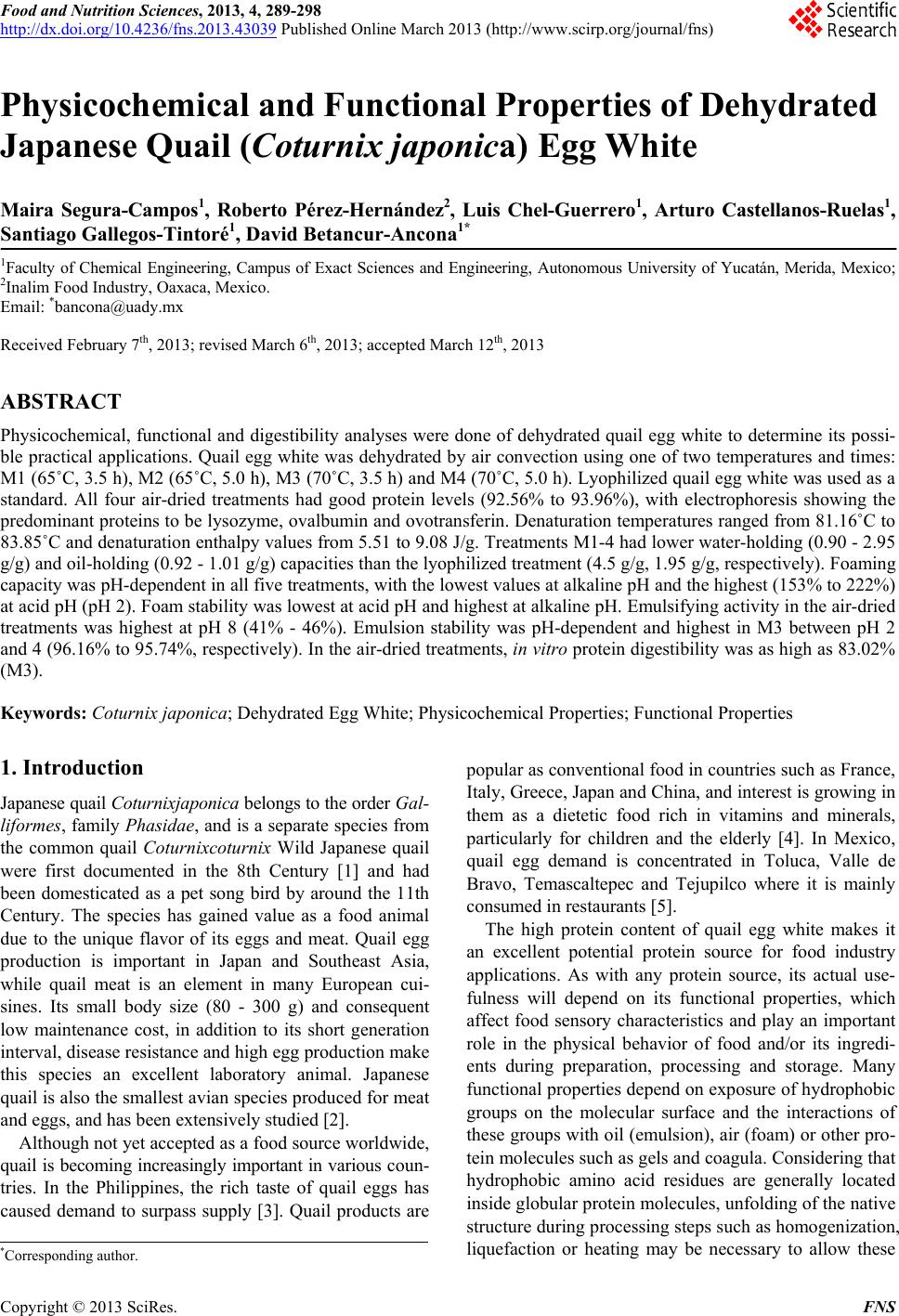 Food and Nutrition Sciences, 2013, 4, 289-298 http://dx.doi.org/10.4236/fns.2013.43039 Published Online March 2013 (http://www.scirp.org/journal/fns) Physicochemical and Functional Properties of Dehydrated Japanese Quail (Coturnix japonica) Egg White Maira Segura-Campos1, Roberto Pérez-Hernández2, Luis Chel-Guerrero1, Arturo Castellanos-Ruelas1, Santiago Gallegos-Tintoré1, David Betancur-Ancona1* 1Faculty of Chemical Engineering, Campus of Exact Sciences and Engineering, Autonomous University of Yucatán, Merida, Mexico; 2Inalim Food Industry, Oaxaca, Mexico. Email: *bancona@uady.mx Received February 7th, 2013; revised March 6th, 2013; accepted March 12th, 2013 ABSTRACT Physicochemical, functional and digestibility analyses were done of dehydrated quail egg white to determine its possi- ble practical applications. Quail egg white was dehydrated by air convection using one of two temperatures and times: M1 (65˚C, 3.5 h), M2 (65˚C, 5.0 h), M3 (70˚C, 3.5 h) and M4 (70˚C, 5.0 h). Lyophilized quail egg white was used as a standard. All four air-dried treatments had good protein levels (92.56% to 93.96%), with electrophoresis showing the predominant proteins to be lysozyme, ovalbumin and ovotransferin. Denaturation temperatures ranged from 81.16˚C to 83.85˚C and denaturation enthalpy values from 5.51 to 9.08 J/g. Treatments M1-4 had lower water-holding (0.90 - 2.95 g/g) and oil-holding (0.92 - 1.01 g/g) capacities than the lyophilized treatment (4.5 g/g, 1.95 g/g, respectively). Foaming capacity was pH-dependent in all five treatments, with the lowest values at alkaline pH and the highest (153% to 222%) at acid pH (pH 2). Foam stability was lowest at acid pH and highest at alkaline pH. Emulsifying activity in the air-dried treatments was highest at pH 8 (41% - 46%). Emulsion stability was pH-dependent and highest in M3 between pH 2 and 4 (96.16% to 95.74%, respectively). In the air-dried treatments, in vitro protein digestibility was as high as 83.02% (M3). Keywords: Coturnix japonica; Dehydrated Egg White; Physicochemical Properties; Functional Properties 1. Introduction Japanese quail Coturnixjaponica belongs to the order Gal- liformes, family Phasidae, and is a separate species from the common quail Coturnixcoturnix Wild Japanese quail were first documented in the 8th Century [1] and had been domesticated as a pet song bird by around the 11th Century. The species has gained value as a food animal due to the unique flavor of its eggs and meat. Quail egg production is important in Japan and Southeast Asia, while quail meat is an element in many European cui- sines. Its small body size (80 - 300 g) and consequent low maintenance cost, in addition to its short generation interval, disease resistance and high egg production make this species an excellent laboratory animal. Japanese quail is also the smallest avian species produced for meat and eggs, and has been extensively studied [2]. Although not yet accepted as a food source worldwide, quail is becoming increasingly important in various coun- tries. In the Philippines, the rich taste of quail eggs has caused demand to surpass supply [3]. Quail products are popular as conventional food in countries such as France, Italy, Greece, Japan and China, and interest is growing in them as a dietetic food rich in vitamins and minerals, particularly for children and the elderly [4]. In Mexico, quail egg demand is concentrated in Toluca, Valle de Bravo, Temascaltepec and Tejupilco where it is mainly consumed in restaurants [5]. The high protein content of quail egg white makes it an excellent potential protein source for food industry applications. As with any protein source, its actual use- fulness will depend on its functional properties, which affect food sensory characteristics and play an important role in the physical behavior of food and/or its ingredi- ents during preparation, processing and storage. Many functional properties depend on exposure of hydrophobic groups on the molecular surface and the interactions of these groups with oil (emulsion), air (foam) or other pro- tein molecules such as gels and coagula. Considering that hydrophobic amino acid residues are generally located inside globular protein molecules, unfolding of the native structure during processing steps such as homogenization, liquefaction or heating may be necessary to allow these *Corresponding author. Copyright © 2013 SciRes. FNS
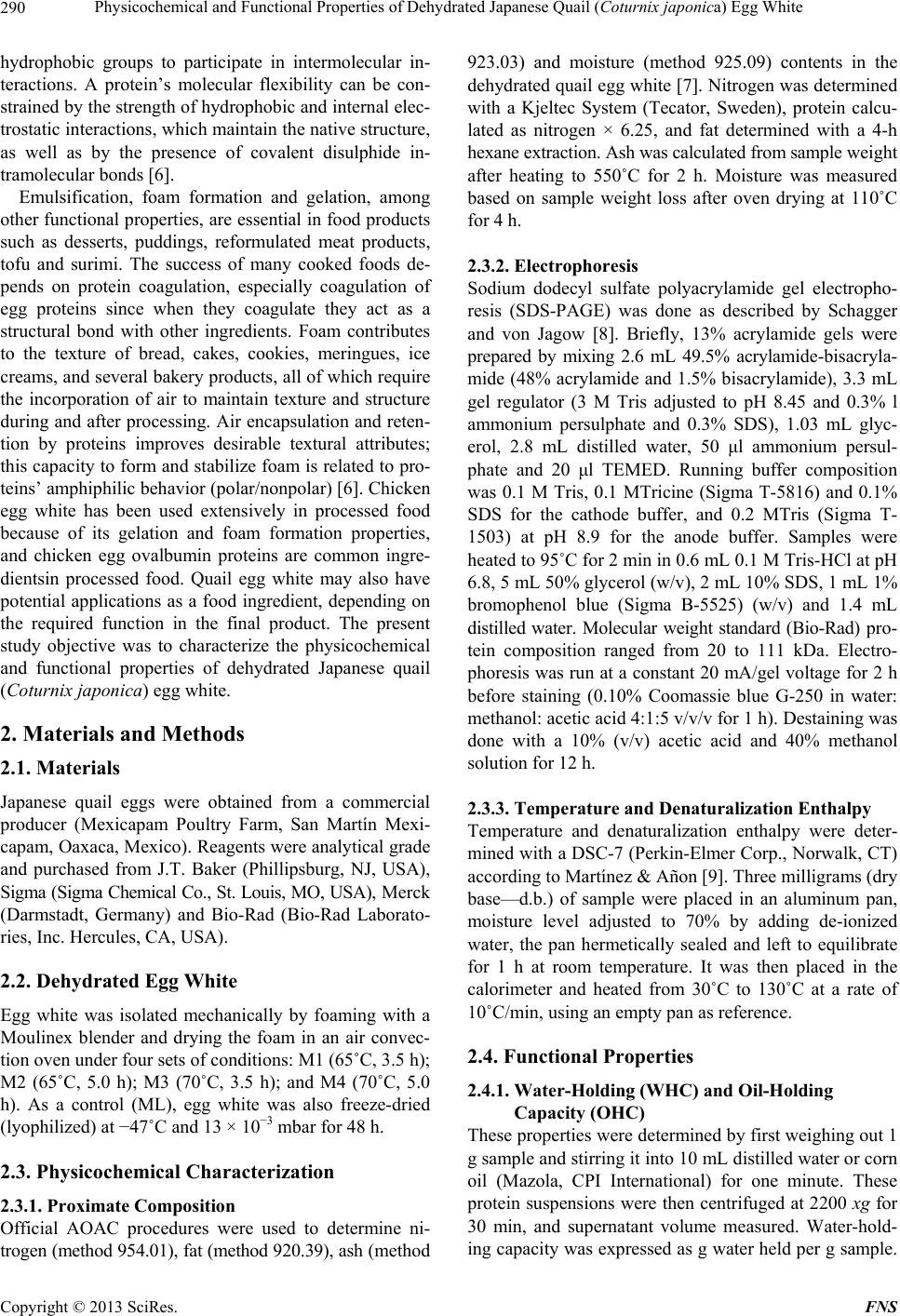 Physicochemical and Functional Properties of Dehydrated Japanese Quail (Coturnix japonica) Egg White 290 hydrophobic groups to participate in intermolecular in- teractions. A protein’s molecular flexibility can be con- strained by the strength of hydrophobic and internal elec- trostatic interactions, which maintain the native structure, as well as by the presence of covalent disulphide in- tramolecular bonds [6]. Emulsification, foam formation and gelation, among other functional properties, are essential in food products such as desserts, puddings, reformulated meat products, tofu and surimi. The success of many cooked foods de- pends on protein coagulation, especially coagulation of egg proteins since when they coagulate they act as a structural bond with other ingredients. Foam contributes to the texture of bread, cakes, cookies, meringues, ice creams, and several bakery products, all of which require the incorporation of air to maintain texture and structure during and after processing. Air encapsulation and reten- tion by proteins improves desirable textural attributes; this capacity to form and stabilize foam is related to pro- teins’ amphiphilic behavior (polar/nonpolar) [6]. Chicken egg white has been used extensively in processed food because of its gelation and foam formation properties, and chicken egg ovalbumin proteins are common ingre- dientsin processed food. Quail egg white may also have potential applications as a food ingredient, depending on the required function in the final product. The present study objective was to characterize the physicochemical and functional properties of dehydrated Japanese quail (Coturnix japonica) egg white. 