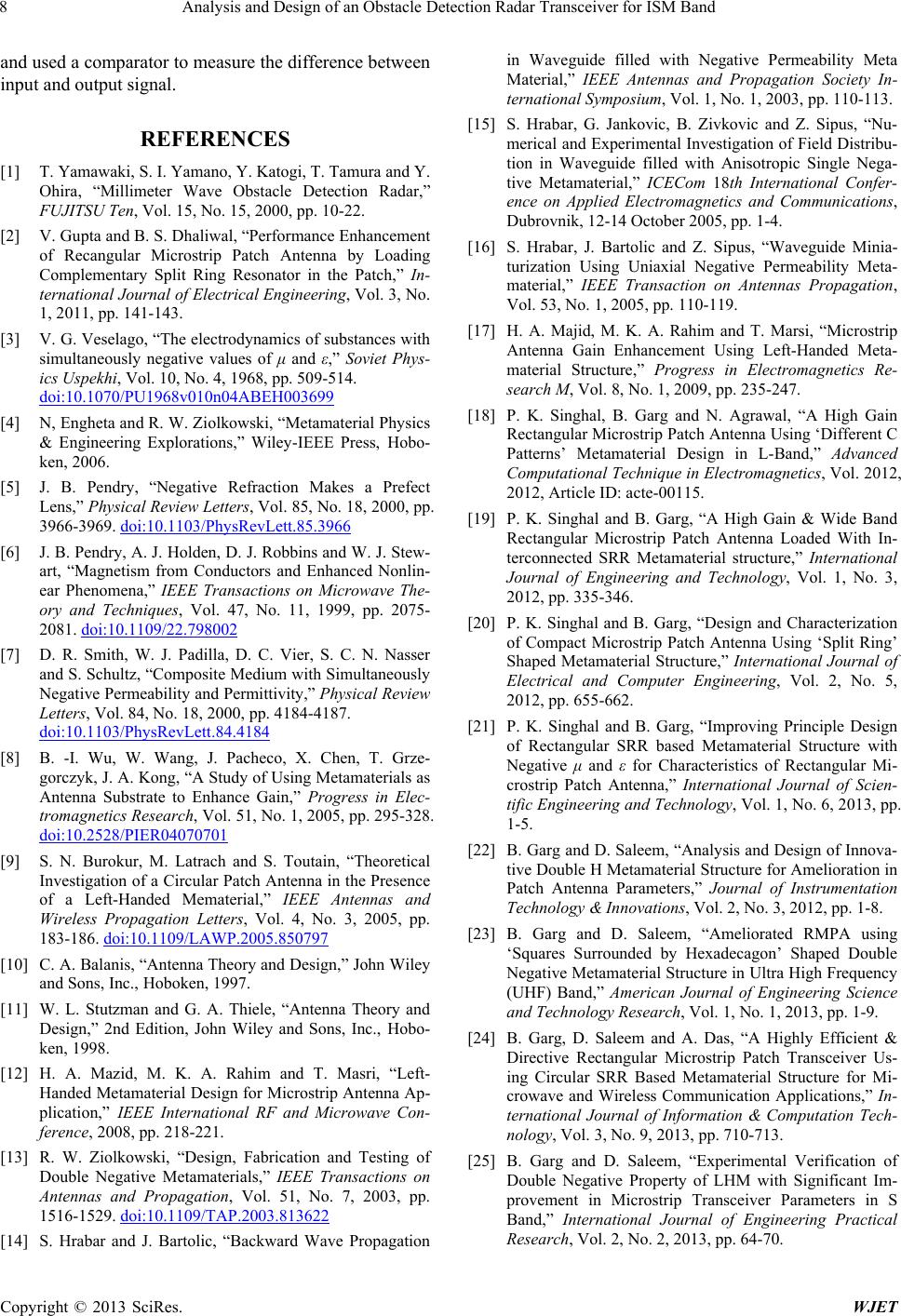
Analysis and Design of an Obstacle Detection Radar Transceiver for ISM Band
Copyright © 2013 SciRes. WJET
8
and used a comparator to measure the difference between
input and output signal.
REFERENCES
[1] T. Yamawaki, S. I. Yamano, Y. Katogi, T. Tamura and Y.
Ohira, “Millimeter Wave Obstacle Detection Radar,”
FUJITSU Ten, Vol. 15, No. 15, 2000, pp. 10-22.
[2] V. Gupta and B. S. Dhaliwal, “Performance Enhancement
of Recangular Microstrip Patch Antenna by Loading
Complementary Split Ring Resonator in the Patch,” In-
ternational Journal of Electrical Engineering, Vol. 3, No.
1, 2011, pp. 141-143.
[3] V. G. Veselago, “The electrodynamics of substances with
simultaneously negative values of μ and ε,” Soviet Phys-
ics Uspekhi, Vol. 10, No. 4, 1968, pp. 509-514.
doi:10.1070/PU1968v010n04ABEH003699
[4] N, Engheta and R. W. Ziolkowski, “Metamaterial Physics
& Engineering Explorations,” Wiley-IEEE Press, Hobo-
ken, 2006.
[5] J. B. Pendry, “Negative Refraction Makes a Prefect
Lens,” Physical Review Letters, Vol. 85, No. 18, 2000, pp.
