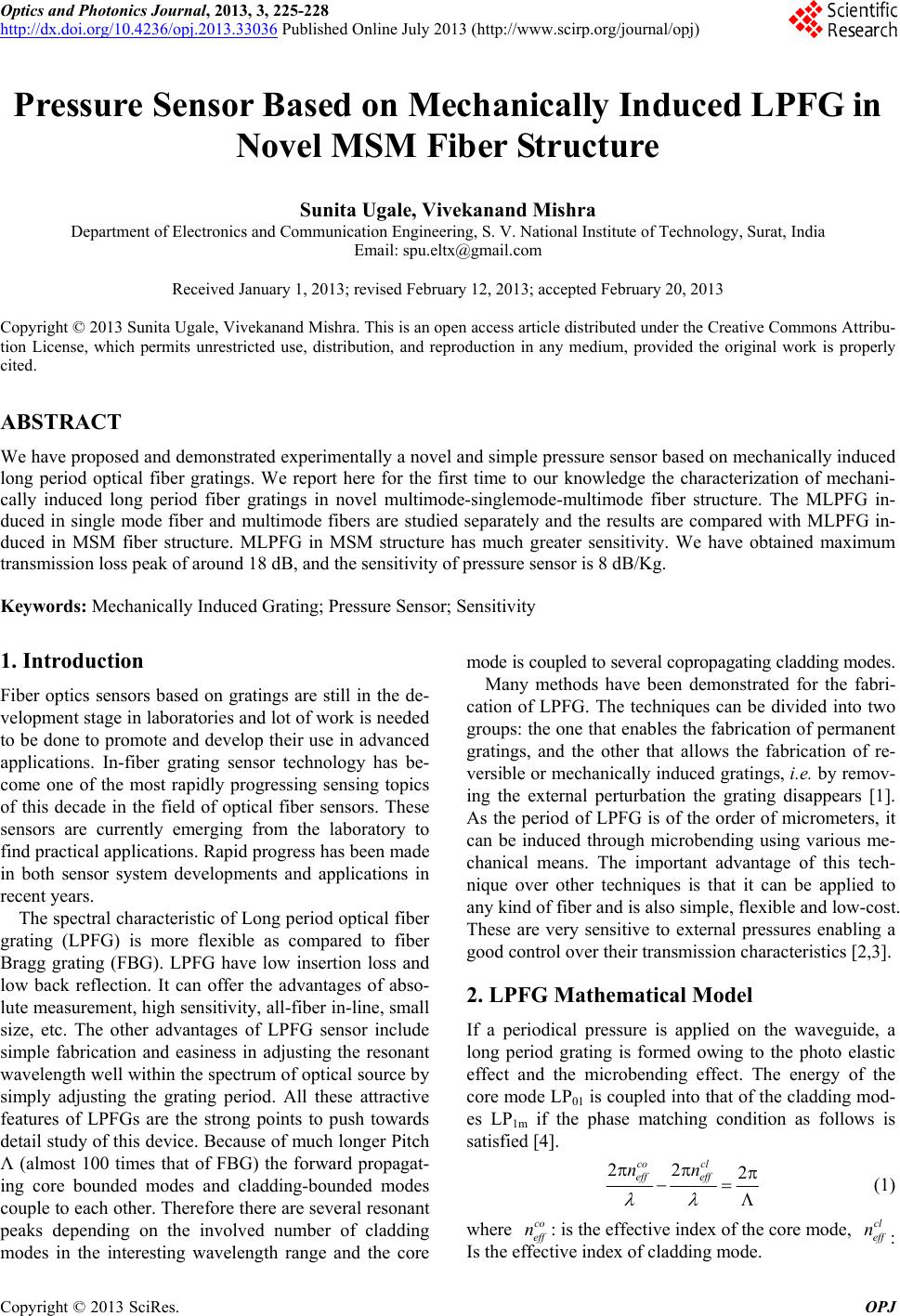
Optics and Photonics Journal, 2013, 3, 225-228
http://dx.doi.org/10.4236/opj.2013.33036 Published Online July 2013 (http://www.scirp.org/journal/opj)
Pressure Sensor Based on Mechanically Induced LPFG in
Novel MSM Fiber Structure
Sunita Ugale, Vivekanand Mishra
Department of Electronics and Communication Engineering, S. V. National Institute of Technology, Surat, India
Email: spu.eltx@gmail.com
Received January 1, 2013; revised February 12, 2013; accepted February 20, 2013
Copyright © 2013 Sunita Ugale, Vivekanand Mishra. This is an open access article distributed under the Creative Commons Attribu-
tion License, which permits unrestricted use, distribution, and reproduction in any medium, provided the original work is properly
cited.
ABSTRACT
We have proposed and demonstrated experimentally a novel and simple pressure sensor based on mechanically induced
long period optical fiber gratings. We report here for the first time to our knowledge the characterization of mechani-
cally induced long period fiber gratings in novel multimode-singlemode-multimode fiber structure. The MLPFG in-
duced in single mode fiber and multimode fibers are studied separately and the results are compared with MLPFG in-
duced in MSM fiber structure. MLPFG in MSM structure has much greater sensitivity. We have obtained maximum
transmission loss peak of around 18 dB, and the sensitivity of pressure sensor is 8 dB/Kg.
Keywords: Mechanically Induced Grating; Pressure Sensor; Sensitivity
1. Introduction
Fiber optics sensors based on gratings are still in the de-
velopment stage in laboratories and lot of work is needed
to be done to promote and develop their use in advanced
applications. In-fiber grating sensor technology has be-
come one of the most rapidly progressing sensing topics
of this decade in the field of optical fiber sensors. These
sensors are currently emerging from the laboratory to
find practical applications. Rapid progress has been made
in both sensor system developments and applications in
recent years.
The spectral characteristic of Long period optical fiber
grating (LPFG) is more flexible as compared to fiber
Bragg grating (FBG). LPFG have low insertion loss and
low back reflection. It can offer the advantages of abso-
lute measurement, high sensitivity, all-fiber in-line, small
size, etc. The other advantages of LPFG sensor include
simple fabrication and easiness in adjusting the resonant
wavelength well within the spectrum of optical source by
simply adjusting the grating period. All these attractive
features of LPFGs are the strong points to push towards
detail study of this device. Because of much longer Pitch
Λ (almost 100 times that of FBG) the forward propagat-
ing core bounded modes and cladding-bounded modes
couple to each other. Therefore there are several resonant
peaks depending on the involved number of cladding
modes in the interesting wavelength range and the core
mode is coupled to several copropagating cladding modes.
Many methods have been demonstrated for the fabri-
cation of LPFG. The techniques can be divided into two
groups: the one that enables the fabrication of permanent
gratings, and the other that allows the fabrication of re-
versible or mechanically induced gratings, i.e. by remov-
ing the external perturbation the grating disappears [1].
As the period of LPFG is of the order of micrometers, it
can be induced through microbending using various me-
chanical means. The important advantage of this tech-
nique over other techniques is that it can be applied to
any kind of fiber and is also simple, flexible and low-cost.
These are very sensitive to external pressures enabling a
good control over their transmission characteristics [2,3].
2. LPFG Mathematical Model
If a periodical pressure is applied on the waveguide, a
long period grating is formed owing to the photo elastic
effect and the microbending effect. The energy of the
core mode LP01 is coupled into that of the cladding mod-
es LP1m if the phase matching condition as follows is
satisfied [4].
22 2
co cl
eff eff
nn
(1)
where : is the effective index of the core mode, :
Is the effective index of cladding mode.
co
eff
ncl
eff
n
C
opyright © 2013 SciRes. OPJ