2. Materials and Methods 2.1. Materials Japanese quail eggs were obtained from a commercial producer (Mexicapam Poultry Farm, San Martín Mexi- capam, Oaxaca, Mexico). Reagents were analytical grade and purchased from J.T. Baker (Phillipsburg, NJ, USA), Sigma (Sigma Chemical Co., St. Louis, MO, USA), Merck (Darmstadt, Germany) and Bio-Rad (Bio-Rad Laborato- ries, Inc. Hercules, CA, USA). 2.2. Dehydrated Egg White Egg white was isolated mechanically by foaming with a Moulinex blender and drying the foam in an air convec- tion oven under four sets of conditions: M1 (65˚C, 3.5 h); M2 (65˚C, 5.0 h); M3 (70˚C, 3.5 h); and M4 (70˚C, 5.0 h). As a control (ML), egg white was also freeze-dried (lyophilized) at −47˚C and 13 × 10−3 mbar for 48 h. 2.3. Physicochemical Characterization 2.3.1. Proximate Composition Official AOAC procedures were used to determine ni- trogen (method 954.01), fat (method 920.39), ash (method 923.03) and moisture (method 925.09) contents in the dehydrated quail egg white [7]. Nitrogen was determined with a Kjeltec System (Tecator, Sweden), protein calcu- lated as nitrogen × 6.25, and fat determined with a 4-h hexane extraction. Ash was calculated from sample weight after heating to 550˚C for 2 h. Moisture was measured based on sample weight loss after oven drying at 110˚C for 4 h. 2.3.2. Electrophoresis Sodium dodecyl sulfate polyacrylamide gel electropho- resis (SDS-PAGE) was done as described by Schagger and von Jagow [8]. Briefly, 13% acrylamide gels were prepared by mixing 2.6 mL 49.5% acrylamide-bisacryla- mide (48% acrylamide and 1.5% bisacrylamide), 3.3 mL gel regulator (3 M Tris adjusted to pH 8.45 and 0.3% l ammonium persulphate and 0.3% SDS), 1.03 mL glyc- erol, 2.8 mL distilled water, 50 μl ammonium persul- phate and 20 μl TEMED. Running buffer composition was 0.1 M Tris, 0.1 MTricine (Sigma T-5816) and 0.1% SDS for the cathode buffer, and 0.2 MTris (Sigma T- 1503) at pH 8.9 for the anode buffer. Samples were heated to 95˚C for 2 min in 0.6 mL 0.1 M Tris-HCl at pH 6.8, 5 mL 50% glycerol (w/v), 2 mL 10% SDS, 1 mL 1% bromophenol blue (Sigma B-5525) (w/v) and 1.4 mL distilled water. Molecular weight standard (Bio-Rad) pro- tein composition ranged from 20 to 111 kDa. Electro- phoresis was run at a constant 20 mA/gel voltage for 2 h before staining (0.10% Coomassie blue G-250 in water: methanol: acetic acid 4:1:5 v/v/v for 1 h). Destaining was done with a 10% (v/v) acetic acid and 40% methanol solution for 12 h. 2.3.3. Temperature and Denaturalization Enthalpy Temperature and denaturalization enthalpy were deter- mined with a DSC-7 (Perkin-Elmer Corp., Norwalk, CT) according to Martínez & Añon [9]. Three milligrams (dry base—d.b.) of sample were placed in an aluminum pan, moisture level adjusted to 70% by adding de-ionized water, the pan hermetically sealed and left to equilibrate for 1 h at room temperature. It was then placed in the calorimeter and heated from 30˚C to 130˚C at a rate of 10˚C/min, using an empty pan as reference. 2.4. Functional Properties 2.4.1. Water-Holding (WHC) and Oil-Holding Capacity (OHC) These properties were determined by first weighing out 1 g sample and stirring it into 10 mL distilled water or corn oil (Mazola, CPI International) for one minute. These protein suspensions were then centrifuged at 2200 xg for 30 min, and supernatant volume measured. Water-hold- ing capacity was expressed as g water held per g sample. Copyright © 2013 SciRes. FNS
 Physicochemical and Functional Properties of Dehydrated Japanese Quail (Coturnix japonica) Egg White Copyright © 2013 SciRes. FNS 291 Oil-holding capacity was expressed as g oil held per g protein sample [10]. 2.4.2. Foaming Capacity and Foam Stability Foam properties were evaluated over a pH range of 2 to 10. A 100 mL sample of 1.5% protein (w/v) suspension was blended at low speed in a Waring blender (Osterizer 10S-E) for 5 min, and foam volume recorded after 30 s. Foaming capacity was expressed as the percentage in- crease in foam volume measured at 30 s. Foam stability was quantified by measuring residual foam volume 5, 30 and 120 min after blending. Both properties were deter- mined as a function of pH [10]. 2.4.3. Emulsifying Activity (EA) and Emulsion Stability (ES) Emulsion properties were analyzed using 10 mL samples of a 2% (w/v) suspension adjusted to pH values ranging from 2 to 10. These were homogenized using a Caframo RZ-1 homogenizer at 2000 rpm for 2 min, 10 mL corn oil (Mazola, CPI International) added to each sample and the mixture homogenized for 1 min. The emulsions were centrifuged in 15 mL graduated centrifuge tubes at 1200 xg for 5 min and emulsion volume measured. Emulsify- ing activity was expressed as the percentage represented by the emulsified layer volume of the entire layer in the centrifuge tube. To determine emulsion stability, the emul- sions were heated to 80˚C for 30 min, cooled to room temperature and centrifuged at 1200 xg for 5 min. Emul- sion stability was expressed as the percentage repre- sented by the remaining emulsified layer volume of the original emulsion volume [10]. 