3966-3969. doi:10.1103/PhysRevLett.85.3966
[6] J. B. Pendry, A. J. Holden, D. J. Robbins and W. J. Stew-
art, “Magnetism from Conductors and Enhanced Nonlin-
ear Phenomena,” IEEE Transactions on Microwave The-
ory and Techniques, Vol. 47, No. 11, 1999, pp. 2075-
2081. doi:10.1109/22.798002
[7] D. R. Smith, W. J. Padilla, D. C. Vier, S. C. N. Nasser
and S. Schultz, “Composite Medium with Simultaneously
Negative Permeability and Permittivity,” Physical Review
Letters, Vol. 84, No. 18, 2000, pp. 4184-4187.
doi:10.1103/PhysRevLett.84.4184
[8] B. -I. Wu, W. Wang, J. Pacheco, X. Chen, T. Grze-
gorczyk, J. A. Kong, “A Study of Using Metamaterials as
Antenna Substrate to Enhance Gain,” Progress in Elec-
tromagnetics Research, Vol. 51, No. 1, 2005, pp. 295-328.
doi:10.2528/PIER04070701
[9] S. N. Burokur, M. Latrach and S. Toutain, “Theoretical
Investigation of a Circular Patch Antenna in the Presence
of a Left-Handed Mematerial,” IEEE Antennas and
Wireless Propagation Letters, Vol. 4, No. 3, 2005, pp.
183-186. doi:10.1109/LAWP.2005.850797
[10] C. A. Balanis, “Antenna Theory and Design,” John Wiley
and Sons, Inc., Hoboken, 1997.
[11] W. L. Stutzman and G. A. Thiele, “Antenna Theory and
Design,” 2nd Edition, John Wiley and Sons, Inc., Hobo-
ken, 1998.
[12] H. A. Mazid, M. K. A. Rahim and T. Masri, “Left-
Handed Metamaterial Design for Microstrip Antenna Ap-
plication,” IEEE International RF and Microwave Con-
ference, 2008, pp. 218-221.
[13] R. W. Ziolkowski, “Design, Fabrication and Testing of
Double Negative Metamaterials,” IEEE Transactions on
Antennas and Propagation, Vol. 51, No. 7, 2003, pp.
1516-1529. doi:10.1109/TAP.2003.813622
[
14] S. Hrabar and J. Bartolic, “Backward Wave Propagation
in Waveguide filled with Negative Permeability Meta
Material,” IEEE Antennas and Propagation Society In-
ternational Symposium, Vol. 1, No. 1, 2003, pp. 110-113.
[15] S. Hrabar, G. Jankovic, B. Zivkovic and Z. Sipus, “Nu-
merical and Experimental Investigation of Field Distribu-
tion in Waveguide filled with Anisotropic Single Nega-
tive Metamaterial,” ICECom 18th International Confer-
ence on Applied Electromagnetics and Communications,
Dubrovnik, 12-14 October 2005, pp. 1-4.
[16] S. Hrabar, J. Bartolic and Z. Sipus, “Waveguide Minia-
turization Using Uniaxial Negative Permeability Meta-
material,” IEEE Transaction on Antennas Propagation,
Vol. 53, No. 1, 2005, pp. 110-119.
[17] H. A. Majid, M. K. A. Rahim and T. Marsi, “Microstrip
Antenna Gain Enhancement Using Left-Handed Meta-
material Structure,” Progress in Electromagnetics Re-
search M, Vol. 8, No. 1, 2009, pp. 235-247.
[18] P. K. Singhal, B. Garg and N. Agrawal, “A High Gain
Rectangular Microstrip Patch Antenna Using ‘Different C
Patterns’ Metamaterial Design in L-Band,” Advanced
Computational Technique in Electromagnetics, Vol. 2012,
2012, Article ID: acte-00115.
[19] P. K. Singhal and B. Garg, “A High Gain & Wide Band
Rectangular Microstrip Patch Antenna Loaded With In-
terconnected SRR Metamaterial structure,” International
Journal of Engineering and Technology, Vol. 1, No. 3,
2012, pp. 335-346.
[20] P. K. Singhal and B. Garg, “Design and Characterization
of Compact Microstrip Patch Antenna Using ‘Split Ring’
Shaped Metamaterial Structure,” International Journal of
Electrical and Computer Engineering, Vol. 2, No. 5,
2012, pp. 655-662.
[21] P. K. Singhal and B. Garg, “Improving Principle Design
of Rectangular SRR based Metamaterial Structure with
Negative μ and ε for Characteristics of Rectangular Mi-
crostrip Patch Antenna,” International Journal of Scien-
tific Engineering and Technology, Vol. 1, No. 6, 2013, pp.
1-5.
[22] B. Garg and D. Saleem, “Analysis and Design of Innova-
tive Double H Metamaterial Structure for Amelioration in
Patch Antenna Parameters,” Journal of Instrumentation
Technology & Innovations, Vol. 2, No. 3, 2012, pp. 1-8.
[23] B. Garg and D. Saleem, “Ameliorated RMPA using
‘Squares Surrounded by Hexadecagon’ Shaped Double
Negative Metamaterial Structure in Ultra High Frequency
(UHF) Band,” American Journal of Engineering Science
and Technology Research, Vol. 1, No. 1, 2013, pp. 1-9.
[24] B. Garg, D. Saleem and A. Das, “A Highly Efficient &
Directive Rectangular Microstrip Patch Transceiver Us-
ing Circular SRR Based Metamaterial Structure for Mi-
crowave and Wireless Communication Applications,” In-
ternational Journal of Information & Computation Tech-
nology, Vol. 3, No. 9, 2013, pp. 710-713.
[25] B. Garg and D. Saleem, “Experimental Verification of
Double Negative Property of LHM with Significant Im-
provement in Microstrip Transceiver Parameters in S
Band,” International Journal of Engineering Practical
Research, Vol. 2, No. 2, 2013, pp. 64-70.