 Journal of Behavioral and Brain Science, 2013, 3, 26-48 http://dx.doi.org/10.4236/jbbs.2013.31004 Published Online February 2013 (http://www.scirp.org/journal/jbbs) Copyright © 2013 SciRes. JBBS Heart Rate Variability, Standard of Measurement, Physiological Interpretation and Clinical Use in Mountain Marathon Runners during Sleep and after Acclimatization at 3480 m Ivana Gritti1*, Stefano Defendi1, Clara Mauri1, Giuseppe Banfi1, Piergiorgio Duca1, Giulio Sergio Roi2 1Department of Biomedical and Clinical Science Luigi Sacco, University of Milan, Milan, Italy 2Education and Research Department Isokinetic, Bologna, Italy Email: *Ivana.Gritti@unimi.it, Piergiorgio.Duca@unimi.it, gs.roi@isokinetic.com Received November 19, 2012; revised December 20, 2012; accepted December 27, 2012 ABSTRACT Fluctuations in autonomic cardiovascular regulation during exposure to high altitude may increase the risk of heart at- tack during waking and sleep. This study compared heart rate variability (HVR) and its components during sleep at low altitude and after 30 - 41 hours of acclimatization at high altitude (3480 m) in five mountain marathon runners con- trolled for diet, drugs, light-dark cycle and jet lag. In comparison to sea level, RR-intervals during sleep at high altitude decreased significantly (P < 0.001). The significant increase in sympathetic autonomic cardiovascular modulation at high altitude protects against excessive oxygen deprivation during sleep. Increases in R-R intervals can require longer periods of acclimatization at 3480 m to mitigate the effects of altitude/hypoxia on sympathetic tone, thus reducing car- diovascular distress at rest during waking and sleep and probably before during and after athletic performance at alti- tude. Keywords: Heart Rate Variability; Very Low Frequency Fluctuation (VLF, <0.04 Hz) Rhythm; Low-Frequency (LF, 0.04 - 0.15 Hz) Rhythm; High-Frequency (HF, 0.15 - 0.4 Hz) Rhythm; Mountain Marathon Runners 1. Introduction 1.1. Heart Rate Variability Intrinsic to pacemaker tissues, cardiac automaticity is re- gulated by the central nervous systems (CNS). Control of the cardiac cycle is also mediated by local and autonomic nervous system components: the parasympathetic influ- ence on heart rate is modulated by acetylcholine released by the vagus nerve on the sinoatrial node and the sympa- thetic influence by the release of epinephrine and nore- pinephrine. Under resting conditions, vagal modulation and tone predominate at the level of the sinoatrial node. Vagal and sympathetic activities interact constantly [1 hereinafter Task Force 1996]. In the sympathovagal and thoracic systems, CNS con- trol and influences on the autonomic mechanism can be physiologically and voluntarily cut off to different de- grees during relaxed attentive waking and involuntarily during the progressive deepening of slow-wave sleep [1]. Alterations in the autonomic nervous system may give rise to cardiovascular and/or cerebrovascular diseases and have been frequently associated with death in hu- mans. Research into predisposition to arrhythmias and increased sympathetic activity or reduced vagal activity has led to the development of quantitative markers of autonomic activity [1]. The Task Force [1] has suggested that cardiovascular changes can be investigated non-invasively by electro- cardiography (ECG) and by common parameters derived from ECG such as heart rate variability (HRV), i.e., the variation in the duration of two consecutive R-R intervals. R-R interval variations during resting condition are pre- cisely tuned by reflexes directed to the sinus node and modulated by central (vasomotor and respiratory centre) and peripheral (arterial pressure and respiratory move- ments) oscillators, particularly during high altitude ex- posure. Analysis of R-R intervals provides information about the state and function of central oscillators, sympa- thetic and vagal efferent activities, humoral cardiac fac- tors, and sinus node characteristics [1]. *Corresponding author.