2.5. In Vitro Protein Digestibility Following Hsu et al. [11], a multienzyme system con- sisting of porcine pancreatic trypsin type IX, bovine pan- creatic chymotrypsin type II, and porcine intestinal pep- tidase grade III (Sigma Chemical Co, St. Louis, MO, USA) was selected. A total volume of 50 mL of an aqueous protein suspension (6.25 mg/mL) was prepared by mixing the sample with distilled water, adjusting pH to 8.0, and stirring in a water bath at 37˚C. The multien- zyme solution was maintained in an ice bath and adjusted to pH 8.0. While stirring, a 5 mL aliquot of multienzyme solution was added to the protein suspension at 37˚C. A rapid decline in pH occurred which was recorded over a 10 min period using a pH meter. In vitro protein digesti- bility was calculated with the equation PD = 210.46 − 18.10 (pH after 10 min). 2.6. Statistical Analysis All results were analyzed using descriptive statistics with a central tendency and dispersion measures. Data were analyzed using a one-way analysis of variance (ANOVA) and a Duncan’s multiple range test; all calculations were done in triplicate. All analyses were done according to Montgomery [12] and processed using the Statgraphics Plus version 5.1 software. 3. Results and Discussion 3.1. Proximate Composition Proximate analyses showed moisture content of the de- hydrated quail egg white to range from 6.16 (M2) to 7.42 (M3) (Table 1). Initial moisture content in quail albumen is reported to be 87.82% (Dudusola, 2010), meaning a substantial loss of moisture occurred. Ash content ranged from 5.96% (M2) to 7.08% (M4), which are higher than reported for quail (1.00%) and guinea fowl (0.79%) al- bumen [13]. This relatively high ash content suggests that dehydrated quail egg white is a good source of min- erals. Protein content ranged from 92.56% (M4) to 93.96% (M1). These values are higher than the 80.72% [14] and 90% [15] reported for aspersion-dried chicken egg, the 80% reported for dehydrated chicken egg white [16], and the 83.9% reported for quail albumen [13]. The protein content observed here in dehydrated quail egg white was also higher than protein content in young (18.99 %) and spent (17.48%) quail meat [17]. Fat con- tent ranged from 0.42 (M2) to 0.58 (M1), which is higher than reported for dehydrated chicken egg white (0.04%), and quail (0.09%) and guinea fowl (0.13%) albumen [14]. 3.2. Electrophoresis Electrophoresis showed the lyophilized quail egg white Table 1. Moisture, ash, protein and fat contents in quail egg white dried by air convection at two temperatures and two times. Components M1 65˚C, 3.5 h M2 65˚C, 5.0 h M3 70˚C, 3.5 h M4 70˚C, 5.0 h Moisture (6.36 0.08)b (6.16 0.02)b (7.42 0.03)a (6.24 0.05)b Ash 6.06 0.15b 5.96 0.22c 6.63 0.28b 7.08 0.09a Protein 93.96 0.50a 93.71 0.07a 93.16 0.24a 92.56 0.06c Fat 0.58 0.01a 0.42 0.01b 0.49 0.01b 0.56 0.06a
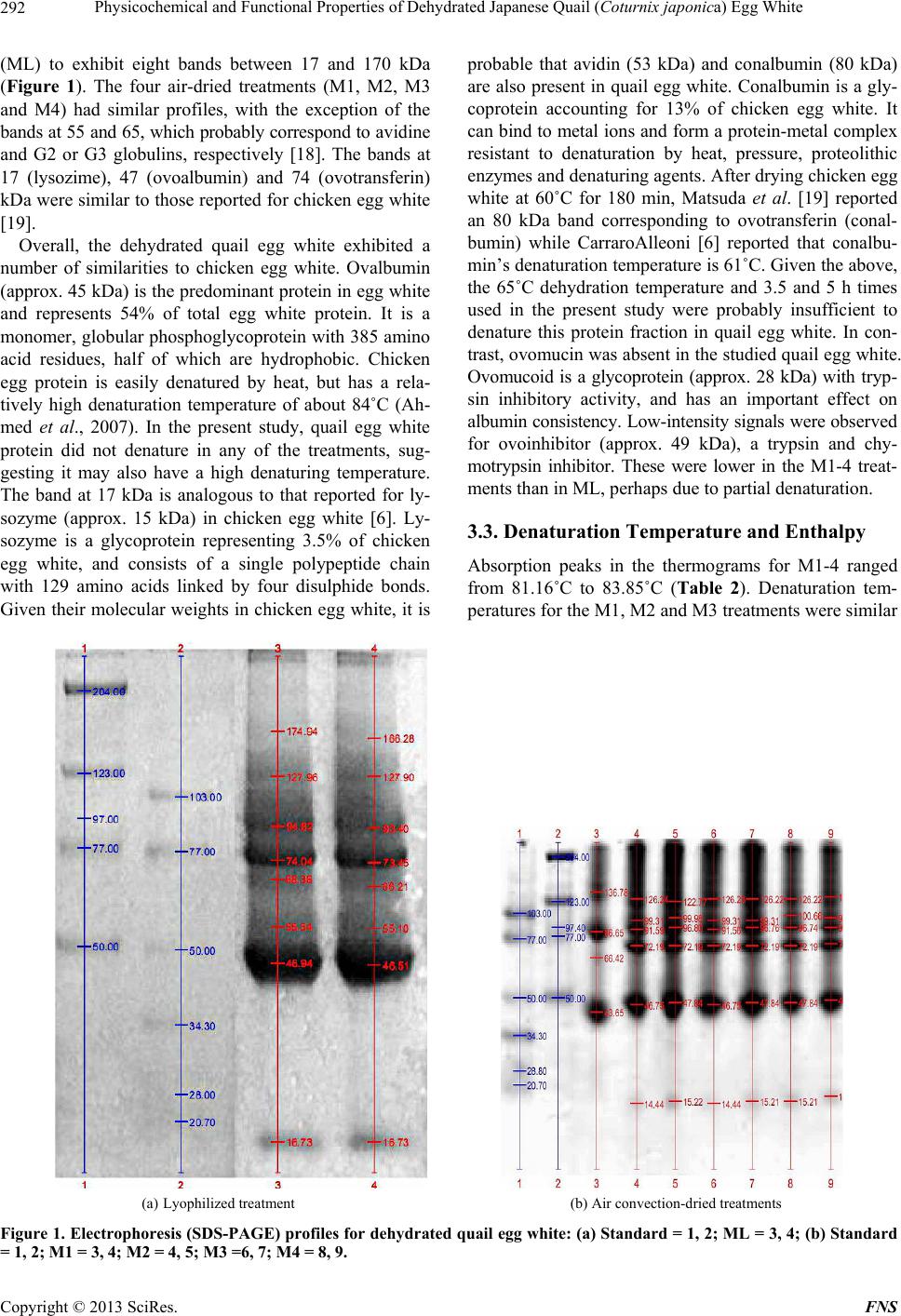 Physicochemical and Functional Properties of Dehydrated Japanese Quail (Coturnix japonica) Egg White 292 (ML) to exhibit eight bands between 17 and 170 kDa (Figure 1). The four air-dried treatments (M1, M2, M3 and M4) had similar profiles, with the exception of the bands at 55 and 65, which probably correspond to avidine and G2 or G3 globulins, respectively [18]. The bands at 17 (lysozime), 47 (ovoalbumin) and 74 (ovotransferin) kDa were similar to those reported for chicken egg white [19]. Overall, the dehydrated quail egg white exhibited a number of similarities to chicken egg white. Ovalbumin (approx. 45 kDa) is the predominant protein in egg white and represents 54% of total egg white protein. It is a monomer, globular phosphoglycoprotein with 385 amino acid residues, half of which are hydrophobic. Chicken egg protein is easily denatured by heat, but has a rela- tively high denaturation temperature of about 84˚C (Ah- med et al., 2007). In the present study, quail egg white protein did not denature in any of the treatments, sug- gesting it may also have a high denaturing temperature. The band at 17 kDa is analogous to that reported for ly- sozyme (approx. 15 kDa) in chicken egg white [6]. Ly- sozyme is a glycoprotein representing 3.5% of chicken egg white, and consists of a single polypeptide chain with 129 amino acids linked by four disulphide bonds. Given their molecular weights in chicken egg white, it is probable that avidin (53 kDa) and conalbumin (80 kDa) are also present in quail egg white. Conalbumin is a gly- coprotein accounting for 13% of chicken egg white. It can bind to metal ions and form a protein-metal complex resistant to denaturation by heat, pressure, proteolithic enzymes and denaturing agents. After drying chicken egg white at 60˚C for 180 min, Matsuda et al. [19] reported an 80 kDa band corresponding to ovotransferin (conal- bumin) while CarraroAlleoni [6] reported that conalbu- min’s denaturation temperature is 61˚C. Given the above, the 65˚C dehydration temperature and 3.5 and 5 h times used in the present study were probably insufficient to denature this protein fraction in quail egg white. In con- trast, ovomucin was absent in the studied quail egg white. Ovomucoid is a glycoprotein (approx. 