 Open Journal of Cell Biology, 2012, 2, 1-9 doi:10.4236/ojcb.2012.21001 Published Online March 2012 (http://www.SciRP.org/journal/ojcb) 1 Immunoresponse to Allogeneic Synovial or Xenogenic Mesenchymal Stromal Cells in a Co-Culture Model Seth S. Jump1,2, David S. Smith1, David C. Flanigan3, Alicia L. Bertone1,2 1Comparative Orthopaedic Research Laboratories, The Ohio State University, Columbus, USA 2Department of Veterinary Biosciences, College of Veterinary Medicine, The Ohio State University, Columbus, USA 3Department of Orthopaedics, College of Medicine, The Ohio State University, Columbus, USA Email: bertone.1@osu.edu Received February 2, 2012; revised March 7, 2012; accepted March 16, 2012 ABSTRACT The purpose of our investigation s was to measure, in a co-cu lture cond ition, th e immunoresponse to allog eneic or xeno- genic cells, selected as potential sources for cell therapy of arthritis. We challenged human spleen-derived cells (hSpl) by three different mechanisms: 1) exposure to donor allogeneic or xenogeneic cellular antigens; 2) exposure to donor cells transduced with adenoviral antigens (Ad) and 3) lipopolysaccharide (LPS), a known inflammatory immunostimu- lant. The immunoresponse to allogeneic human synovial-derived mesenchymal stromal cells alone or transduced with adenoviral green fluorescent protein (hSD-MSC or hSD-MSC/GFP) or the immunoresponse to xenogeneic equine mesenchymal stromal cells (eqMSC) or equine dermal fibroblasts (eqDFb), characterized by the proportion of CD3+, CD4+, and CD8+ human splenocytes (hSpl), was measured on Day 0 and Day 6 of co-culture by flow cytometry. In culture with hSD-MSC, hSD-MSC/GFP, eqDFb, or eqMSC, the proportion of CD3+ and CD8+ hSpl increased with time in culture but not with expo sure to cell allo- or xeno-antigens. Both hSD-MSC and hSD-MSC/GFP increased in number during culture and were not affected in viab ility or proliferation b y co-culture with allogeneic hSpl. In this in vitro, pri- mary exposure stud y, hSpl demonstrated a natural selectio n and adap tation to a short-term cell culture env ironment, and that neither allogeneic nor xenogeneic cell antigens incited a greater cellular immunoactivation than co-cultured hSpl alone. Keywords: Arthritis; Stem Cells; Allogeneic; Xenogeneic; Immunoresponse 1. Introduction Cutting-edge advancements in regenerative medicine may harness the potential of an engineered cell source as a therapeutic vecto r for the repair and restoration of multi- ple human tissues including articular cartilage damaged by injury or degenerated through either osteoarthritis or rheumatoid arthritis. Allogeneic cells, from a different organism of the same species or xenogenic cells, from a different species, could provide a nearly limitless supply of therapeutic cells for use in tissue repair. Allogeneic or xenogeneic cell sources, engineered to serve as a clinical tool, could dramatically and irrevocably enhance current medical practices that promote healing. Allogeneic mesenchymal stem cells in Phase III clini- cal trials, have been used as a treatment for inflammatory conditions including acute Bone Versus Graft Disease and Crohn’s disease [1]. In orthopaedic medicine, au tolo- gus cell-based strategies for tissue repair and restoration, including autologous chondroctye implantation and os- teochondral grafts, are currently used in clinical practice with some promising results [2-6]. A potentially promis- ing alternative or ad junct strategy to current regenerative techniques could use allogeneic cells or xenogenic cells. To this end, a cell-based allogeneic therapy has been provided for 1st generation, commercially-available car- tilage neograft (DeNovo® ET Live Cho ndral Engineered Tissue Graft, Zimmer Holdings, Inc). This product, ap- proved last year, relies on allogeneic, juvenile chondro- cytes and a proprietary cell-scaffold system to promote healing. Potentially, allogeneic or xenogeneic cells could serve as effective therapeutic vectors in vivo to integrate into the native biological environment of tissue. The im- munoresponse of allogeneic and xenogeneic cells needs to be further investigated, and further observations of the cellular mechanisms and interactions will help to eluci- date and describe the immunoresponse for future clinical trials. New cell sources could improve the repair and restora- tion of articular cartilage, such as in injured cartilage or deteriorating cartilage as in osteoarthritis (OA), the most common form of arthritis. It is believed that there are immune processes that could be responsible for the de- Copyright © 2012 SciRes. OJCB
