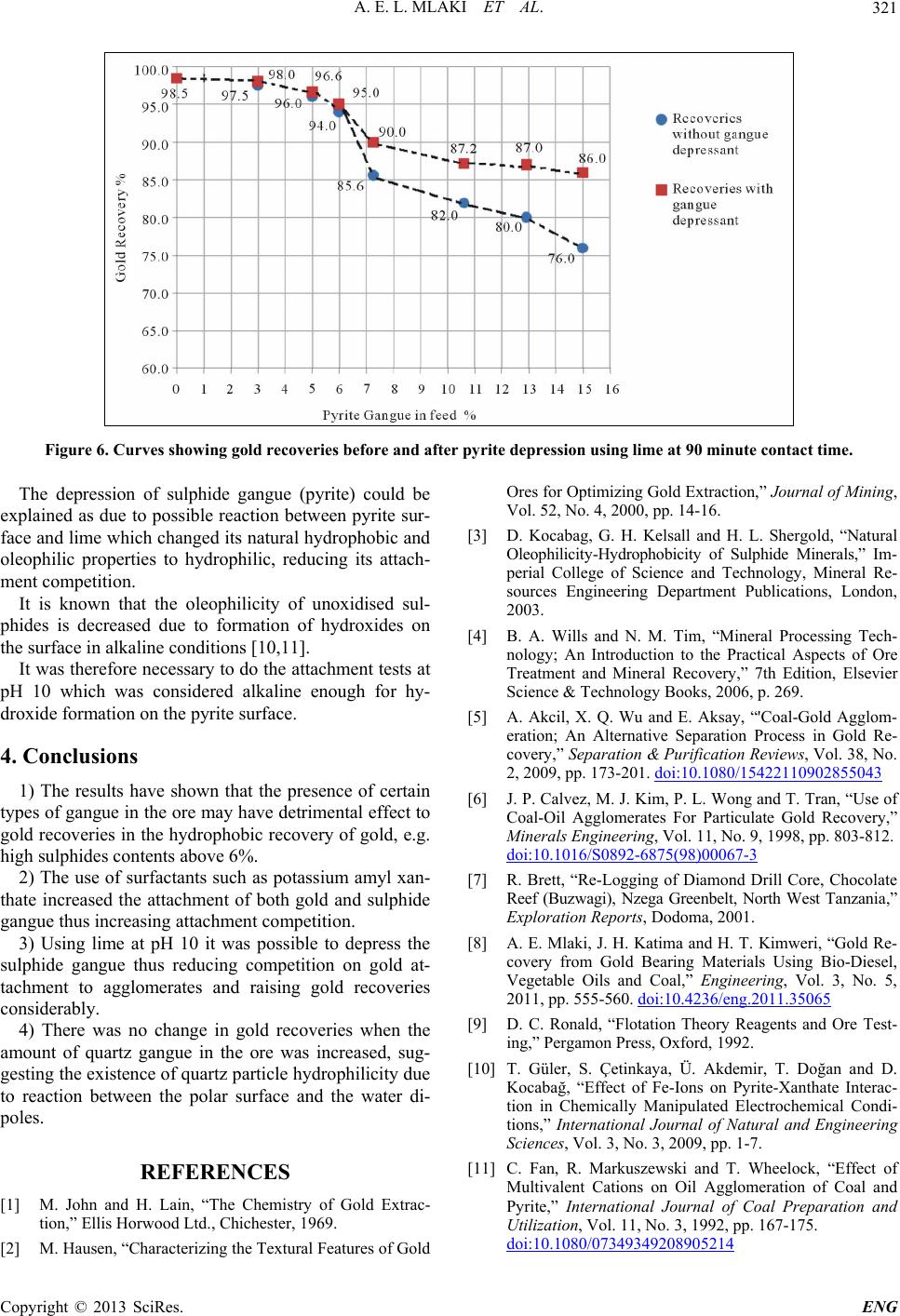
A. E. L. MLAKI ET AL. 321
Figure 6. Curves showing gold recoveries before and after pyrite depression using lime at 90 minute contact time.