28 kDa) with tryp- sin inhibitory activity, and has an important effect on albumin consistency. Low-intensity signals were observed for ovoinhibitor (approx. 49 kDa), a trypsin and chy- motrypsin inhibitor. These were lower in the M1-4 treat- ments than in ML, perhaps due to partial denaturation. 3.3. Denaturation Temperature and Enthalpy Absorption peaks in the thermograms for M1-4 ranged from 81.16˚C to 83.85˚C (Table 2). Denaturation tem- peratures for the M1, M2 and M3 treatments were similar (a) Lyophilized treatment (b) Air convection-dried treatments Figure 1. Electrophoresis (SDS-PAGE) profiles for dehydrated quail egg white: (a) Standard = 1, 2; ML = 3, 4; (b) Standard = 1, 2; M1 = 3, 4; M2 = 4, 5; M3 =6, 7; M4 = 8, 9. Copyright © 2013 SciRes. FNS
 Physicochemical and Functional Properties of Dehydrated Japanese Quail (Coturnix japonica) Egg White 293 Table 2. Denaturation temperatures (Td) and enthalpies (ΔH) of quail egg white dried by air convection (M1, 65˚C and 3.5 h; M2, 65˚C and 5 h; M3, 70˚C and 3.5 h; M4, 70˚C and 5 h), and lyophilization (ML). Treatment Td (˚C) ΔH (J/g) M1 83.43 ± 0.0a 7.79 ± 0.02b M2 83.85 ± 0.32a 7.39 ± 0.24b M3 83.61 ± 0.24a 5.51 ± 0.17c M4 82.86 ± 0.18b 5.59 ± 0.05c ML 81.16 ± 0.01b 9.08 ± 0.03a to the 84.5˚C reported by Raeker and Johnson [20] for chicken egg ovalbumin suggesting that the denaturation temperatures observed here corresponded to ovalbumin. During storage, ovalbumin is altered to s-ovalbumin, a much more thermo-stable form (denaturation at 92.5˚C) than ovalbumin (denaturation at 84˚C) [6]. S-ovalbumin has a slightly lighter molecular weight than ovalbumin and its relative proportion in the egg white can increase from 5% in fresh egg to 81% after six months of refrig- erated storage. The present results suggest that ovalbu- min had not converted to s-ovalbumin in the studied treatments. Denaturation temperature was slightly lower in the ML treatment than in the M1-4 treatments, possi- bly due to conformational changes caused by drying method [21]. Protein denaturation involves alteration of a protein’s native form through significant changes in its three-di- mensional (3D) conformation produced by movements of different protein domains, a phenomenon which increases molecular entropy. These changes cause a loss in secon- dary, tertiary and quaternary structure, generating greater interaction between hydrophobic residues and resulting in aggregation of deployed proteins. Heat is one of the most frequently used denaturing methods, and affects stability in the non-covalent interactions of a protein’s 3D structure because it lowers molecule enthalpy and breaks the bonds which maintain balance within the structure. In the present study, denaturation enthalpy ranged from 5.51 J/g in M3 to 9.08 J/g in ML, meaning the former requires less energy to denature than the latter. Air drying at the studied temperatures may therefore have partially denatured the protein in the quail egg white, whereas drying by lyophilization probably did not [22]. Air-dried quail egg white is therefore a promising ingredient in baked products such as breads and cakes because its denaturation temperature is similar to that of starch gelatinization (85˚C - 90˚C). Incorporation of this ingredient into baked products would allow an increase in shake viscosity and attainment of maximum volume and texture before and during the baking. 3.4. Functional Characterization 3.4.1. Water-Holding (WHC) and Oil-Holding Capacity (OHC) Water-holding capacity was higher in ML (4.5 g/g) than in M1-4 (0.90 to 2.95 g/g). Similar values have been re- ported for chicken egg white: untreated white (1.30 g/g) [22], lyophilized white (2.58 g/g) [23]. Differences in WHC between the ML and M1-4 treatments can proba- bly be attributed to heat-induced changes in conforma- tion or partial denaturation. Denaturation led to increased dispersion velocity as the protein molecule unfolded and consequently expanded molecule dimensions. The air- dried treatments may have had primarily attractive forces which would force water out of the network matrix and lower WHC. External factors (e.g., stirring velocity, pH, and protein concentration) which can be manipulated during recovery or measurement may also have influ- enced this property. Food processing and development of new food products require ingredients such as gelling agents which can build up a structural matrix and provide a desirable texture. The dehydrated quail egg white stud- ied here is a promising alternative ingredient in products such as desserts, puddings and reformulated meat prod- ucts. Oil-holding capacity was also higher in the ML (1.95 g/g) than in the M1-4 treatments (0.92 to 1.01 g/g). The higher OHC in the ML treatment was probably due to a higher nonpolar residue content, which would have pro- vided more contact surface and therefore increased OHC. The OHC values from the M1-4 treatments were lower than reported for lyophilized chicken egg white (4.22 g/g) [23]. Based on its OHC values, the ML treatment would be useful in improving structural interactions in food such as flavor retention and improvement of palatability, while the M1-4 treatments would be useful as ingredients in fried products since they would provide a non-greasy sensation. 3.4.2. Foaming Capacity and Foam Stability The ability of the quail egg white proteins to form and stabilize foams was related to their amphiphilic behavior (polar/nonpolar). Foams consist of an aqueous continu- ous phase and a gaseous disperse phase [24]. Textural properties in foams depend on dispersion of numerous air bubbles and formation of a thin film (lamella) in the liq- uid-gas interphase. The proteins in the dehydrated quail egg white effectively formed a gaseous disperse phase with each droplet enveloped by a thin, continuous film of protein molecules and each bubble separated by a dense lamella. Foaming capacity in the dehydrated quail egg white was pH-dependent (Figure 2): alkaline pH resulted in low values, and acid pH (pH 2) produced high values. The higher foaming capacity observed at acid pH was Copyright © 2013 SciRes. FNS