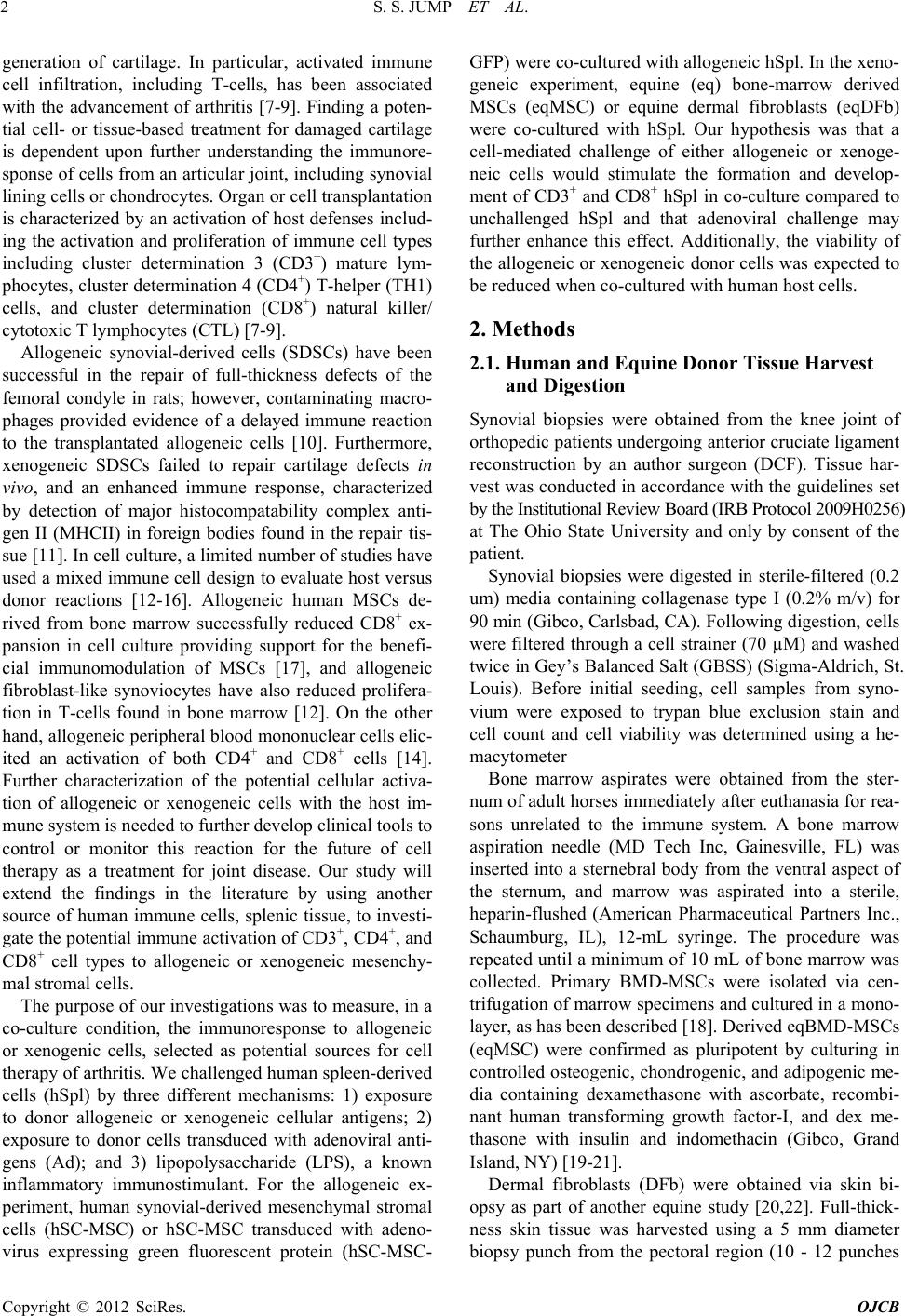 S. S. JUMP ET AL. 2 generation of cartilage. In particular, activated immune cell infiltration, including T-cells, has been associated with the advancement of arthritis [7-9]. Finding a poten- tial cell- or tissue-based treatment for damaged cartilage is dependent upon further understanding the immunore- sponse of cells from an articular joint, in cluding synovial lining cells or chondrocytes. Organ or cell transp lantation is characterized by an activation of host defenses includ- ing the activation and proliferation of immune cell types including cluster determination 3 (CD3+) mature lym- phocytes, cluster determination 4 (CD4+) T-helper (TH1) cells, and cluster determination (CD8+) natural killer/ cytotoxic T lymphocytes (CTL) [7-9]. Allogeneic synovial-derived cells (SDSCs) have been successful in the repair of full-thickness defects of the femoral condyle in rats; however, contaminating macro- phages provided evidence of a delayed immune reaction to the transplantated allogeneic cells [10]. Furthermore, xenogeneic SDSCs failed to repair cartilage defects in vivo, and an enhanced immune response, characterized by detection of major histocompatability complex anti- gen II (MHCII) in foreign bodies found in the repair tis- sue [11]. In cell culture, a limited number of studies have used a mixed immune cell design to evaluate host versus donor reactions [12-16]. Allogeneic human MSCs de- rived from bone marrow successfully reduced CD8+ ex- pansion in cell culture providing support for the benefi- cial immunomodulation of MSCs [17], and allogeneic fibroblast-like synoviocytes have also reduced prolifera- tion in T-cells found in bone marrow [12]. On the other hand, allogeneic peripheral blood mononuclear cells elic- ited an activation of both CD4+ and CD8+ cells [14]. Further characterization of the potential cellular activa- tion of allogeneic or xenogeneic cells with the host im- mune system is needed to further develop clinical tools to control or monitor this reaction for the future of cell therapy as a treatment for joint disease. Our study will extend the findings in the literature by using another source of human immune cells, splenic tissue, to investi- gate the potential immune activation of CD3+, CD4+, and CD8+ cell types to allogeneic or xenogeneic mesenchy- mal stromal cells. The purpose of our investigatio ns was to measure, in a co-culture condition, the immunoresponse to allogeneic or xenogenic cells, selected as potential sources for cell therapy of arthritis. We challeng ed hu man spleen-d erived cells (hSpl) by three different mechanisms: 1) exposure to donor allogeneic or xenogeneic cellular antigens; 2) exposure to donor cells transduced with adenoviral anti- gens (Ad); and 3) lipopolysaccharide (LPS), a known inflammatory immunostimulant. For the allogeneic ex- periment, human synovial-derived mesenchymal stromal cells (hSC-MSC) or hSC-MSC transduced with adeno- virus expressing green fluorescent protein (hSC-MSC- GFP) were co-cultured with allogen eic hSpl. In the xeno- geneic experiment, equine (eq) bone-marrow derived MSCs (eqMSC) or equine dermal fibroblasts (eqDFb) were co-cultured with hSpl. Our hypothesis was that a cell-mediated challenge of either allogeneic or xenoge- neic cells would stimulate the formation and develop- ment of CD3+ and CD8+ hSpl in co-culture compared to unchallenged hSpl and that adenoviral challenge may further enhance this effect. Additionally, the viability of the allogeneic or xenogeneic donor cells was expected to be reduced when co-cultured with human host cells. 2. Methods 2.1. Human and Equine Donor Tissue Harvest and Digestion Synovial biopsies were obtained from the knee joint of orthopedic pa tients undergoing anterio r cruciate ligament reconstruction by an author surgeon (DCF). Tissue har- vest was conducted in accordance with the guidelines set by the Institutional Review Board (IRB Protocol 2009H0256) at The Ohio State University and only by consent of the patient. Synovial biopsies were digested in sterile-filtered (0.2 um) media containing collagenase type I (0.2% m/v) for 90 min (Gibco, Carlsbad, CA). Following digestion, cells were filtered through a cell strainer (70 µM) and washed twice in Gey’s Balanced Salt (GBSS) (Sigma-Aldrich, St. Louis). Before initial seeding, cell samples from syno- vium were exposed to trypan blue exclusion stain and cell count and cell viability was determined using a he- macytometer Bone marrow aspirates were obtained from the ster- num of adult horses immediately after euthanasia for rea- sons unrelated to the immune system. A bone marrow aspiration needle (MD Tech Inc, Gainesville, FL) was inserted into a sternebral body from the ventral aspect of the sternum, and marrow was aspirated into a sterile, heparin-flushed (American Pharmaceutical Partners Inc., Schaumburg, IL), 12-mL syringe. The procedure was repeated until a minimum of 1 0 mL of bone marrow was collected. Primary BMD-MSCs were isolated via cen- trifugation of marrow specimens and cultured in a mono- layer, as has been described [18]. Derived eqBMD-MSCs (eqMSC) were confirmed as pluripotent by culturing in controlled osteogenic, chondrogenic, and adipogenic me- dia containing dexamethasone with ascorbate, recombi- nant human transforming growth factor-I, and dex me- thasone with insulin and indomethacin (Gibco, Grand Island, NY) [19-21]. Dermal fibroblasts (DFb) were obtained via skin bi- opsy as part of another equine study [20,22]. Full-thick- ness skin tissue was harvested using a 5 mm diameter biopsy punch from the pectoral region (10 - 12 punches Copyright © 2012 SciRes. OJCB
