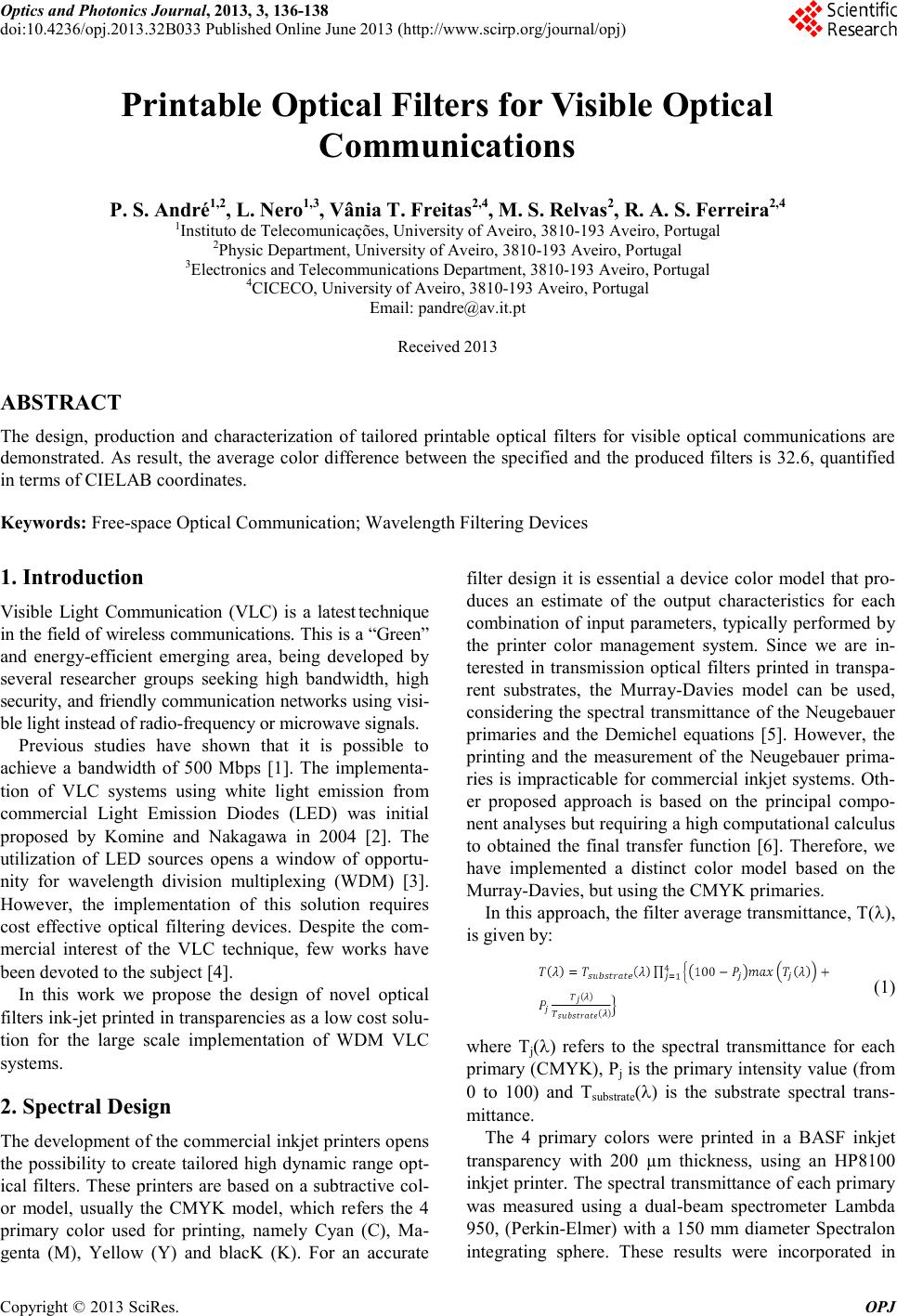
Optics and Photonics Journal, 2013, 3, 136-138
doi:10.4236/opj.2013.32B033 Published Online June 2013 (http://www.scirp.org/journal/opj)
Copyright © 2013 SciRes. OPJ
Printable Optical Filters for Visible Optical
Communications
P. S. André1,2, L. Nero1,3, Vânia T. Freitas2,4, M. S. Relvas2, R. A. S. Ferreira2,4
1Instituto de Telecomunicações, University of Aveiro, 3810-193 A v e i ro, Port ugal
2Physic Depar tment, U niversity of Aveiro, 3810-193 Aveiro, Portugal
3Electronics and Telecommunications Department, 3810-193 Avei ro, Por tugal
4CICECO, University of Aveiro, 3810-193 Aveiro, Portugal
Email: pandre@av.it.pt
Received 2013
ABSTRACT
The design, production and characterization of tailored printable optical filters for visible optical communications are
demonstrated. As result, the average color difference between the specified and the produced filters is 32.6, quantified
in terms of CIELAB coordinates.
Keywords: Free-space Optical Communication; Wavelen gth Filterin g Devices
1. Introduction
Visible Light Communication (VLC) is a latest technique
in the field of wirele ss commu nications. T his is a “Gree n”
and energy-efficient emerging area, being developed by
several researcher groups seeking high bandwidth, high
sec urity, a nd frie ndly co mmun icatio n networ ks usi ng visi-
ble light instead of radio-frequ enc y or microwave signals.
Previous studies have shown that it is possible to
achieve a bandwidth of 500 Mbps [1]. The implementa-
tion of VLC systems using white light emission from
commercial Light Emission Diodes (LED) was initial
proposed by Komine and Nakagawa in 2004 [2]. The
utilization of LED sources opens a window of opportu-
nity for wavelength division multiplexing (WDM) [3].
However, the implementation of this solution requires
cost effective optical filtering devices. Despite the com-
mercial interest of the VLC technique, few works have
been devoted to the subject [4].
In this work we propose the design of novel optical
filters i nk-jet printed in transparencies as a low cost solu-
tion for the large scale implementation of WDM VLC
systems.
2. Spectral Design
The development of the commercial inkjet printers opens
the possibility to create tailored high dynamic range opt-
ical filters. These printers are based on a subtractive col-
or model, usually the CMYK model, which refers the 4
primary color used for printing, namely Cyan (C), Ma-
genta (M), Yellow (Y) and blacK (K). For an accurate
filter design it is essential a device color model that pro-
duces an estimate of the output characteristics for each
combination of input parameters, typically performed by
the printer color management system. Since we are in-
terested in transmission optical filters printed in transpa-
rent substrates, the Murray-Davies model can be used,
considering the spectral transmittance of the Neugebauer
primaries and the Demichel equations [5]. However, the
printing and the measurement of the Neugebauer prima-
ries is impracticable for commercial inkjet systems. Oth-
er proposed approach is based on the principal compo-
nent a na l yse s b ut r e qui ri n g a hig h c o mp uta ti onal c a lculus
to obtained the final transfer function [6]. Therefore, we
have implemented a distinct color model based on the
Murray-Davies, but using the CM YK pri maries.
In this approach, the filter average transmittance, T(λ),
is given by:
(1)
where Tj(λ) refers to the spectral transmittance for each
primary (CMYK), Pj is the p rimary intensit y value (fro m
0 to 100) and Tsubstrate(λ) is the substrate spectral trans-
mittance.
The 4 primary colors were printed in a BASF inkjet
transparency with 200 µm thickness, using an HP8100
inkjet printer. The spectral transmittance of each primary
was measured using a dual-beam spectrometer Lambda
950, (Perkin-Elmer) with a 150 mm diameter Spectralon
integrating sphere. These results were incorporated in