 Physicochemical and Functional Properties of Dehydrated Japanese Quail (Coturnix japonica) Egg White 294 Figure 2. Effect of pH on the foaming capacity (%) in de- hydrated quail egg white: Air convection-dried treatments M1 (65˚C, 3.5 h), M2 (65˚C, 5 h), M3 (70˚C, 3.5 h) and M4 (70˚C, 5 h); Lyophilized treatment, ML. probably due to an increase in proteinnet charge. This weakens hydrophobic interactions and increases protein flexibility, allowing them to more rapidly spread the air-water interface, encapsulate air particles and increase foam formation. At acid pH (2), the M1 treatment exhib- ited a higher (p < 0.05) foaming capacity (222%) than M2-4 (153% - 198%) and ML (168%). In M1, this be- havior could be explained by a loss of secondary and tertiary structure due to the air-drying treatment (65˚C, 3.5 h), which would increase molecule surfactant power. This suggests that a certain sequence of reactions oc- curred during foam formation with the quail egg white proteins. Energy input would have triggered the process, causing soluble proteins to reach the air-water interface by diffusion, adsorption, concentration and critical sur- face tension. Polypeptide rearrangement in the interface was oriented by polar mobility, with polar segments drawn to water and non-polar segments drawn to air par- ticles. During interfacial movement, the partially-opened proteins would have formed new intermolecular associa- tions with neighboring molecules, generating the cohe- sive and continuous films essential to foam formation. The main attractive forces in this process may have been hydrogen bonds, hydrophobic interactions, and electro- static and van der Waals forces. At alkaline pH values, foaming capacity in the dehydrated quail egg white treat- ments was similar to the 60% reported for dehydrated chicken egg white at pH 10 and a 2% concentration [16]. Superficial activity in the quail egg white proteins de- pended on both the hydrophobic/hydrophilic relationship and protein conformation. The high foaming capacity in the M1 treatment was therefore a product of the polypep- tide chain stability/flexibility relationship, its adaptability to environmental changes and the distribution patterns of hydrophilic and hydrophobic groups on the protein sur- face. Foam stability was lowest in the M1-4 treatments at pH 2, with losses of 137 (M1), 116 (M2), 103 (M3) and 91% (M4) from 30 seconds to 120 min (Figure 3). These values reflect the rapid loss of the proteins’ capacity to stabilize foam against gravitational and mechanical stress. The foams steadily lost volume after rapid adsorption of the proteins into the interphase, which depended on dis- tribution of hydrophobic and hydrophilic zones on the surface. This loss of volume may have occurred because the protein film formed around each gas bubble was not sufficiently viscous, elastic and resistant to produce gas permeability and inhibit foam coalescence. Therefore, at pH 2 the dehydrated quail egg white in all the treatments was unable to support the weight of the very high foam volume produced. During the same time period (30 sec to 120 min), foam stability was highest in M1 (17%) at pH 10, in M2 (11%) at pH 8, in M3 (12%) at pH 4 and in M4 (10%) at pH 6. The structural components and attractive electrostatic interactions (forces) present under these Figure 3. Effect of pH on foam stability (%) in air convec- tion-dried quail egg white: M1 (65˚C, 3.5 h); M2 (65˚C, 5 h); M3 (70˚C, 3.5 h); M4 (70˚C, 5 h). Copyright © 2013 SciRes. FNS
 Physicochemical and Functional Properties of Dehydrated Japanese Quail (Coturnix japonica) Egg White 295 conditions enabled the formation of intermolecular asso- ciations and improved foaming properties. Non-polar residues intensively contribute to interactive forces at the hydrophobic interface, increasing the surface activity within which hydrophilic residues associated to decrease surface activity, thus improving film quality and foam stability. Foam stability depended on the ability of pro- tein surface activity to improve film elasticity, and was reflected in the balance between forces inside the film and the forces among adjacent bubbles. The high foam stability at alkaline pH values may also be an artifact of the lower foam volume at these pH values, which led to lower bubble coalescence. The proteins which stabilize foams are more stable at their isoelectric point than at other pH levels. For in- stance, chicken egg white has good foaming properties at pH 8 - 9, but at or near its isoelectric pH (4 - 5) the re- duced presence of repulsion interactions promotes pro- tein-protein interactions and the formation of a