 S. S. JUMP ET AL. 3 per horse) from each of six adult horses. The dermal layer was dissected from the epidermis under a micro- scope, and DFbs were isolated by type-1 collagenase digestion (GIBCO, Grand Island, NY) and cultured in DMEM supplemented with L-glutamine (300 mg/mL), penicillin (30 mg/mL), streptomycin (30 mg/mL), and 10% fetal bovine serum at 37˚C in a 5% CO2 atmosphere. Synovial-derived mesenchymal stromal cells were pas- saged a minimum of 4 times, but no more than 7 times. Previous studies in the literature have demonstrated that hSD-MSCs cells maintain a consistent phenotype be- tween passages 3 and 8 [23,24]. Equine BMD- MSC and Dfb were low passage ( <3 passages ). 2.2. Tissue Harvest of Host Mixed Immune Cells Human spleens were selected as the host mixed cell population for co-culture experiments. Approval for re- ceiving portions of human spleens was granted by Life- line of Ohio, an organ donor center in Columbus, Ohio. Splenocytes were h arvested and p repared with h igh yield and successfully grown in cell culture using methods adapted from murine splenocyte culture [25,26]. Briefly, tissue was trimmed into small pieces and thoroughly minced using a syringe plunger inside of a nylon cell strainer (70 µm) in a 35 mm cell culture plate. Cells were digested using Ack lysing buffer (Gibco, Carlsbad, CA), and the samples were subjected to consecutive washes in ice cold PBS. Isolated splenocytes were immediately allocated to cultures as described below in experimental design. 2.3. Adenoviral Transduction of Donor Allogeneic Synovial-Derived Mesenchymal Stromal Cells Recombinant, E1-deficient, serotype-5 adenovirus vec- tors containing the open reading frame segment of hu- man GFP (AdGFP) under the control of the cytomega- lovirus promoter were generated. Successful transduction of AdGFP was verified in cell culture. Viral titer [infec- tion units per mL, (IFU/mL)] was determined (Adeno-X Rapid TiterKit; Clonetech, Mountain View, CA, USA) and SD-MSCs were transduced at 100 multiplicities of infection (MOI) or 1 × 102 infectious units per cell [19- 21]. 2.4. Cell Co-Culture Conditions and Experimental Design For the overall experimental design, three sources of do- nor cells, hSD-MSC (allogeneic; Experiment 1) and eqMSC and eqDFb (xenogeneic; Experiment 2), were seeded at 10,000 cells/cm2 onto 24-well cell culture plates at Day –2 in 10% FBS containing 1% penicillin streptomycin in DMEM (Invitrogen). Human SD-MSC cultures were transduced with Ad-GFP on Day –1. For Experiments 1 and 2, isolated hSpl were obtained from 3 different hu- man spleens for each experiment (6 spleens) and cultured in triplicate alone or added to donor cultures at a 50:1 ratio or 500,000 hSpl/cm2 on Day 0. For Experiment 1, hSpl were added to one of three co-culture immune chal- lenges at Day 0; allogeneic hSD-MSC, allogeneic hSD- MSC-GFP, or lipopolysaccharide stimulation (LPS 10 µg/mL, Sigma-Aldrich, St. Louis, MO). For Experiment 2, hSpl were assigned to one of three co-culture immune challenges at Day 0; xenogeneic eqMSC, xenogeneic eqDFb, or lipopolysacch aride stimulation (LPS 10µg/mL, Sigma-Aldrich, St. Louis, MO). On Day –1, hSD-MSC wells were assigned to receive adenoviral vector trans- duction. Cells were transduced with AdGFP at 100 MOI for 2 h in Gey’s Balanced Salt Solution. Effective trans- duction methods with AdGFP have been validated in previously published work [19]. On Day 0, prior to the addition of the allogeneic hSpl cells, GFP expression was confirmed by fluorescent microscopy and recorded. In addition to co-cultures, hSpl were cultured alone. At day 0, hSpl were removed for cellular viability staining and flow cytometry analysis approximately two hours after co-cultures were established to serve as a baseline im- mune status. At Days 0 and 6, hSpl were harvested for cell surface marker content as measured by flow cytome- try. 2.5. Flow Cytometry, Cell Numbers, and Cell Viability Flow cytometry (C-Flow Plus, Accuri, Ann Arbor, MI) was performed on hSpl subjected to extracellular staining to determine the proportion of cells pos itively-stained for binding of CD3, CD4, or CD8 antibodies. Human mono- clonal antibodies for each immune cell subtype (FITC- conjugated-anti-CD3, APC-conjugated anti-CD4, and PE- conjugated anti-CD8, R&D Systems, Minneapolis, MN) were used to assess the potential formation of immune cell types. At Days 0 and 6, we investigated formation of CD3+, CD4+, and CD8+ cells; an unstained control and an antibody control for each respective antibody were used on each of flow cytometry analysis. Cell number and viability for both hSD-MSC and hSD-MSC/GFP were determined on Day 6. Cells were trypsinized, washed, and subjected to a trypan blue ex- clusion stain. Cells were counted in a hemacyometer; the number of live cells was recorded. 