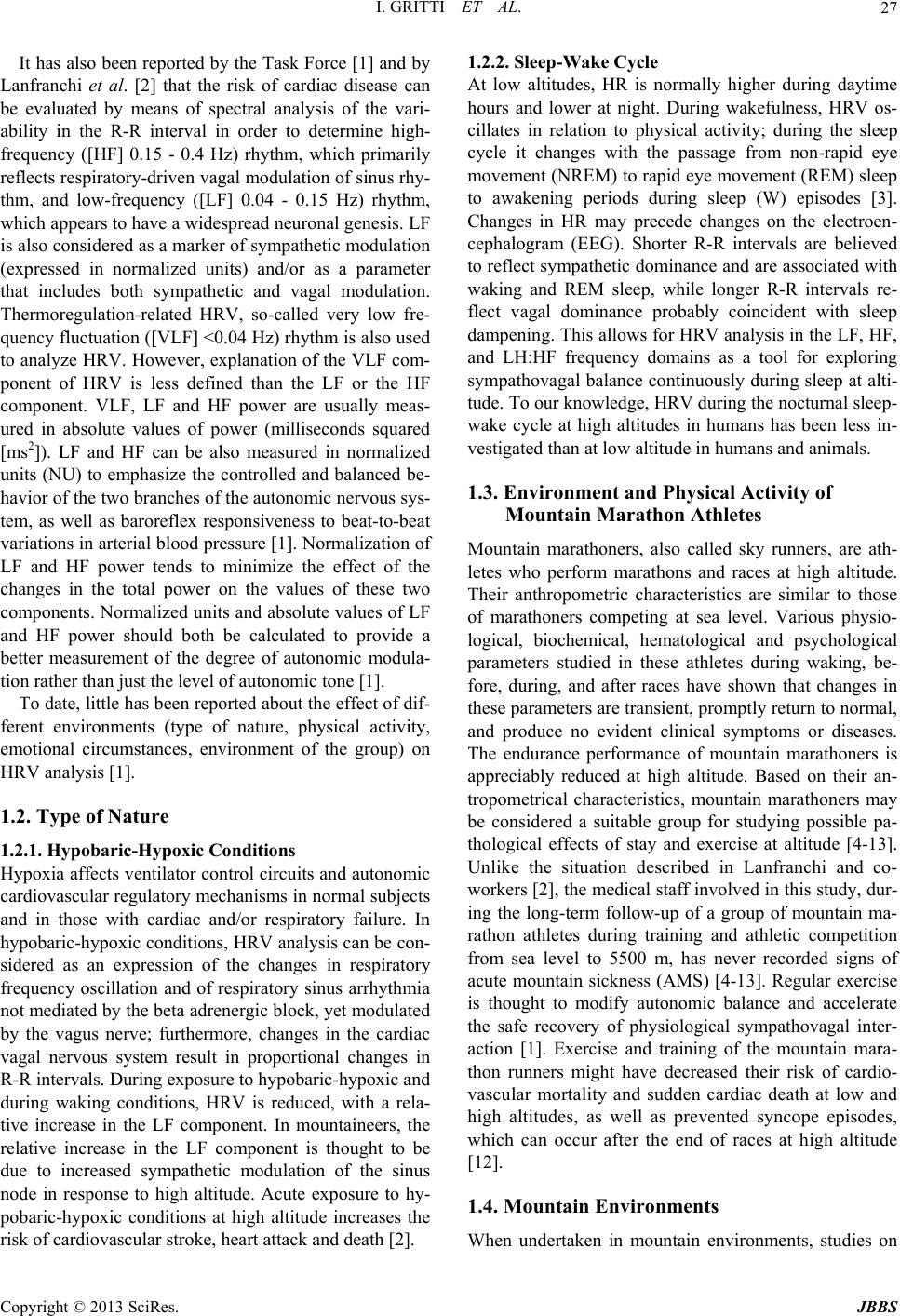 I. GRITTI ET AL. Copyright © 2013 SciRes. JBBS 27 It has also been reported by the Task Force [1] and by Lanfranchi et al. [2] that the risk of cardiac disease can be evaluated by means of spectral analysis of the vari- ability in the R-R interval in order to determine high- frequency ([HF] 0.15 - 0.4 Hz) rhythm, which primarily reflects respiratory-driven vagal modulation of sinus rhy- thm, and low-frequency ([LF] 0.04 - 0.15 Hz) rhythm, which appears to have a widespread neuronal genesis. LF is also considered as a marker of sympathetic modulation (expressed in normalized units) and/or as a parameter that includes both sympathetic and vagal modulation. Thermoregulation-related HRV, so-called very low fre- quency fluctuation ([VLF] <0.04 Hz) rhythm is also used to analyze HRV. However, explanation of the VLF com- ponent of HRV is less defined than the LF or the HF component. VLF, LF and HF power are usually meas- ured in absolute values of power (milliseconds squared [ms2]). LF and HF can be also measured in normalized units (NU) to emphasize the controlled and balanced be- havior of the two branches of the autonomic nervous sys- tem, as well as baroreflex responsiveness to beat-to-beat variations in arterial blood pressure [1]. Normalization of LF and HF power tends to minimize the effect of the changes in the total power on the values of these two components. Normalized units and absolute values of LF and HF power should both be calculated to provide a better measurement of the degree of autonomic modula- tion rather than just the level of autonomic tone [1]. To date, little has been reported about the effect of dif- ferent environments (type of nature, physical activity, emotional circumstances, environment of the group) on HRV analysis [1]. 1.2. Type of Nature 1.2.1. Hypobaric-Hypoxic Conditions Hypoxia affects ventilator control circuits and autonomic cardiovascular regulatory mechanisms in normal subjects and in those with cardiac and/or respiratory failure. In hypobaric-hypoxic conditions, HRV analysis can be con- sidered as an expression of the changes in respiratory frequency oscillation and of respiratory sinus arrhythmia not mediated by the beta adrenergic block, yet modulated by the vagus nerve; furthermore, changes in the cardiac vagal nervous system result in proportional changes in R-R intervals. During exposure to hypobaric-hypoxic and during waking conditions, HRV is reduced, with a rela- tive increase in the LF component. In mountaineers, the relative increase in the LF component is thought to be due to increased sympathetic modulation of the sinus node in response to high altitude. Acute exposure to hy- pobaric-hypoxic conditions at high altitude increases the risk of cardiovascular stroke, heart attack and death [2]. 