viscous film in the interphase which favors foaming capacity and foam stability. In a study of dehydrated chicken egg white, Vani and Zayas [16] reported foam stability values of 52 (30 min), 46.6 (60 min), 42 (90 min) and 37.6% (120 min) at pH 10 and a 2% concentration. Foam stabil- ity at 30 min increased at protein concentrations of 8% due to a lower liquid flow inside the lamella. Effectively forming a protein capsule that holds air bubbles also re- quires non-covalent interactions, such as electrostatic and hydrophobic forces, hydrogen bounds, and disulphide linkages. A balance between non-covalent interactions may help to form a cohesive, viscous film, which is re- quired for foam stability. The lower foam stability values observed in the present study were possibly due to elec- trostatic repulsions. Disulphide linkages reduce protein flexibility, and the change of a thiol group to a disulphide has significant repercussions on functional properties. For example, molecular alterations induced by reduction of disulphide linkages between protein molecules im- prove film formation in foam and contribute to its stabi- lization [25]. In another study, Doi et al. [26] found that the essential factor in the formation of stable foam with ovalbumin was not disulphide linkage formation, but network formation by other non-covalent interactions. Disulphide bindingis critical to stabilizing protein struc- ture, constraining molecular unfolding and preventing total exposition of hydrophobic regions, meaning forma- tion of disulphide linkages at the air-water interface may improve foam stability [27]. The proteins in dehydrated egg white encapsulate and retain air, making them useful in improving desirable textural attributes in products such as bread, cookies, meringues, ice creams, and other bak- ery products during and after processing. However, foam- ing properties also depend on protein concentration, film thickness, ionic strength, pH, temperature, the presence of other components in food systems, and protein phys- icochemical properties. 3.4.3. Emulsifying Activity (EA) and Emulsion Stability (ES) Proteins are excellent surfactants in the formulation of food emulsions (oil-water) because their surface is active and favors resistance to coalescence. In the air-dried quail egg white treatments, EA was highest overall at pH 8 (41% - 46%), values only slightly lower than that of chicken egg white (53%) [28]. Overall EA ranges dif- fered (p < 0.05) between treatments across the studied pH levels (2 - 10): M1 = 3% to 40%; M2 = 4% to 42%; M3 = 3% to 43%; M4 = 2% to 45%; and ML = 5% to 15% (Figure 4). At pH levels from 2 to 6, M1-4 exhib- ited EA values between 8 and 23%. At pH 8 they reached peak EA and then decreased drastically to the lowest levels at pH 10. The ML treatment exhibited EA values (5% - 18%) lower than those of the air-dried treatments at all pH levels. Conformational stability was a deter- mining factor in the EA behavior of the dehydrated quail egg white as shown by their ΔH values. Greater instabil- ity and emulsifying activity are associated with low ΔH values, and the relatively lower values for the M1-4 treatments compared to the ML treatment indicate that the conditions in the air-dried treatments allowed the protein to unfold within the oil-water interface, thus re- ducing interfacial tension and aiding in emulsion forma- tion. According to Chau et al. [29], the viscosity gener- ated by variation in pH allows formation of a rigid and elastic protein film appropriate for reducing interfacial tension and producing high EA values at pH 8. Emulsion stability was pH-dependent in the dehy- drated quail egg white (Figure 5). Maximum values were observed at pH 2 and 4 in M1 (91.81% and 88.98%, re- spectively), M3 (97.50% and 92.16%, respectively) and M4 (96.16% and 95.74%, respectively). The amphiphilic Figure 4. Effect of pH on emulsifying activity (%) in dehy- drated quail egg white: Air convection-dried treatments M1 (65˚C, 3.5 h), M2 (65˚C, 5 h), M3 (70˚C, 3.5 h) and M4 (70˚C, 5 h); Lyophilized treatment, ML. Copyright © 2013 SciRes. FNS
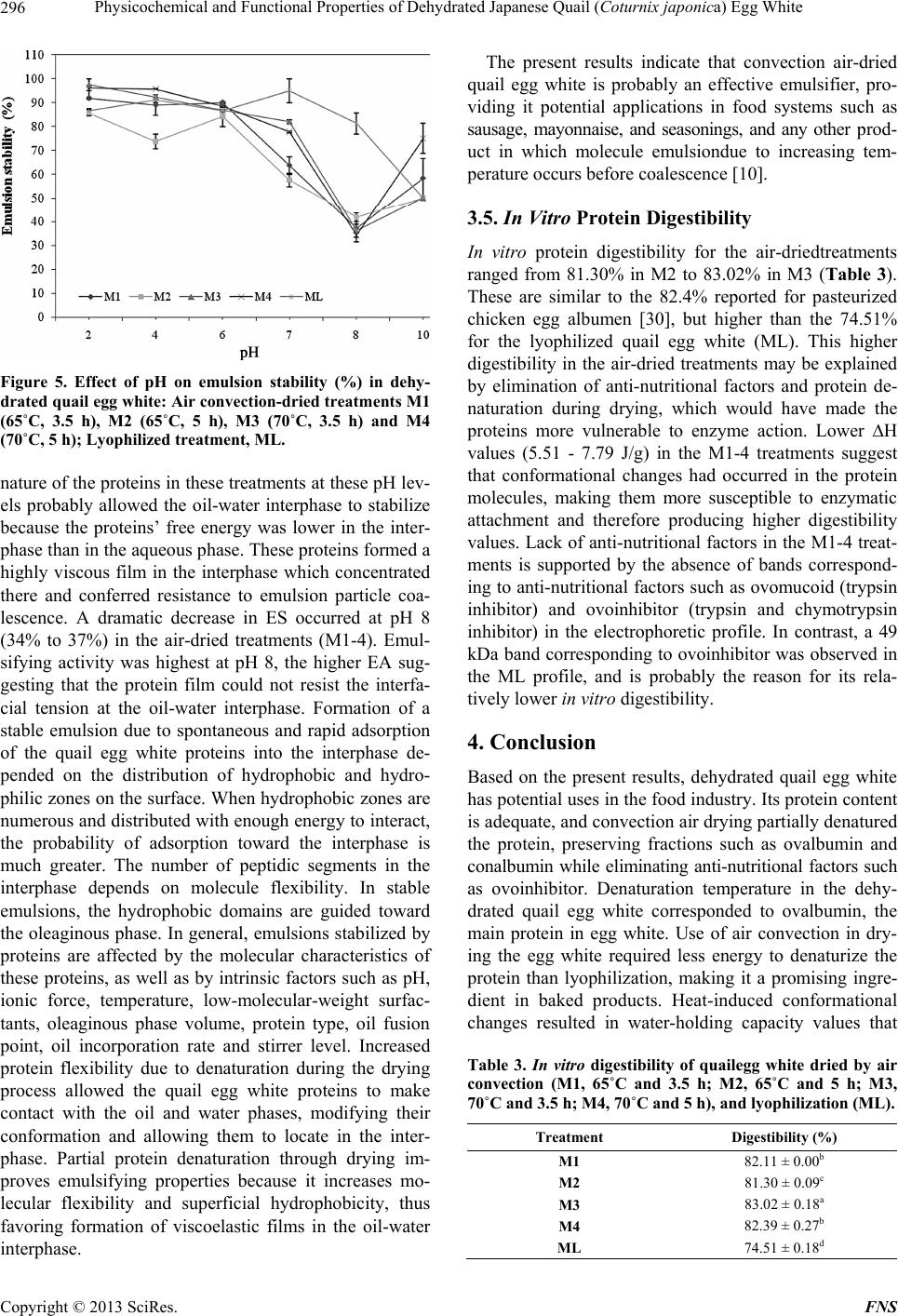 Physicochemical and Functional Properties of Dehydrated Japanese Quail (Coturnix japonica) Egg White 296 Figure 5. Effect of pH on emulsion stability (%) in dehy- drated quail egg white: Air convection-dried treatments M1 (65˚C, 3.5 h), M2 (65˚C, 5 h), M3 (70˚C, 3.5 h) and M4 (70˚C, 5 h); Lyophilized treatment, ML. nature of the proteins in these treatments at these pH lev- els probably allowed the oil-water interphase to stabilize because the proteins’ free energy was lower in the inter- phase than in the aqueous phase. These proteins formed a highly viscous film in the interphase which concentrated there and conferred resistance to emulsion particle coa- lescence. A dramatic decrease in ES occurred at pH 8 (34% to 37%) in the air-dried treatments (M1-4). Emul- sifying activity was highest at pH 8, the higher EA sug- gesting that the protein film could not resist the interfa- cial tension at the oil-water interphase. Formation of a stable emulsion due to spontaneous and rapid adsorption of the quail egg white proteins into the interphase de- pended on the distribution of hydrophobic and hydro- philic zones on the surface. When hydrophobic zones are numerous and distributed with enough energy to interact, the probability of adsorption toward the interphase is much greater. The