2.6. Statistical Analysis For co-culture experiments, cells isolated from one hu- man joint (hSD-MSC or hSD-MSC/GFP or one animal (eqMSC or eqDFb) and an individual spleen (hSpl) were Copyright © 2012 SciRes. OJCB
 S. S. JUMP ET AL. 4 considered an n of 1. Data for quantitative outcomes of hSpl CD3+, CD4+, CD8+ number and proportion, as well as donor cell number and viability were presented as means ± standard error of the mean (SEM). Two factor analysis of variance (ANOVA) for Day (0 and 6) and hSpl condition (alone, hSD-MSC, hSD-MSC-GFP; or alone, eqMSC, eqDFb, LPS) was performed for hSpl number, donor cell number, and hSpl or donor cell vi- ability. All analyses were performed using a comer- cially available statistical software package (Statistical Analysis Software, SAS 9.1). Statistical significance was accepted at p < 0.05 3. Results Human spleens were obtained on ice, and splenoctyes were harvested with initial high hSpl number and viabil- ity (>95%) (Figure 1(A)) and co-cultured with donor cells at Day 0 (Figure 1(B)). The proportion of gated viable hSpl on the Day 0 of cultur e measurement by flow cytometry was in the range of 65% - 74% (Figures 2(a) and 2(b)). The proportion of CD+ hSpl (CD3+, CD8+, plus CD4+) was <15% of total gated cells on Day 0 cul- ture measurement by flow cytometry (Figures 3 and 4). Culture of hSpl alone resu lted in a significan t decrease in hSpl numbers and viability to an average of approxi- mately 31% by Day 6 as measured by flow cytometry (Figures 2(a) and 2( b) ). Co-culture of hSpl with alloge- neic or xenogeneic donor cells did not further decrease hSpl numbers (Figures 2(a) and 2(b)). Both allogeneic and xenogeneic donor cells proliferated in cell co -culture with hSpl (Figure 5) and were of normal morphology with high efficiency of GFP expression demonstrated in hSD-MSC-GFP cells for the entire 6 days. (Figure 1(B), insert) Synovial-derived mesenchymal stromal cells were successfully transduced (hSD-MSC-GFP) 24h after trans- duction with AdGFP at 100 multiplicities of infection (100 MOI). (Day 0). In the allogeneic co-culture Experiment 1, the propor- tion of CD3+ and CD8+ hSpl was significantly greater on Day 6 (4-fo ld and 5-fold, respectively) r egardless of cul- ture condition as compared to Day 0. (Figures 3(a) and 3 (b)) On Day 6, hSpl cultured in LPS had a greater proportion o f CD3+ hSpl than hSpl cultured alone. There was no change in the proportion of CD4+ hSpl on Day 6 versus 0 (Figure 3(c)). In the xenogeneic co-culture Experiment 2, the propor- tion of CD3+ and CD8+ hSpl was significantly greater on Day 6 (3-fo ld and 6-fold, respectively) r egardless of cul- ture condition as compared to Day 6 as in the allogeneic co-culture experiment and compared to Day 0. (Figures 4(a) and 4(b)) On Day 6, hSpl co-cultured with eqDFb had a lesser proportion of CD8+ hSpl than hSpl cultured alone. There was no change in the proportion of CD4+ Figure 1. (A) Photomicrograph (100× magnification) of a hemacytometer containing a cell dilution of human spleno- cytes stained with trypan blue stain after being digested from a human spleen. Viable hSpl are yellow and circular, and non-viable human splenocytes (hSpl) are blue and py k- notic. Each spleen sample that was digested had greater than 95% living cells prior to the start of the co-culture experiments (Day 0); (B) Photomicrograph (100× magnfi- cation) of a co-culture containing hSpl (small circ ular cells) and human synovial-derived mesenchymal stromal cells (hSD-MSC) 100x magnification. Human SD-MSC trans- duced with AdGFP at 100 infectious Ad particles per cell, had >95% expression of GFP under fluore scent microscopy at 24 hours (insert; 200× magnification). cells between days (Figure 4(c)). 4. Discussion This study provided evid ence that, in a short-term culture environment, hSpl undergo a natural selection and adap- tation to cell culture conditions. In the allogeneic or xenogenic co-culture conditions, no particular evaluated cell source (allogeneic synovial or xenogeneic bone- marrow, or skin) or donor source (human allogeneic or equine xenogeneic) promoted survival, inhibited death, or promoted death of the lymphocyte cell fraction of hSpl. In addition, these data show ed that neither allogene ic nor Copyright © 2012 SciRes. OJCB