1.2.2. Sleep-Wake Cycle At low altitudes, HR is normally higher during daytime hours and lower at night. During wakefulness, HRV os- cillates in relation to physical activity; during the sleep cycle it changes with the passage from non-rapid eye movement (NREM) to rapid eye movement (REM) sleep to awakening periods during sleep (W) episodes [3]. Changes in HR may precede changes on the electroen- cephalogram (EEG). Shorter R-R intervals are believed to reflect sympathetic dominance and are associated with waking and REM sleep, while longer R-R intervals re- flect vagal dominance probably coincident with sleep dampening. This allows for HRV analysis in the LF, HF, and LH:HF frequency domains as a tool for exploring sympathovagal balance continuously during sleep at alti- tude. To our knowledge, HRV during the nocturnal sleep- wake cycle at high altitudes in humans has been less in- vestigated than at low altitude in humans and animals. 1.3. Environment and Physical Activity of Mountain Marathon Athletes Mountain marathoners, also called sky runners, are ath- letes who perform marathons and races at high altitude. Their anthropometric characteristics are similar to those of marathoners competing at sea level. Various physio- logical, biochemical, hematological and psychological parameters studied in these athletes during waking, be- fore, during, and after races have shown that changes in these parameters are transient, promptly return to normal, and produce no evident clinical symptoms or diseases. The endurance performance of mountain marathoners is appreciably reduced at high altitude. Based on their an- tropometrical characteristics, mountain marathoners may be considered a suitable group for studying possible pa- thological effects of stay and exercise at altitude [4-13]. Unlike the situation described in Lanfranchi and co- workers [2], the medical staff involved in this study, dur- ing the long-term follow-up of a group of mountain ma- rathon athletes during training and athletic competition from sea level to 5500 m, has never recorded signs of acute mountain sickness (AMS) [4-13]. Regular exercise is thought to modify autonomic balance and accelerate the safe recovery of physiological sympathovagal inter- action [1]. Exercise and training of the mountain mara- thon runners might have decreased their risk of cardio- vascular mortality and sudden cardiac death at low and high altitudes, as well as prevented syncope episodes, which can occur after the end of races at high altitude [12]. 1.4. Mountain Environments When undertaken in mountain environments, studies on
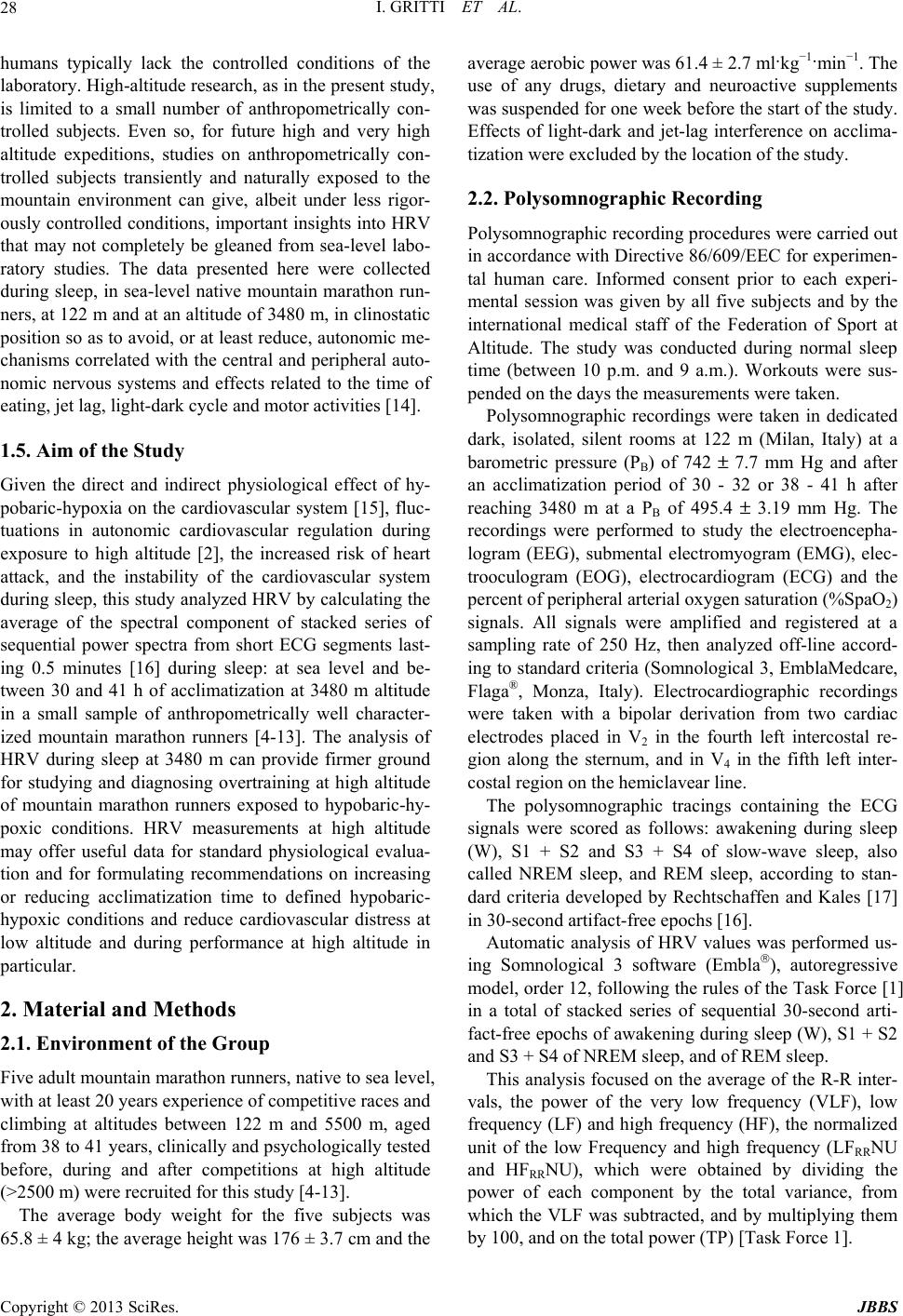 I. GRITTI ET AL. Copyright © 2013 SciRes. JBBS 28 humans typically lack the controlled conditions of the laboratory. High-altitude research, as in the present study, is limited to a small number of anthropometrically con- trolled subjects. Even so, for future high and very high altitude expeditions, studies on anthropometrically con- trolled subjects transiently and naturally exposed to the mountain environment can give, albeit under less rigor- ously controlled conditions, important insights into HRV that may not completely be gleaned from sea-level labo- ratory studies. The data presented here were collected during sleep, in sea-level native mountain marathon run- ners, at 122 m and at an altitude of 3480 m, in clinostatic position so as to avoid, or at least reduce, autonomic me- chanisms correlated with the central and peripheral auto- nomic nervous systems and effects related to the time of eating, jet lag, light-dark cycle and motor activities [14]. 