The depression of sulphide gangue (pyrite) could be
explained as due to possible reaction between pyrite sur-
face and lime which changed its natural hydrophobic and
oleophilic properties to hydrophilic, reducing its attach-
ment competition.
It is known that the oleophilicity of unoxidised sul-
phides is decreased due to formation of hydroxides on
the surface in alkaline conditions [10,11].
It was therefore necessary to do the attachment tests at
pH 10 which was considered alkaline enough for hy-
droxide formation on the pyrite surface.
4. Conclusions
1) The results have shown that the presence of certain
types of gangue in the ore may have detrimental effect to
gold recoveries in the hydrophobic recovery of gold, e.g.
high sulphides contents above 6%.
2) The use of surfactants such as potassium amyl xan-
thate increased the attachment of both gold and sulphide
gangue thus increasing attachment competition.
3) Using lime at pH 10 it was possible to depress the
sulphide gangue thus reducing competition on gold at-
tachment to agglomerates and raising gold recoveries
considerably.
4) There was no change in gold recoveries when the
amount of quartz gangue in the ore was increased, sug-
gesting the existence of quartz particle hydrophilicity due
to reaction between the polar surface and the water di-
poles.
REFERENCES
[1] M. John and H. Lain, “The Chemistry of Gold Extrac-
tion,” Ellis Horwood Ltd., Chichester, 1969.
[2] M. Hausen, “Characterizing the Textural Features of Gold
Ores for Optimizing Gold Extraction,” Journal of Mining,
Vol. 52, No. 4, 2000, pp. 14-16.
[3] D. Kocabag, G. H. Kelsall and H. L. Shergold, “Natural
Oleophilicity-Hydrophobicity of Sulphide Minerals,” Im-
perial College of Science and Technology, Mineral Re-
sources Engineering Department Publications, London,
2003.
[4] B. A. Wills and N. M. Tim, “Mineral Processing Tech-
nology; An Introduction to the Practical Aspects of Ore
Treatment and Mineral Recovery,” 7th Edition, Elsevier
Science & Technology Books, 2006, p. 269.
[5] A. Akcil, X. Q. Wu and E. Aksay, “'Coal-Gold Agglom-
eration; An Alternative Separation Process in Gold Re-
covery,” Separation & Purification Reviews, Vol. 38, No.
2, 2009, pp. 173-201. doi:10.1080/15422110902855043
[6] J. P. Calvez, M. J. Kim, P. L. Wong and T. Tran, “Use of
Coal-Oil Agglomerates For Particulate Gold Recovery,”
Minerals Engineering, Vol. 11, No. 9, 1998, pp. 803-812.
doi:10.1016/S0892-6875(98)00067-3
[7] R. Brett, “Re-Logging of Diamond Drill Core, Chocolate
Reef (Buzwagi), Nzega Greenbelt, North West Tanzania,”
Exploration Reports, Dodoma, 2001.
[8] A. E. Mlaki, J. H. Katima and H. T. Kimweri, “Gold Re-
covery from Gold Bearing Materials Using Bio-Diesel,
Vegetable Oils and Coal,” Engineering, Vol. 3, No. 5,
2011, pp. 555-560. doi:10.4236/eng.2011.35065
[9] D. C. Ronald, “Flotation Theory Reagents and Ore Test-
ing,” Pergamon Press, Oxford, 1992.
[10] T. Güler, S. Çetinkaya, Ü. Akdemir, T. Doğan and D.
Kocabağ, “Effect of Fe-Ions on Pyrite-Xanthate Interac-
tion in Chemically Manipulated Electrochemical Condi-
tions,” International Journal of Natural and Engineering
Sciences, Vol. 3, No. 3, 2009, pp. 1-7.
[11] C. Fan, R. Markuszewski and T. Wheelock, “Effect of
Multivalent Cations on Oil Agglomeration of Coal and
Pyrite,” International Journal of Coal Preparation and
Utilization, Vol. 11, No. 3, 1992, pp. 167-175.
doi:10.1080/07349349208905214
Copyright © 2013 SciRes. ENG