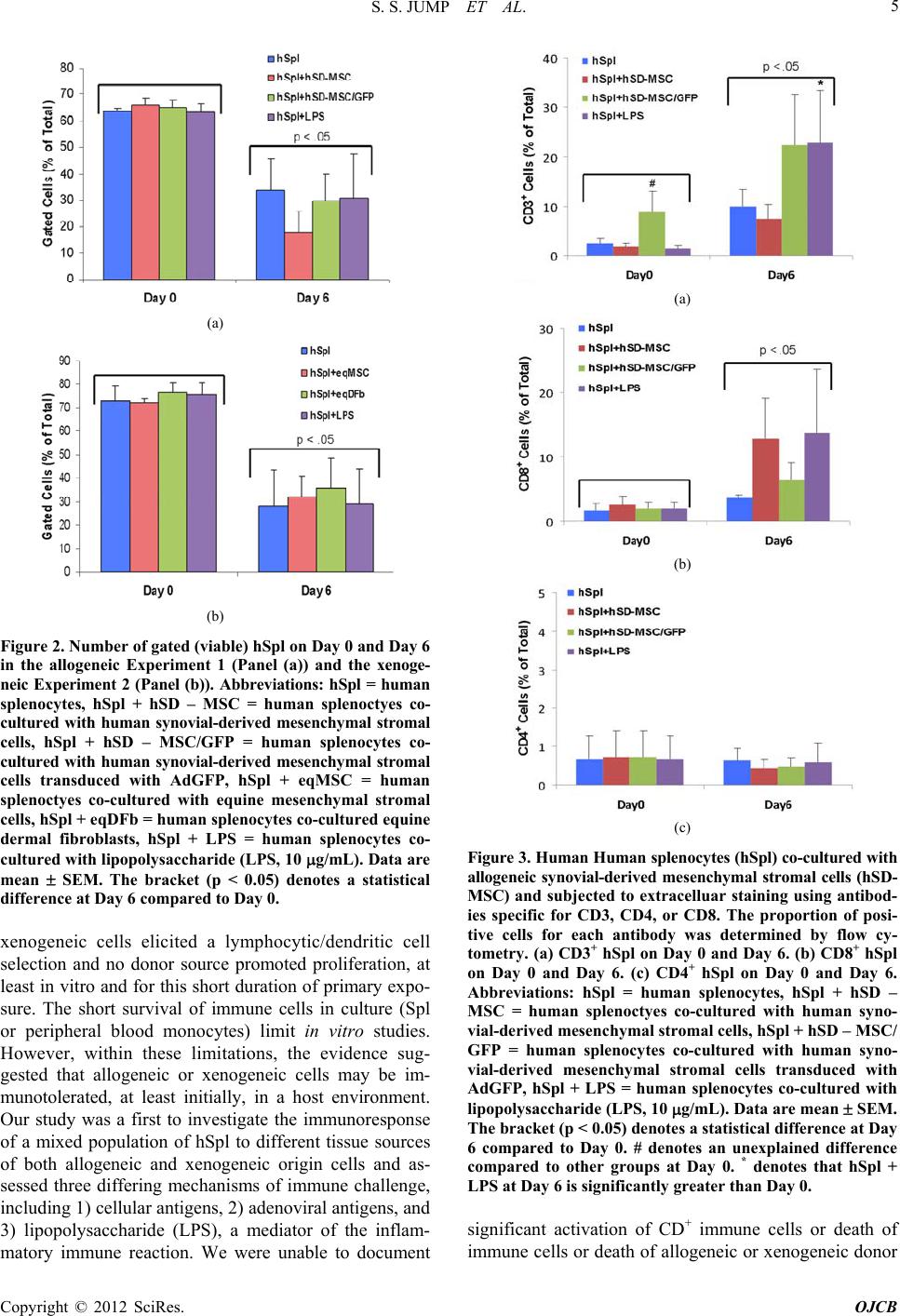 S. S. JUMP ET AL. 5 (a) (b) Figure 2. Number of gated (viable) hSpl on Day 0 and Day 6 in the allogeneic Experiment 1 (Panel (a)) and the xenoge- neic Experiment 2 (Panel (b)). Abbreviations: hSpl = human splenocytes, hSpl + hSD – MSC = human splenoctyes co- cultured with human synovial-derived mesenchymal stromal cells, hSpl + hSD – MSC/GFP = human splenocytes co- cultured with human synovial-derived mesenchymal stromal cells transduced with AdGFP, hSpl + eqMSC = human splenoctyes co-cultured with equine mesenchymal stromal cells, hSpl + eqDFb = human splenoc yte s co-culture d equine dermal fibroblasts, hSpl + LPS = human splenocytes co- cultured with lipopolysaccharide (LPS, 10 g/mL). Data are mean SEM. The bracket (p < 0.05) denotes a statistical difference at Day 6 compared to Day 0. xenogeneic cells elicited a lymphocytic/dendritic cell selection and no donor source promoted proliferation, at least in vitro and for this short duration of primary expo- sure. The short survival of immune cells in culture (Spl or peripheral blood monocytes) limit in vitro studies. However, within these limitations, the evidence sug- gested that allogeneic or xenogeneic cells may be im- munotolerated, at least initially, in a host environment. Our study was a first to investigate the immunoresponse of a mixed population of hSpl to different tissue sources of both allogeneic and xenogeneic origin cells and as- sessed three differing mechanisms of immune challenge, including 1) cellular antig ens, 2) aden oviral antigens, and 3) lipopolysaccharide (LPS), a mediator of the inflam- matory immune reaction. We were unable to document (a) (b) (c) Figure 3. Human Human splenocytes (hSpl) co-cultured with allogeneic synovial-derived mesenchymal stromal cells (hSD- MSC) and subjected to extracelluar staining using antibod- ies specific for CD3, CD4, or CD8. The proportion of posi- tive cells for each antibody was determined by flow cy- tometry. (a) CD3+ hSpl on Day 0 and Day 6. (b) CD8+ hSpl on Day 0 and Day 6. (c) CD4+ hSpl on Day 0 and Day 6. Abbreviations: hSpl = human splenocytes, hSpl + hSD – MSC = human splenoctyes co-cultured with human syno- vial-derived mesenchy mal stromal cells, hSpl + hSD – M SC/ GFP = human splenocytes co-cultured with human syno- vial-derived mesenchymal stromal cells transduced with AdGFP, hSpl + LPS = human splenocytes co-cultured with lipopolysaccharide (LPS, 10 g/mL). Data are mean SEM. The bracket (p < 0.05) denotes a statistical difference at Day 6 compared to Day 0. # denotes an unexplained difference compared to other groups at Day 0. * denotes that hSpl + LPS at Day 6 is significantly greater than Day 0. significant activation of CD+ immune cells or death of immune cells or death of allogeneic or xenogeneic donor Copyright © 2012 SciRes. OJCB
 S. S. JUMP ET AL. 6 (a) (b) (c) Figure 4. Human splenocytes (hSpl) co-cultured with xeno- geneic equine bone-marrow derived mesenchymal stromal cells (eqMSC) or dermal fibroblasts (eqDFb) and subjected to extracelluar staining using antibodies specific for CD3, CD4, or CD8. The proportion of positive cells for each an- tibody was determined by flow cytometry. (a) CD3+ hSpl on Day 0 and Day 6. (b) CD8+ hSpl on Day 0 and Day 6. (c) CD4+ hSpl on Day 0 and Day 6. Abbreviations: hSpl = hu- man splenocytes, hSpl + eqMSC = human splenoctyes co- cultured with equine mesenchymal stromal cells, hSpl + eqDFb = human splenocytes co-cultured equine dermal fi- broblasts, hSpl + LPS = human splenocytes co-cultured with lipopolysaccharide (LPS, 10 g/mL). Data are mean SEM. The bracket (p < 0.05) denotes a statistical difference at Day 6 compared to Day 0. *denotes that hSpl + EqDFb was significantly lower than other groups at Day 6. cells. Dermal fibroblasts have not been stated to be im- munoprivileged as has been claimed for the synovial fibroblast or bone-marrow derived mesenchymal pro- Figure 5. Cell number of human synovial-derived mesen- chymal stromal cells (hSD-MSC) and human synovial-de- rived mesenchymal stromal cells transduced with adenovi- rus containing green fluorescent protein (hSD – MSC/GFP) on Day 0 and Day 6 as determined by hemacytometer live cell count following a trypan blue exclusion staining Data are mean SEM. The bracket (p < 0.05) denotes a statisti- cal difference at Day 6 compared to Day 0. genitor and stromal cells, but our data suggested that xenogeneic dermal fibroblasts may be immunosuppres- sive to CD8+ cells and would warrant further investiga- tion. Importantly, further experiments in vivo would be ne- cessary to more critically assess immunotolerance, to include longer exposure to antigens, and evaluate a sec- ond exposure to elucidate the cellular contributions and anamnestic response to isolated allogeneic or xenogeneic cells. To our knowledge, our splenocyte co-culture sys- tem is novel and contributed initial information