1.5. Aim of the Study Given the direct and indirect physiological effect of hy- pobaric-hypoxia on the cardiovascular system [15], fluc- tuations in autonomic cardiovascular regulation during exposure to high altitude [2], the increased risk of heart attack, and the instability of the cardiovascular system during sleep, this study analyzed HRV by calculating the average of the spectral component of stacked series of sequential power spectra from short ECG segments last- ing 0.5 minutes [16] during sleep: at sea level and be- tween 30 and 41 h of acclimatization at 3480 m altitude in a small sample of anthropometrically well character- ized mountain marathon runners [4-13]. The analysis of HRV during sleep at 3480 m can provide firmer ground for studying and diagnosing overtraining at high altitude of mountain marathon runners exposed to hypobaric-hy- poxic conditions. HRV measurements at high altitude may offer useful data for standard physiological evalua- tion and for formulating recommendations on increasing or reducing acclimatization time to defined hypobaric- hypoxic conditions and reduce cardiovascular distress at low altitude and during performance at high altitude in particular. 2. Material and Methods 2.1. Environment of the Group Five adult mountain marathon runners, native to sea level, with at least 20 years experience of competitive races and climbing at altitudes between 122 m and 5500 m, aged from 38 to 41 years, clinically and psychologically tested before, during and after competitions at high altitude (>2500 m) were recruited for this study [4-13]. The average body weight for the five subjects was 65.8 ± 4 kg; the average height was 176 ± 3.7 cm and the average aerobic power was 61.4 ± 2.7 ml·kg−1·min−1. The use of any drugs, dietary and neuroactive supplements was suspended for one week before the start of the study. Effects of light-dark and jet-lag interference on acclima- tization were excluded by the location of the study. 2.2. Polysomnographic Recording Polysomnographic recording procedures were carried out in accordance with Directive 86/609/EEC for experimen- tal human care. Informed consent prior to each experi- mental session was given by all five subjects and by the international medical staff of the Federation of Sport at Altitude. The study was conducted during normal sleep time (between 10 p.m. and 9 a.m.). Workouts were sus- pended on the days the measurements were taken. Polysomnographic recordings were taken in dedicated dark, isolated, silent rooms at 122 m (Milan, Italy) at a barometric pressure (PB) of 742 ± 7.7 mm Hg and after an acclimatization period of 30 - 32 or 38 - 41 h after reaching 3480 m at a PB of 495.4 ± 3.19 mm Hg. The recordings were performed to study the electroencepha- logram (EEG), submental electromyogram (EMG), elec- trooculogram (EOG), electrocardiogram (ECG) and the percent of peripheral arterial oxygen saturation (%SpaO2) signals. All signals were amplified and registered at a sampling rate of 250 Hz, then analyzed off-line accord- ing to standard criteria (Somnological 3, EmblaMedcare, Flaga®, Monza, Italy). Electrocardiographic recordings were taken with a bipolar derivation from two cardiac electrodes placed in V2 in the fourth left intercostal re- gion along the sternum, and in V4 in the fifth left inter- costal region on the hemiclavear line. The polysomnographic tracings containing the ECG signals were scored as follows: awakening during sleep (W), S1 + S2 and S3 + S4 of slow-wave sleep, also called NREM sleep, and REM sleep, according to