number of peptidic segments in the interphase depends on molecule flexibility. In stable emulsions, the hydrophobic domains are guided toward the oleaginous phase. In general, emulsions stabilized by proteins are affected by the molecular characteristics of these proteins, as well as by intrinsic factors such as pH, ionic force, temperature, low-molecular-weight surfac- tants, oleaginous phase volume, protein type, oil fusion point, oil incorporation rate and stirrer level. Increased protein flexibility due to denaturation during the drying process allowed the quail egg white proteins to make contact with the oil and water phases, modifying their conformation and allowing them to locate in the inter- phase. Partial protein denaturation through drying im- proves emulsifying properties because it increases mo- lecular flexibility and superficial hydrophobicity, thus favoring formation of viscoelastic films in the oil-water interphase. The present results indicate that convection air-dried quail egg white is probably an effective emulsifier, pro- viding it potential applications in food systems such as sausage, mayonnaise, and seasonings, and any other prod- uct in which molecule emulsiondue to increasing tem- perature occurs before coalescence [10]. 3.5. In Vitro Protein Digestibility In vitro protein digestibility for the air-driedtreatments ranged from 81.30% in M2 to 83.02% in M3 (Table 3). These are similar to the 82.4% reported for pasteurized chicken egg albumen [30], but higher than the 74.51% for the lyophilized quail egg white (ML). This higher digestibility in the air-dried treatments may be explained by elimination of anti-nutritional factors and protein de- naturation during drying, which would have made the proteins more vulnerable to enzyme action. Lower ∆H values (5.51 - 7.79 J/g) in the M1-4 treatments suggest that conformational changes had occurred in the protein molecules, making them more susceptible to enzymatic attachment and therefore producing higher digestibility values. Lack of anti-nutritional factors in the M1-4 treat- ments is supported by the absence of bands correspond- ing to anti-nutritional factors such as ovomucoid (trypsin inhibitor) and ovoinhibitor (trypsin and chymotrypsin inhibitor) in the electrophoretic profile. In contrast, a 49 kDa band corresponding to ovoinhibitor was observed in the ML profile, and is probably the reason for its rela- tively lower in vitro digestibility. 4. Conclusion Based on the present results, dehydrated quail egg white has potential uses in the food industry. Its protein content is adequate, and convection air drying partially denatured the protein, preserving fractions such as ovalbumin and conalbumin while eliminating anti-nutritional factors such as ovoinhibitor. Denaturation temperature in the dehy- drated quail egg white corresponded to ovalbumin, the main protein in egg white. Use of air convection in dry- ing the egg white required less energy to denaturize the protein than lyophilization, making it a promising ingre- dient in baked products. Heat-induced conformational changes resulted in water-holding capacity values that Table 3. In vitro digestibility of quailegg white dried by air convection (M1, 65˚C and 3.5 h; M2, 65˚C and 5 h; M3, 70˚C and 3.5 h; M4, 70˚C and 5 h), and lyophilization (ML). Treatment Digestibility (%) M1 82.11 ± 0.00b M2 81.30 ± 0.09c M3 83.02 ± 0.18a M4 82.39 ± 0.27b ML 74.51 ± 0.18d Copyright © 2013 SciRes. FNS
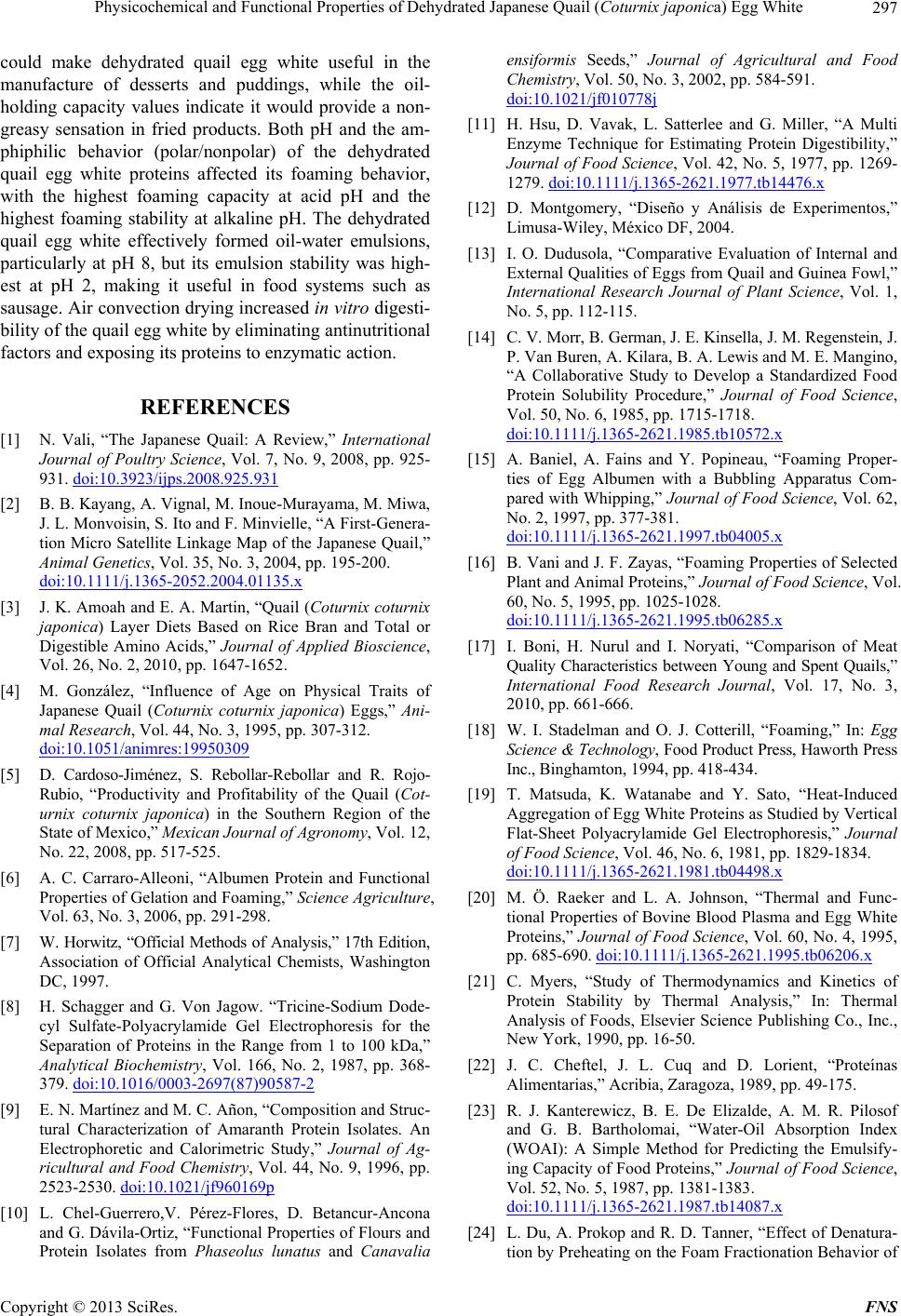 Physicochemical and Functional Properties of Dehydrated Japanese Quail (Coturnix japonica) Egg White 297 could make dehydrated quail egg white useful in the manufacture of desserts and puddings, while the oil- holding capacity values indicate it would provide a non- greasy sensation in fried products. Both pH and the am- phiphilic behavior (polar/nonpolar) of the dehydrated quail egg white proteins affected its foaming behavior, with the highest foaming capacity at acid pH and the highest foaming stability at alkaline pH. The dehydrated quail egg white effectively formed oil-water emulsions, particularly at pH 8, but its emulsion stability was high- est at pH 2, making it useful in food systems such as sausage. Air convection drying increased in vitro digesti- bility of the quail egg white by eliminating antinutritional factors and exposing its proteins to enzymatic action. REFERENCES [1] N. Vali, “The Japanese Quail: A Review,” International Journal of Poultry Science, Vol. 7, No. 9, 2008, pp. 925- 931. doi:10.3923/ijps.2008.925.931 [2] B. B. Kayang, A. Vignal, M. Inoue-Murayama, M. Miwa, J. L. Monvoisin, S. Ito and F. Minvielle, “A First-Genera- tion Micro Satellite Linkage Map of the Japanese Quail,” Animal Genetics, Vol. 35, No. 3, 2004, pp. 195-200. doi:10.1111/j.1365-2052.2004.01135.x [3] J. K. Amoah and E. A. Martin, “Quail (Coturnix coturnix japonica) Layer Diets Based on Rice Bran and Total or Digestible Amino Acids,” Journal of Applied Bioscience, Vol. 26, No. 2, 2010, pp. 1647-1652. [4] M. González, “Influence of Age on Physical Traits of Japanese Quail (Coturnix coturnix japonica) Eggs,” Ani- mal Research, Vol. 44, No. 3, 1995, pp. 307-312. doi:10.1051/animres:19950309 [5] D. Cardoso-Jiménez, S. Rebollar-Rebollar and R. Rojo- Rubio, “Productivity and Profitability of the Quail (Cot- urnix coturnix japonica) in the Southern Region of the State of Mexico,” Mexican Journal of Agronomy, Vol. 12, No. 