on toler- ance of these mixed immune cells to donor cells on short term exposure and supports previous publications claim- ing allogeneic juvenile chondroctyes exhibit immunotol- erance [27]. Previous work has also provided evidence that synovial cells have immune privilege an d will not be rejected by a host organism [28,29]. Some studies sup- ported the tolerance of allo- or xenotransplants for tissue healing [30,31]. An additional study showed that xeno- geneic chondrocytes can successfully repair full-thick- ness cartilage defects [32]. However, in a recent 2010 study, xenotransplantation of porcine chondrocytes into rabbits was unsuccessful for long term cartilage healing [11]. Our study provided a unique contribution to the rela- tively limited body of work in the literature using this type of co-culture model. Our data is in agreement with previous literature suggesting that mesenchymal stromal cells maybe immunotolerated or immunoprivileged [15, 17,33]. Findings in our human allogeneic experiment demonstrated that no detectable immune activation was prompted; however, an immunosuppression was not ob- served as in other studies. In cell culture, MSCs have also been able to suppress T cell proliferation [13], sug- Copyright © 2012 SciRes. OJCB
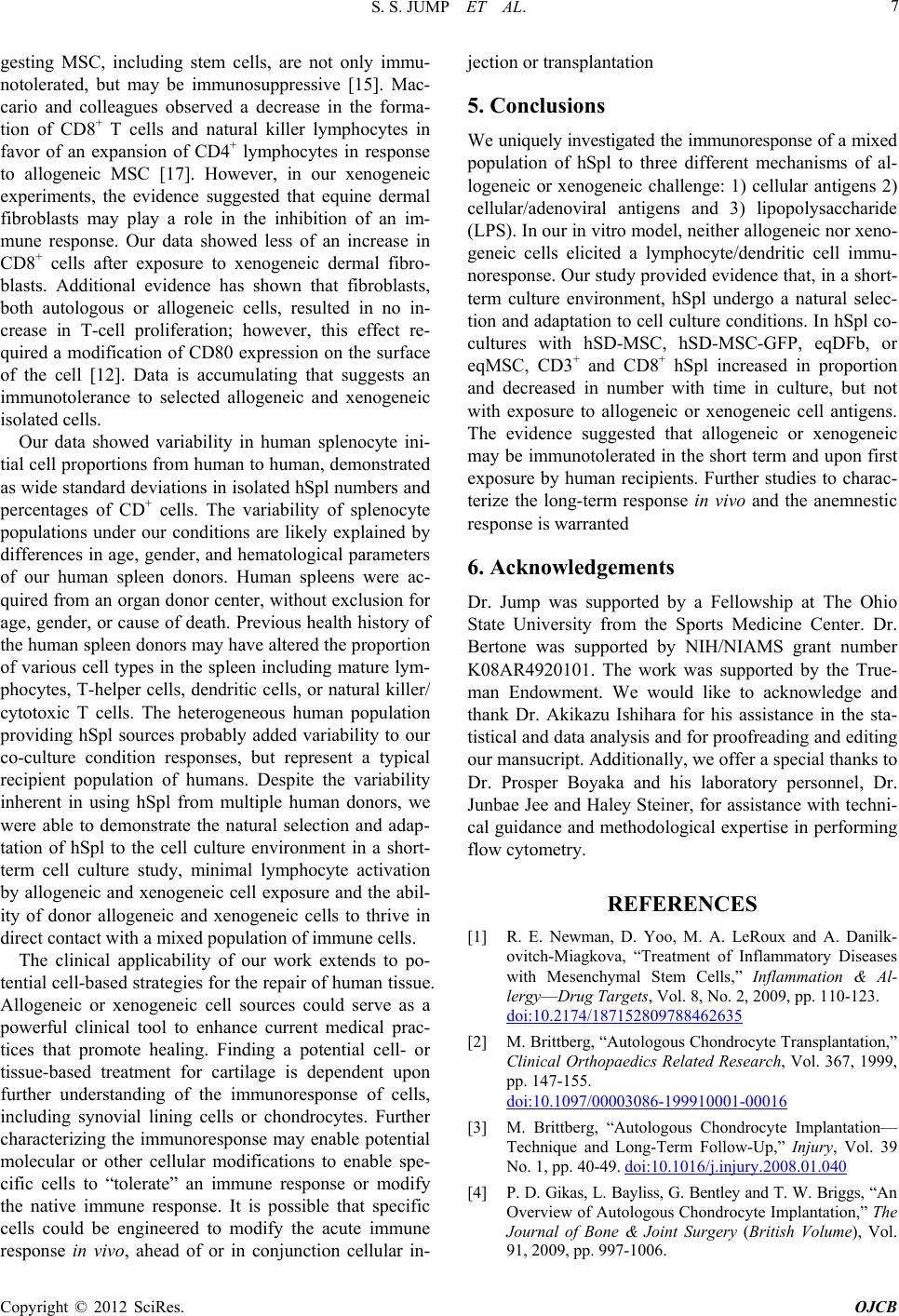 S. S. JUMP ET AL. 7 gesting MSC, including stem cells, are not only immu- notolerated, but may be immunosuppressive [15]. Mac- cario and colleagues observed a decrease in the forma- tion of CD8+ T cells and natural killer lymphocytes in favor of an expansion of CD4+ lymphocytes in response to allogeneic MSC [17]. However, in our xenogeneic experiments, the evidence suggested that equine dermal fibroblasts may play a role in the inhibition of an im- mune response. Our data showed less of an increase in CD8+ cells after exposure to xenogeneic dermal fibro- blasts. Additional evidence has shown that fibroblasts, both autologous or allogeneic cells, resulted in no in- crease in T-cell proliferation; however, this effect re- quired a modification of CD80 expression on the surface of the cell [12]. Data is accumulating that suggests an immunotolerance to selected allogeneic and xenogeneic isolated cells. Our data showed variability in human splenocyte ini- tial cell proportions from human to human, demonstrated as wide standard deviations in isolated hSpl numbers and percentages of CD+ cells. The variability of splenocyte populations under our conditions are likely explained by differences in age, gender, and hematological parameters of our human spleen donors. Human spleens were ac- quired from an organ donor center, without exclusion for age, gender, or cause of death. Previous health history of the human spleen donor s may have altered the propor tion of various cell types in the spleen including mature lym- phocytes, T-helper cells, dendritic cells, or natural killer/ cytotoxic T cells. The heterogeneous human population providing hSpl sources probably added variability to our co-culture condition responses, but represent a typical recipient population of humans. Despite the variability inherent in using hSpl from multiple human donors, we were able to demonstrate the natural selection and adap- tation of hSpl to the cell culture environment in a short- term cell culture study, minimal lymphocyte activation by allogeneic and xenogeneic cell exposure and the abil- ity of donor allogeneic and xenogeneic cells to thrive in direct contact with a mixed population of immune cells. The clinical applicability of our work extends to po- tential cell-based strategies for the repair of human tissue. Allogeneic or xenogeneic cell sources could serve as a powerful clinical tool to enhance current medical prac- tices that promote healing. Finding a potential cell- or tissue-based treatment for cartilage is dependent upon further understanding of the immunoresponse of cells, including synovial lining cells or chondrocytes. Further characterizing the immunoresponse may enable potential molecular or other cellular modifications to enable spe- cific cells to “tolerate” an immune response or modify the native immune response. It is possible that specific cells could be engineered to modify the acute immune response in vivo, ahead of or in conjunction cellular in- jection or transplantation 5. Conclusions We uniquely investigated the immunoresponse of a mixed population of hSpl to three different mechanisms of al- logeneic or xenogeneic challenge: 1) cellular antigens 2) cellular/adenoviral antigens and 3) lipopolysaccharide (LPS). In our in vitro model, neither allogeneic nor xeno- geneic cells elicited a lymphocyte/dendritic cell immu- noresponse. Our study provided evidence that, in a short- term culture environment, hSpl undergo a natural selec- tion and adap tation to cell culture conditions. In hSpl co- cultures with