stan- dard criteria developed by Rechtschaffen and Kales [17] in 30-second artifact-free epochs [16]. Automatic analysis of HRV values was performed us- ing Somnological 3 software (Embla), autoregressive model, order 12, following the rules of the Task Force [1] in a total of stacked series of sequential 30-second arti- fact-free epochs of awakening during sleep (W), S1 + S2 and S3 + S4 of NREM sleep, and of REM sleep. This analysis focused on the average of the R-R inter- vals, the power of the very low frequency (VLF), low frequency (LF) and high frequency (HF), the normalized unit of the low Frequency and high frequency (LFRRNU and HFRRNU), which were obtained by dividing the power of each component by the total variance, from which the VLF was subtracted, and by multiplying them by 100, and on the total power (TP) [Task Force 1].
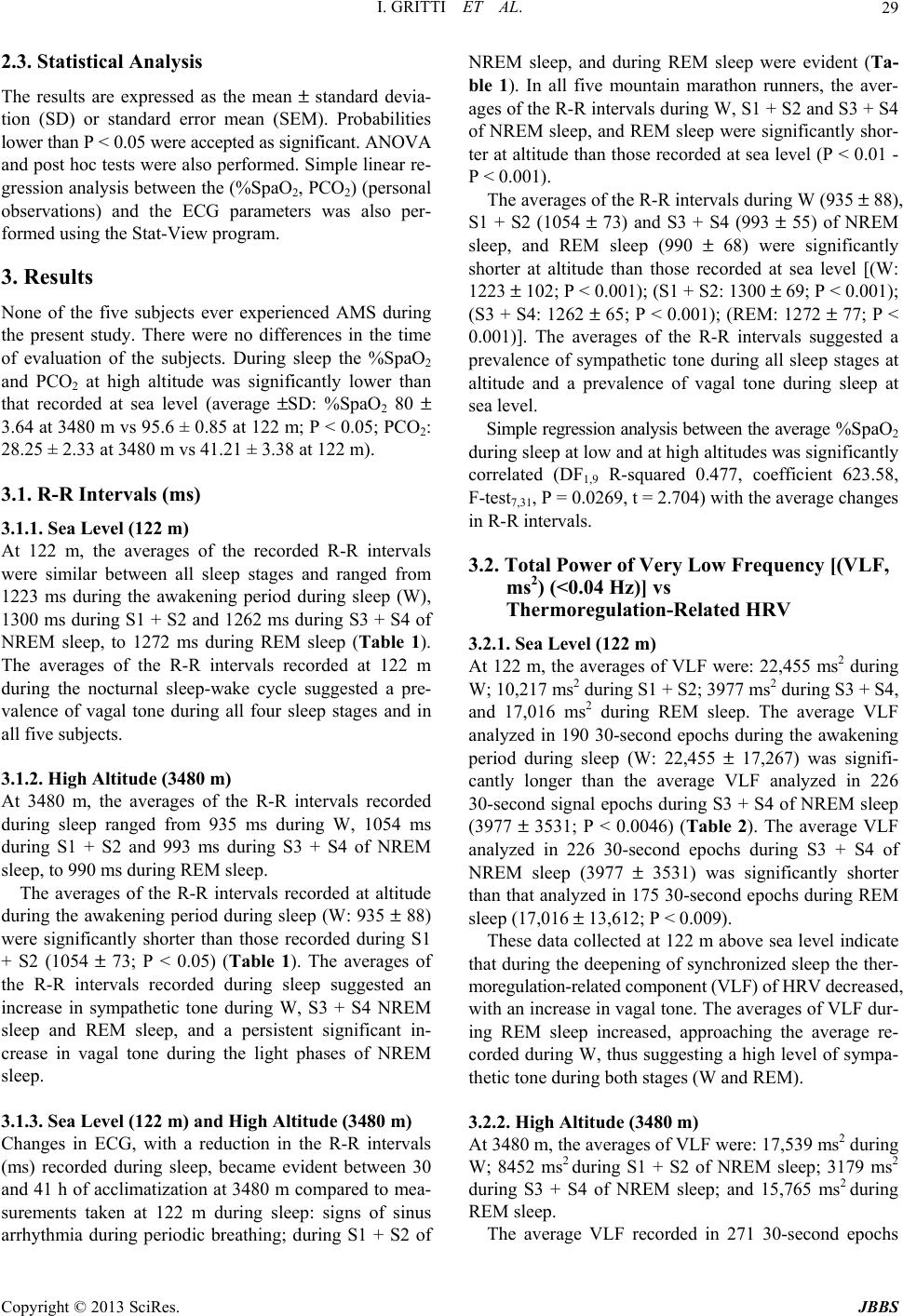 I. GRITTI ET AL. Copyright © 2013 SciRes. JBBS 29 2.3. Statistical Analysis The results are expressed as the mean ± standard devia- tion (SD) or standard error mean (SEM). Probabilities lower than P < 0.05 were accepted as significant. ANOVA and post hoc tests were also performed. Simple linear re- gression analysis between the (%SpaO2, PCO2) (personal observations) and the ECG parameters was also per- formed using the Stat-View program. 3. Results None of the five subjects ever experienced AMS during the present study. There were no differences in the time of evaluation of the subjects. During sleep the %SpaO2 and PCO2 at high altitude was significantly lower than that recorded at sea level (average ±SD: %SpaO2 80 ± 3.64 at 3480 m vs 95.6 ± 0.85 at 122 m; P < 0.05; PCO2: 28.25 ± 2.33 at 3480 m vs 41.21 ± 3.38 at 122 m). 3.1. R-R Intervals (ms) 3.1.1. Sea Level (122 m) At 122 m, the averages of the recorded R-R intervals were similar between all sleep stages and ranged from 1223 ms during the awakening period during sleep (W), 1300 ms during S1 + S2 and 1262 ms during S3 + S4 of NREM sleep, to 1272 ms during REM sleep (Table 1). The averages of the R-R intervals recorded at 122 m during the nocturnal sleep-wake cycle suggested a pre- valence of vagal tone during all four sleep stages and in all five subjects. 3.1.2. High Altitude (3480 m) At 3480 m, the averages of the R-R intervals recorded during sleep ranged from 935 ms during W, 1054 ms during S1 + S2 and 993 ms during S3 + S4 of NREM sleep, to 990 ms during REM sleep. The averages of the R-R intervals recorded at altitude during the awakening period during sleep (W: 935 ± 88) were significantly shorter than those recorded during S1 + S2 (1054 ± 73; P < 0.05) (Table 1). The averages of the R-R intervals recorded during sleep suggested an increase in sympathetic tone during W, S3 + S4 NREM sleep and REM sleep, and a persistent significant in- crease in vagal tone during the light phases of NREM sleep. 3.1.3. Sea Level (122 m) and High Altitude (3480 m) Changes in ECG, with a reduction in the R-R intervals (ms) recorded during sleep, became evident between 30 and 41 h of acclimatization at 3480 m compared to mea- surements taken at 122 m during sleep: signs of sinus arrhythmia during periodic breathing; during S1 + S2 of NREM sleep, and during REM sleep were evident (Ta- ble 1). In all five mountain marathon runners, the aver- ages of the R-R intervals during W, S1 + S2 and S3 + S4 of NREM sleep, and REM sleep were significantly shor- ter at altitude than those recorded at sea level (P < 0.01 - P < 0.001). The averages of the R-R