22, 2008, pp. 517-525. [6] A. C. Carraro-Alleoni, “Albumen Protein and Functional Properties of Gelation and Foaming,” Science Agriculture, Vol. 63, No. 3, 2006, pp. 291-298. [7] W. Horwitz, “Official Methods of Analysis,” 17th Edition, Association of Official Analytical Chemists, Washington DC, 1997. [8] H. Schagger and G. Von Jagow. “Tricine-Sodium Dode- cyl Sulfate-Polyacrylamide Gel Electrophoresis for the Separation of Proteins in the Range from 1 to 100 kDa,” Analytical Biochemistry, Vol. 166, No. 2, 1987, pp. 368- 379. doi:10.1016/0003-2697(87)90587-2 [9] E. N. Martínez and M. C. Añon, “Composition and Struc- tural Characterization of Amaranth Protein Isolates. An Electrophoretic and Calorimetric Study,” Journal of Ag- ricultural and Food Chemistry, Vol. 44, No. 9, 1996, pp. 2523-2530. doi:10.1021/jf960169p [10] L. Chel-Guerrero,V. Pérez-Flores, D. Betancur-Ancona and G. Dávila-Ortiz, “Functional Properties of Flours and Protein Isolates from Phaseolus lunatus and Canavalia ensiformis Seeds,” Journal of Agricultural and Food Chemistry, Vol. 50, No. 3, 2002, pp. 584-591. doi:10.1021/jf010778j [11] H. Hsu, D. Vavak, L. Satterlee and G. Miller, “A Multi Enzyme Technique for Estimating Protein Digestibility,” Journal of Food Science, Vol. 42, No. 5, 1977, pp. 1269- 1279. doi:10.1111/j.1365-2621.1977.tb14476.x [12] D. Montgomery, “Diseño y Análisis de Experimentos,” Limusa-Wiley, México DF, 2004. [13] I. O. Dudusola, “Comparative Evaluation of Internal and External Qualities of Eggs from Quail and Guinea Fowl,” International Research Journal of Plant Science, Vol. 1, No. 5, pp. 112-115. [14] C. V. Morr, B. German, J. E. Kinsella, J. M. Regenstein, J. P. Van Buren, A. Kilara, B. A. Lewis and M. E. Mangino, “A Collaborative Study to Develop a Standardized Food Protein Solubility Procedure,” Journal of Food Science, Vol. 50, No. 6, 1985, pp. 1715-1718. doi:10.1111/j.1365-2621.1985.tb10572.x [15] A. Baniel, A. Fains and Y. Popineau, “Foaming Proper- ties of Egg Albumen with a Bubbling Apparatus Com- pared with Whipping,” Journal of Food Science, Vol. 62, No. 2, 1997, pp. 377-381. doi:10.1111/j.1365-2621.1997.tb04005.x [16] B. Vani and J. F. Zayas, “Foaming Properties of Selected Plant and Animal Proteins,” Journal of Food Science, Vol. 60, No. 5, 1995, pp. 1025-1028. doi:10.1111/j.1365-2621.1995.tb06285.x [17] I. Boni, H. Nurul and I. Noryati, “Comparison of Meat Quality Characteristics between Young and Spent Quails,” International Food Research Journal, Vol. 17, No. 3, 2010, pp. 661-666. [18] W. I. Stadelman and O. J. Cotterill, “Foaming,” In: Egg Science & Technology, Food Product Press, Haworth Press Inc., Binghamton, 1994, pp. 418-434. [19] T. Matsuda, K. Watanabe and Y. Sato, “Heat-Induced Aggregation of Egg White Proteins as Studied by Vertical Flat-Sheet Polyacrylamide Gel Electrophoresis,” Journal of Food Science, Vol. 46, No. 6, 1981, pp. 1829-1834. doi:10.1111/j.1365-2621.1981.tb04498.x [20] M. Ö. Raeker and L. A. Johnson, “Thermal and Func- tional Properties of Bovine Blood Plasma and Egg White Proteins,” Journal of Food Science, Vol. 60, No. 4, 1995, pp. 685-690. doi:10.1111/j.1365-2621.1995.tb06206.x [21] C. Myers, “Study of Thermodynamics and Kinetics of Protein Stability by Thermal Analysis,” In: Thermal Analysis of Foods, Elsevier Science Publishing Co., Inc., New York, 1990, pp. 16-50. [22] J. C. Cheftel, J. L. Cuq and D. Lorient, “Proteínas Alimentarias,” Acribia, Zaragoza, 1989, pp. 49-175. [23] R. J. Kanterewicz, B. E. De Elizalde, A. M. R. Pilosof and G. B. Bartholomai, “Water-Oil Absorption Index (WOAI): A Simple Method for Predicting the Emulsify- ing Capacity of Food Proteins,” Journal of Food Science, Vol. 52, No. 5, 1987, pp. 1381-1383. doi:10.1111/j.1365-2621.1987.tb14087.x [24] L. Du, A. Prokop and R. D. Tanner, “Effect of Denatura- tion by Preheating on the Foam Fractionation Behavior of Copyright © 2013 SciRes. FNS
 Physicochemical and Functional Properties of Dehydrated Japanese Quail (Coturnix japonica) Egg White Copyright © 2013 SciRes. FNS 298 Ovalbumin,” Journal of Colloid and Interface Science, Vol. 248, No. 2, 2002, pp. 487-492. doi:10.1006/jcis.2001.8163 [25] J. B. German and L. Phillips, “Protein Interactions in Foams: Protein-Gas Phase Interactions,” In: Protein Func- tionality in Food Systems, Marcel Dekker, Inc., New York, 1994, pp. 181-208. [26] E. Doi and N. Kitabatake, “Structure and Functionality of Egg Proteins,” In: Food Proteins and Their Applications, Marcel Dekker, Inc., New York, 1997, pp. 325-340. [27] E. Li-Chan and S. Nakai, “Raman Spectroscopy Study of Thermally and/or Dithiothreitol Induced Gelation of Li- sozyme,” Journal of Agricultural and Food Chemistry, Vol. 39, No. 7, 1991, pp. 1238-1245. doi:10.1021/jf00007a009 [28] M. Ahmenda, W. Prinyawiwatkul and R. Rao, “Solubi- lized Wheat Protein Isolate: Functional Properties and Potential Food Applications,” Journal of Agricultural and Food Chemistry, Vol. 47, No. 4, 1999, pp. 1340-1345. doi:10.1021/jf981098s [29] C. F. Chau, K. Cheung and Y. S. Wong, “Functional Properties of Protein Concentrates from Three Chinese Indigenous Legume Seeds,” Journal of Agricultural and Food Chemistry, Vol. 45, No. 11, 1997, pp. 2500-2503. doi:10.1021/jf970047c [30] C. R. Hank, M. E. Kunkel, P. L. Dawson, J. C. Acton and F. B. Wardlaw, “The Effect of the Shell Egg Pasteurization on the Protein Quality of Albumen,” Poultry Science, Vol. 80, No. 6, 2001, pp. 821-824.
|