hSD-MSC, hSD-MSC-GFP, eqDFb, or eqMSC, CD3+ and CD8+ hSpl increased in proportion and decreased in number with time in culture, but not with exposure to allogeneic or xenogeneic cell antigens. The evidence suggested that allogeneic or xenogeneic may be immunotolerated in the short term and upon first exposure by human recipients. Further studies to charac- terize the long-term response in vivo and the anemnestic response is warranted 6. Acknowledgements Dr. Jump was supported by a Fellowship at The Ohio State University from the Sports Medicine Center. Dr. Bertone was supported by NIH/NIAMS grant number K08AR4920101. The work was supported by the True- man Endowment. We would like to acknowledge and thank Dr. Akikazu Ishihara for his assistance in the sta- tistical and data analysis and for proofreading and editing our mansucript. Additionally, we offer a special thanks to Dr. Prosper Boyaka and his laboratory personnel, Dr. Junbae Jee and Haley Steiner, for assistance with techni- cal guidance and methodological expertise in performing flow cytometry. REFERENCES [1] R. E. Newman, D. Yoo, M. A. LeRoux and A. Danilk- ovitch-Miagkova, “Treatment of Inflammatory Diseases with Mesenchymal Stem Cells,” Inflammation & Al- lergy—Drug Targets, Vol. 8, No. 2, 2009, pp. 110-123. doi:10.2174/187152809788462635 [2] M. Brittberg, “Autologous Chondrocyte Transplantation,” Clinical Orthopaedics Related Research, Vol. 367, 1999, pp. 147-155. doi:10.1097/00003086-199910001-00016 [3] M. Brittberg, “Autologous Chondrocyte Implantation— Technique and Long-Term Follow-Up,” Injury, Vol. 39 No. 1, pp. 40-49. doi:10.1016/j.injury.2008.01.040 [4] P. D. Gikas, L. Bayliss, G. Bentley and T. W. Briggs, “An Overview of Autologous Chondrocyte Implantation,” The Journal of Bone & Joint Surgery (British Volume), Vol. 91, 2009, pp. 997-1006. Copyright © 2012 SciRes. OJCB
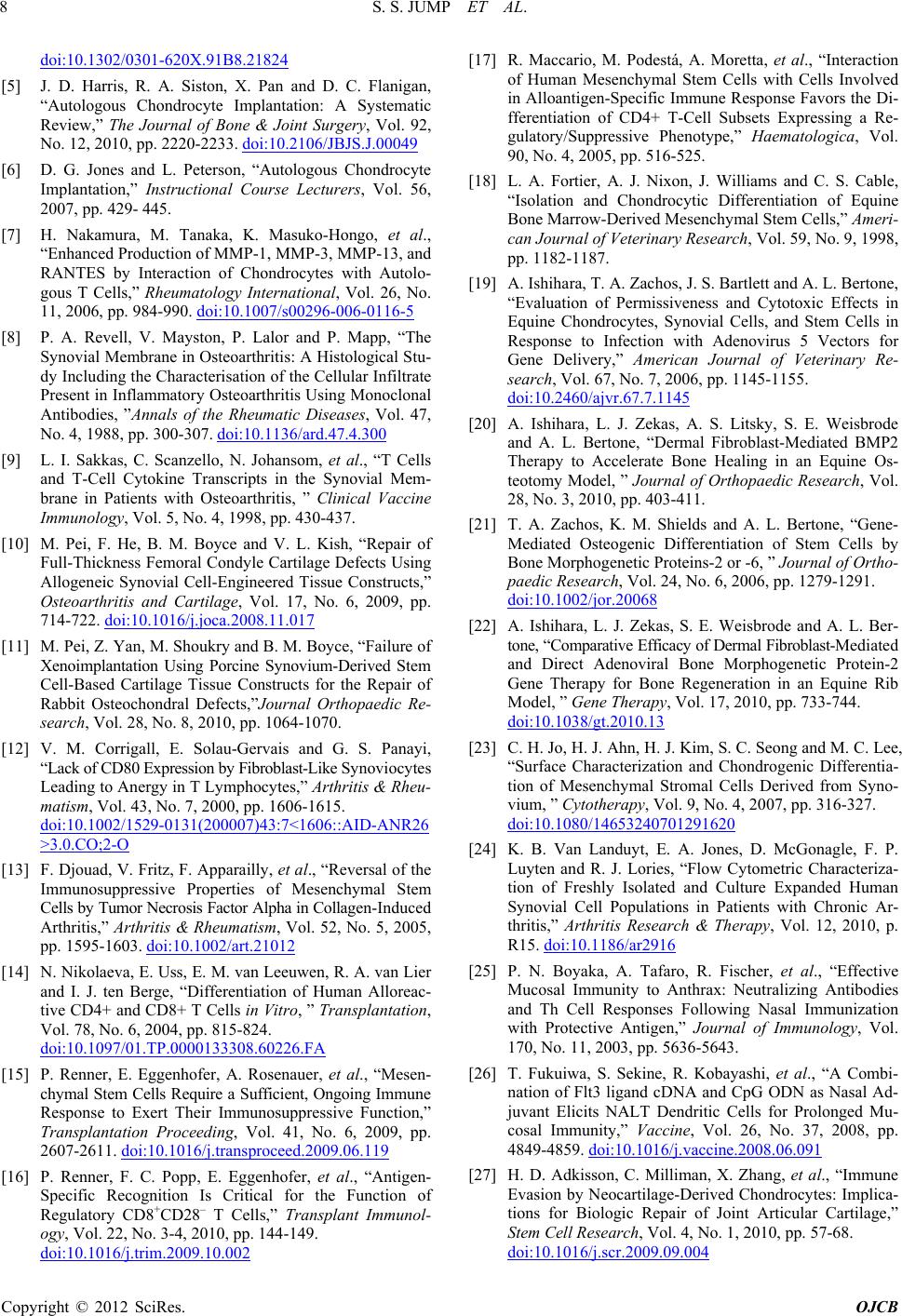 S. S. JUMP ET AL. 8 doi:10.1302/0301-620X.91B8.21824 [5] J. D. Harris, R. A. Siston, X. Pan and D. C. Flanigan, “Autologous Chondrocyte Implantation: A Systematic Review,” The Journal of Bone & Joint Surgery, Vol. 92, No. 12, 2010, pp. 2220-2233. doi:10.2106/JBJS.J.00049 [6] D. G. Jones and L. Peterson, “Autologous Chondrocyte Implantation,” Instructional Course Lecturers, Vol. 56, 2007, pp. 429- 445. [7] H. Nakamura, M. Tanaka, K. Masuko-Hongo, et al., “Enhanced Production of MMP-1, MMP-3, MMP-13, and RANTES by Interaction of Chondrocytes with Autolo- gous T Cells,” Rheumatology International, Vol. 26, No. 11, 2006, pp. 984-990. doi:10.1007/s00296-006-0116-5 [8] P. A. Revell, V. Mayston, P. Lalor and P. Mapp, “The Synovial Membrane in Osteoarthritis: A Histological Stu- dy Including the Characterisation of the Cellular Infiltrate Present in Inflammatory Osteoarthritis Using Monoclonal Antibodies, ”Annals of the Rheumatic Diseases, Vol. 47, No. 4, 1988, pp. 300-307. doi:10.1136/ard.47.4.300 [9] L. I. Sakkas, C. Scanzello, N. Johansom, et al., “T Cells and T-Cell Cytokine Transcripts in the Synovial Mem- brane in Patients with Osteoarthritis, ” Clinical Vaccine Immunology, Vol. 5, No. 4, 1998, pp. 430-437. [10] M. Pei, F. He, B. M. Boyce and V. L. Kish, “Repair of Full-Thickness Femoral Condyle Cartilage Defects Using Allogeneic Synovial Cell-Engineered Tissue Constructs,” Osteoarthritis and Cartilage, Vol. 17, No. 6, 2009, pp. 714-722. doi:10.1016/j.joca.2008.11.017 [11] M. Pei, Z. Yan, M. Shoukry and B. M. Boyce, “Failure of Xenoimplantation Using Porcine Synovium-Derived Stem Cell-Based Cartilage Tissue Constructs for the Repair of Rabbit Osteochondral Defects,”Journal Orthopaedic Re- search, Vol. 28, No. 8, 2010, pp. 1064-1070. [12] V. M. Corrigall, E. Solau-Gervais and G. S. Panayi, “Lack of CD80 Expression by Fibroblas t- Like Synoviocytes Leading to Anergy in T Lymphocytes,” Arthritis & Rheu- matism, Vol. 43, No. 7, 2000, pp. 1606-1615. doi:10.1002/1529-0131(200007)43:7<1606::AID-ANR26 >3.0.CO;2-O [13] F. Djouad, V. Fritz, F. Appa railly, et al., “Reversal of the Immunosuppressive Properties of Mesenchymal Stem Cells by Tumor Necrosis Factor Alpha in Collage n- In d uc e d Arthritis,” Arthritis & Rheumatism, Vol. 52, No. 5, 2005, pp. 1595-1603. doi:10.1002/art.21012 [14] N. Nikolaeva, E. Uss, E. M. van Leeuwen, R. A. van Lier and I. J. ten Berge, “Differentiation of Human Alloreac- tive CD4+ and CD8+ T Cells in Vitro, ” Transplantation, Vol. 78, No. 6, 2004, pp. 815-824. doi:10.1097/01.TP.0000133308.60226.FA [15] P. Renner, E. Eggenhofer, A. Rosenauer, et al., “Mesen- chymal Stem Cells Require a Sufficient, Ongoing Immune Response to Exert Their Immunosuppressive Function,” Transplantation Proceeding, Vol. 41, No. 6, 2009, pp. 2607-2611. doi:10.1016/j.transproceed.2009.06.119 [16] P. Renner, F. C. Popp, E. Eggenhofer, et al., “Antigen- Specific Recognition Is Critical for the Function of Regulatory CD8+CD28– T