intervals during W (935 ± 88), S1 + S2 (1054 ± 73) and S3 + S4 (993 ± 55) of NREM sleep, and REM sleep (990 ± 68) were significantly shorter at altitude than those recorded at sea level [(W: 1223 ± 102; P < 0.001); (S1 + S2: 1300 ± 69; P < 0.001); (S3 + S4: 1262 ± 65; P < 0.001); (REM: 1272 ± 77; P < 0.001)]. The averages of the R-R intervals suggested a prevalence of sympathetic tone during all sleep stages at altitude and a prevalence of vagal tone during sleep at sea level. Simple regression analysis between the average %SpaO2 during sleep at low and at high altitudes was significantly correlated (DF1,9 R-squared 0.477, coefficient 623.58, F-test7,31, P = 0.0269, t = 2.704) with the average changes in R-R intervals. 3.2. Total Power of Very Low Frequency [(VLF, ms2) (<0.04 Hz)] vs Thermoregulation-Related HRV 3.2.1. Sea Level (122 m) At 122 m, the averages of VLF were: 22,455 ms2 during W; 10,217 ms2 during S1 + S2; 3977 ms2 during S3 + S4, and 17,016 ms2 during REM sleep. The average VLF analyzed in 190 30-second epochs during the awakening period during sleep (W: 22,455 ± 17,267) was signifi- cantly longer than the average VLF analyzed in 226 30-second signal epochs during S3 + S4 of NREM sleep (3977 ± 3531; P < 0.0046) (Table 2). The average VLF analyzed in 226 30-second epochs during S3 + S4 of NREM sleep (3977 ± 3531) was significantly shorter than that analyzed in 175 30-second epochs during REM sleep (17,016 ± 13,612; P < 0.009). These data collected at 122 m above sea level indicate that during the deepening of synchronized sleep the ther- moregulation-related component (VLF) of HRV decreased, with an increase in vagal tone. The averages of VLF dur- ing REM sleep increased, approaching the average re- corded during W, thus suggesting a high level of sympa- thetic tone during both stages (W and REM). 3.2.2. High Altitude (3480 m) At 3480 m, the averages of VLF were: 17,539 ms2 during W; 8452 ms2 during S1 + S2 of NREM sleep; 3179 ms2 during S3 + S4 of NREM sleep; and 15,765 ms2 during REM sleep. The average VLF recorded in 271 30-second epochs
 I. GRITTI ET AL. Copyright © 2013 SciRes. JBBS 30 Table 1. Averages of the R-R intervals (ms) analysed in 5 altitude marathon runners, recorded in 30-second physiologically defined number of signal epochs according to the standard criteria developed by Rechtschaffen and Kales (1968) during the waking periods during sleep (W), stages S1 + S2 and S3 + S4 of NREM sleep and REM sleep, at 122 m and after 30 - 41 h of acclimatisation at 3480 m.(1) Stages Subjects 122 m 3480 m Epochs Epochs Awakening during sleep N. MeanS.D. N. MeanS.D. DeltaP value C.S 7 1212 56 35 1007 72 −2050.0001 M.R. 3 1051 76 3 817 37 −2340.0087 G.M. 13 1362 81 12 1061 203 −3010.0001 D.C. 140 1026 73 157 779 36 −2470.0001 S.S. 27 1463 224 64 1011 93 −4520.0001 No. of epochs 190 No. of subjects MeanS.E.M. No. of subjects MeanS.E.M. Delta subjects subjects Average 5 1223 102 5 935 88 −2780.001 S1 + S2 Epochs Epochs N. MeanS.D. N. MeanS.D. DeltaP value C.S 45 1281 60 34 1123 67 −1580.0001 M.R. 58 1130 55 52 878 41 −2520.0001 G.M. 53 1497 71 54 1236 86 −2610.0001 D.C. 302 1096 43 322 835 28 −2610.0001 S.S. 200 1494 115 237 1197 145 −2970.0001 No. of epochs 658 699 No. of subjects MeanS.E.M. No. of subjects MeanS.E.M. Delta subjects subjects Average 5 1300 69 5 1054 73 −2460.0001 S3 + S4 Epochs Epochs N. MeanS.D. N. MeanS.D. DeltaP value C.S 16 1277 48 9 1054 29 −2230.0001 M.R. 11 1121 42 6 833 8 −2880.0001 G.M. 9 1435 113 7 1165 112 −2700.0003 