inhi- bition of Epidermal Growth Factor-Induced Transforma- tion of JB6 Cl 41 Cells,” Molecular Carcinogenesis, Vol. 46, 2007. pp. 436-445. [35] R. Schreck, B. Meier, D. N. Mannel, W. Droge and P. A. Baeuerle, “Dithiocarbamates as Potent Inhibitors of Nu- clear Factor kB Activation in Intact Cells,” Journal of Experimental Medicine, Vol. 175, pp. 1181-1191. [36] H. Zeitler, Y. Ko, B. Glodny, G. Totzke, M. Appen- heimer, A. Sachinidis and H. Vetter, “Cell-Cycle Arrest in G0/ G1 Phase of Growth Factor-Induced Endothelial Cell Proliferation by Various Calcium Channel Block- ers,” Cancer Detection and Prevention, Vol. 21, 1997, pp. 332-339. [37] R. Berkels, G. Egink, T. A. Marsen, H. Bartels, R. Roe- sen and W. Klaus, “Nifedipine Increases Endothelial Ni- tric Oxide Bioavailability by Antioxidative Mechanisms,” Hypertension, Vol. 37, 2001, pp. 240-245. [38] J. P. Cooke, “NO and Angiogenesis,” Atherosclerosis Supplement, Vol.4, 2003, pp. 53-60. [39] S. Yoshida, M. Ono, T. Shono, H. Izumi, T. Ishbashi, H. Suzuki and M. Kuwano, “Involvement of Interleukin-8, Vascular Endothelial Growth Factor, and Basic Fibroblast Growth Factor in Tumor Necrosis Factor-Alpha- De- pendent Angiogenesis,” Molecular and Cellular Biology. Vol. 17, 1997, pp. 4015-4023. [40] Z Zhou, M. C. Connell and D. J. MacEwan, “TNFR1- Induced NF-B, but Not ERK, P38mapk or JNK Activa- tion, Mediates TNF-Induced ICAM-1 and VCAM-1 Ex- pression on Endothelial Cell,” Cellular Signaling, Vol. 19, 2007, pp. 1238-1248. [41] J. Yu, S. Tian, L. Metheny-Barlow, L. J. Chew, A. J. Hayes, H. Pan, G. L. Yu and L. Y. Li, “Modulation of Endothelial Cell Growth Arrest and Apoptosis by Vascu- lar Endothelial Growth Inhibitor,” Circulation Research, Vol. 89, 2001, pp. 1161-1167. [42] K. Alitalo, T. Tammela and T. V. Petrova, “Lymphan- giogenesis in Development and Human Disease,” Nature, Vol. 438, 2005, pp. 946-953.
|