Cells,” Transplant Immunol- ogy, Vol. 22, No. 3-4, 2010, pp. 144-149. doi:10.1016/j.trim.2009.10.002 [17] R. Maccario, M. Podestá, A. Moretta, et al., “Interaction of Human Mesenchymal Stem Cells with Cells Involved in Alloantigen-Specific Immune Response Favors the Di- fferentiation of CD4+ T-Cell Subsets Expressing a Re- gulatory/Suppressive Phenotype,” Haematologica, Vol. 90, No. 4, 2005, pp. 516-525. [18] L. A. Fortier, A. J. Nixon, J. Williams and C. S. Cable, “Isolation and Chondrocytic Differentiation of Equine Bone Marrow-Derived Mesenc hymal Stem Cells,” Ameri- can Journal of Veterinary Research, Vol. 59, No. 9, 1998, pp. 1182-1187. [19] A. Ishihara, T. A. Zachos, J. S. Bartlett and A. L. Bertone, “Evaluation of Permissiveness and Cytotoxic Effects in Equine Chondrocytes, Synovial Cells, and Stem Cells in Response to Infection with Adenovirus 5 Vectors for Gene Delivery,” American Journal of Veterinary Re- search, Vol. 67, No. 7, 2006, pp. 1145-1155. doi:10.2460/ajvr.67.7.1145 [20] A. Ishihara, L. J. Zekas, A. S. Litsky, S. E. Weisbrode and A. L. Bertone, “Dermal Fibroblast-Mediated BMP2 Therapy to Accelerate Bone Healing in an Equine Os- teotomy Model, ” Journal of Orthopaedic Research, Vol. 28, No. 3, 2010, pp. 403-411. [21] T. A. Zachos, K. M. Shields and A. L. Bertone, “Gene- Mediated Osteogenic Differentiation of Stem Cells by Bone Morphogenetic Proteins-2 or -6, ” Journal of Ortho- paedic Research, Vol. 24, No. 6, 2006, pp. 1279-1291. doi:10.1002/jor.20068 [22] A. Ishihara, L. J. Zekas, S. E. Weisbrode and A. L. Ber- tone, “Comparative Efficacy of Dermal Fibroblast-Me d i a t e d and Direct Adenoviral Bone Morphogenetic Protein-2 Gene Therapy for Bone Regeneration in an Equine Rib Model, ” Gene Therapy, Vol. 17, 2010, pp. 733-744. doi:10.1038/gt.2010.13 [23] C. H. Jo, H. J. Ahn, H. J. Kim, S. C. Seong and M. C. Lee, “Surface Characterization and Chondrogenic Differentia- tion of Mesenchymal Stromal Cells Derived from Syno- vium, ” Cytotherapy, Vol. 9, No. 4, 2007, pp. 316-327. doi:10.1080/14653240701291620 [24] K. B. Van Landuyt, E. A. Jones, D. McGonagle, F. P. Luyten and R. J. Lories, “Flow Cytometric Characteriza- tion of Freshly Isolated and Culture Expanded Human Synovial Cell Populations in Patients with Chronic Ar- thritis,” Arthritis Research & Therapy, Vol. 12, 2010, p. R15. doi:10.1186/ar2916 [25] P. N. Boyaka, A. Tafaro, R. Fischer, et al., “Effective Mucosal Immunity to Anthrax: Neutralizing Antibodies and Th Cell Responses Following Nasal Immunization with Protective Antigen,” Journal of Immunology, Vol. 170, No. 11, 2003, pp. 5636-5643. [26] T. Fukuiwa, S. Sekine, R. Kobayashi, et al., “A Combi- nation of Flt3 ligand cDNA and CpG ODN as Nasal Ad- juvant Elicits NALT Dendritic Cells for Prolonged Mu- cosal Immunity,” Vaccine, Vol. 26, No. 37, 2008, pp. 4849-4859. doi:10.1016/j.vaccine.2008.06.091 [27] H. D. Adkisson, C. Milliman, X. Zhang, et al., “Immune Evasion by Neocartilage-Derived Chondrocytes: Implica- tions for Biologic Repair of Joint Articular Cartilage,” Stem Cell Research, Vol. 4, No. 1, 2010, pp. 57-68. doi:10.1016/j.scr.2009.09.004 Copyright © 2012 SciRes. OJCB
 S. S. JUMP ET AL. Copyright © 2012 SciRes. OJCB 9 [28] C. J. Centeno, D. Busse, J. Kisiday, et al., “Regeneration of Meniscus Cartilage in a Knee Treated with Percutane- ously Implanted Autologous Mesenchymal Stem Cells,” Medical Hypotheses, Vol. 71, No. 6, 2008, pp. 900-908. doi:10.1016/j.mehy.2008.06.042 [29] M. Horie, I. Sekiya, T. Muneta, et al., “Intra-Articular Injected Synovial Stem Cells Differentiate into Meniscal Cells Directly and Promote Meniscal Regeneration with- out Mobilization to Distant Organs in Rat Massive Me- niscal Defect,” Stem Cells, Vol. 27, No. 4, 2009, pp. 878- 887. doi:10.1634/stemcells.2008-0616 [30] Q. V. Jichen, G. Che, G. Jiang, et al., “Immune Suppres- sion Produced by Intrathymic Inoculation with Xenoge- neic Antigen and Whole-Body γ-Irradiation in a Pig-to- Monkey Heart Transplantation Model,” Transplantation Proceeding, Vol. 42, No. 9, 2010, pp. 3759-3762. doi:10.1016/j.transproceed.2010.06.040 [31] Y. Wang, X. Che, M. A. Armstrong and G. Li, “Survival of Bone Marrow-Derived Mesenchymal Stem Cells in a Xenotransplantation Model,” Journal Orthopaedic Re- search, Vol. 25, No. 7, 2007, pp. 926-932. doi:10.1002/jor.20385 [32] J. L. C. van Susante, P. Buma, L. Schuman, et al., “Re- surfacing Potential of Heterologous Chondrocytes Sus- pended in Fibrin Glue in Large Full-Thickness Defects of Femoral Articular Cartilage: An Experimental Study in the Goat,” Biomaterials, Vol. 20, No. 13, 1999, pp. 1167- 1175. doi:10.1016/S0142-9612(97)00190-7 [33] F. Djouad, C. Bony, T. Häupl, et al., “Transcriptional Profiles Discriminate Bone Marrow-Derived and Syno- vium-Derived Mesenchymal Stem Cells,” Arthritis Re- search & Therapy, Vol. 7, 2005, pp. R1304-R1315. doi:10.1186/ar1827
|