D.C. 98 1065 55 25 846 20 −2190.0001 S.S. 92 1410 65 59 1068 106 −3420.0001 No. of epochs 226 106 No. of subjects MeanS.E.M. No. of subjects MeanS.E.M. Delta subjects subjects Average 5 1262 65 5 993 55 −2680.001 REM Epochs Epochs N. MeanS.D. N. MeanS.D. DeltaP value C.S 8 1276 73 5 1128 120 −1470.0178 M.R. 23 1085 69 13 813 25 −2720.0001 G.M. 10 1450 60 13 1106 88 −3440.0001 D.C. 62 1062 56 30 832 40 −2310.0001 S.S. 72 1485 125 52 1069 67 −4160.0001 No. of epochs 175 No. of subjects MeanS.E.M. No. of subjects MeanS.E.M. Delta subjects subjects Average 5 1272 77 5 990 68 −2820.001 Footnotes to Table 1: (1)One-way ANOVA tracts analysed in the five mountain runners: the averages of the R-R intervals (ms) measured during the W, S1 + S2, S3 + S4 and REM sleep states were significantly different (DF 7,32,39: F-tests = 4.007, P = 0.003). The post-hoc comparison with Fisher analysis showed a significant difference (P < 0.05) between the average of the R-R intervals recorded during W at 122 m and that at 3480 m, and a significant difference between the average of the R-R intervals recorded during stages S3 + S4 at 3480 m and during REM sleep at 3480 m (P < 0.05). The average of the R-R intervals re- corded during W at 3480 m differed significantly from that during stages S1 + S2 at 122 m (P < 0.05). Post-hoc comparison with Fisher analysis showed that the average of the R-R intervals recorded during stages S3 + S4 at 122 m differed significantly from that during REM sleep at 122 m (P < 0.05). The average of the R-R intervals recorded during stages S1 + S2 at 122 m differed significantly from that during stages S1 + S2 at 3480 m (P < 0.05). Post-hoc comparison of the average of the R-R intervals recorded during stages S1 + S2 at 122 m differed significantly from that during S3 + S4 and REM sleep at 3480 m (P < 0.05). Fisher analysis demonstrated that the average of the R-R intervals recorded during stages S3 + S4 at 3480 m differed significantly from that during REM sleep at 122 m (P < 0.05). There was a significant difference between the R-R intervals recorded during REM sleep at 122 m and those during REM sleep at 3480 m (P < 0.05). *Post-hoc comparison with Student’s t-test showed that the average of the power of the R-R interval tract analysed in 271 30-second epochs during the waking period during sleep (W) at 3480 m was significantly lower (P < 0.05) than the average measured in 699 30-second epochs during stages S1 + S2 of NREM sleep at 3480 m in all 5 subjects.
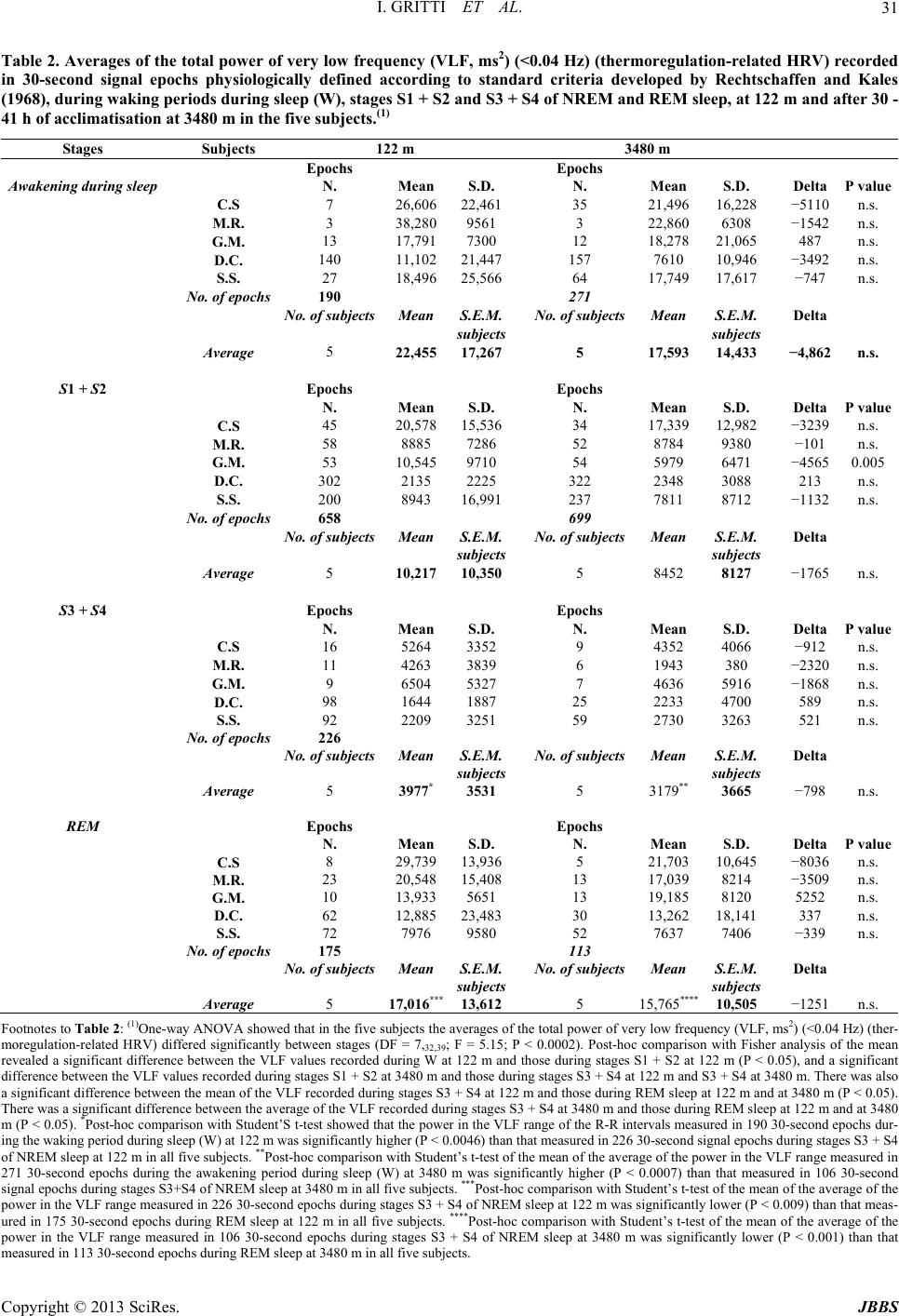 I. GRITTI ET AL. Copyright © 2013 SciRes. JBBS 31 Table 2. Averages of the total power of very low frequency (VLF, ms2) (<0.04 Hz) (thermoregulation-related HRV) recorded in 30-second signal epochs physiologically defined according to standard criteria developed by Rechtschaffen and Kales (1968), during waking periods during sleep (W), stages S1 + S2 and S3 + S4 of NREM and REM sleep, at 122 m and after 30 - 41 h of acclimatisation at 3480 m in the five subjects.(1) Stages Subjects 122 m 3480 m Epochs Epochs Awakening during sleep N. Mean S.D. N. Mean S.D. DeltaP value C.S 7 26,60622,46135 21,496 16,228 −5110n.s. M.R. 3 38,2809561 3 22,860 6308 −1542n.s. G.M. 13 17,7917300 12 18,278 21,065 487 n.s. D.C. 140 11,10221,447157 7610 10,946 −3492n.s. S.S. 27 18,49625,56664 17,749 17,617 −747n.s. No. of epochs 190 271 No. of subjectsMean S.E.M.No. of subjectsMean S.E.M. Delta subjects subjects Average 5 22,455 17,2675 17,593 14,433 −4,862n.s. S1 + S2 Epochs Epochs N. Mean S.D. N. Mean S.D. DeltaP value C.S 45 20,57815,53634 17,339 12,982 −3239n.s. M.R. 58 8885 7286 52 8784 9380 −101n.s. G.M. 53 10,5459710 54 5979 6471 −4565 0.005 D.C. 302 2135 2225 322 2348 3088 213 n.s. S.S. 200 8943 16,991237 7811 8712 −1132n.s. No. of epochs 658 699 No. of subjectsMean S.E.M.No. of subjectsMean S.E.M. Delta subjects subjects Average 5 10,217 10,3505 8452 8127 −1765n.s. S3 + S4 Epochs Epochs N. Mean S.D. N. Mean S.D. DeltaP value C.S 16 5264 3352 9 4352 4066 −912n.s. M.R. 11 4263 3839 6 1943 380 −2320n.s. G.M. 9 6504 5327 7 4636 5916 −1868n.s. D.C. 98 1644 1887 25 2233 4700 589 n.s. S.S. 92 2209 3251 59 2730 3263 521 n.s. No. of epochs 226 No. of subjectsMean S.E.M.No. of subjectsMean S.E.M. Delta subjects subjects Average 5 3977* 3531 5 3179** 3665 −798n.s. REM Epochs Epochs N. Mean S.D. N. Mean S.D. DeltaP value C.S 8 29,73913,9365 21,703 10,645 −8036n.s. M.R. 23 20,54815,40813 17,039 8214 −3509n.s. G.M. 10 13,9335651 13 19,185 8120 5252n.s. D.C. 62 12,88523,48330 13,262 18,141 337 n.s. S.S. 72 7976 9580 52 7637 7406 −339n.s. No. of epochs 175 113 No. of subjectsMean S.E.M.No. of subjectsMean S.E.M. Delta subjects subjects Average 5 17,016*** 13,612 5 15,765**** 10,505 −1251n.s. Footnotes to Table 2: (1)One-way ANOVA showed that in the five subjects the averages of the total power of very low frequency (VLF, ms2) (<0.04 Hz) (ther- moregulation-related HRV) differed significantly between stages (DF = 7,32,39; F = 5.15; P < 0.0002). Post-hoc comparison with Fisher analysis of the mean revealed a significant difference between the VLF values recorded during W at 122 m and those during stages S1 + S2 at 122 m (P < 0.05), and a significant difference between the VLF values recorded during stages S1 + S2 at 3480 m and those during stages S3 + S4 at 122 m and S3 + S4 at 3480 m. There was also a significant difference between the mean of the VLF recorded during stages S3 + S4 at 122 m and those during REM sleep at 122 m and at 3480 m (P < 0.05). There was a significant difference between the average of the VLF recorded during stages S3 + S4 at 3480 m and those during REM sleep at 122 m and at 3480 m (P < 0.05). *Post-hoc comparison with Student’S t-test showed that the power in the VLF range of the R-R intervals measured in 190 30-second epochs dur- ing the waking period during sleep (W) at 122 m was significantly higher (P < 0.0046) than that measured in 226 30-second signal epochs during stages S3 + S4 of NREM sleep at 122 m in all five subjects. **Post-hoc comparison with Student’s t-test of the mean of the average of the power in the VLF range measured in 271 30-second epochs during the awakening period during sleep (W) at 3480 m was significantly higher (P < 0.0007) than that measured in 106 30-second signal epochs during stages S3+S4 of NREM sleep at 3480 m in all five subjects. ***Post-hoc comparison with Student’s t-test of the mean of the average of the power in the VLF range measured in 226 30-second epochs during stages S3 + S4 of NREM sleep at 122 m was significantly lower (P < 0.009) than that meas- ured in 175 30-second epochs during REM sleep at 122 m in all five subjects. ****Post-hoc comparison with Student’s t-test of the mean of the average of the power in the VLF range measured in 106 30-second epochs during stages S3 + S4 of NREM sleep at 3480 m was significantly lower (P < 0.001) than that measured in 113 30-second epochs during REM sleep at 3480 m in all five subjects.
 I. GRITTI ET AL. Copyright © 2013 SciRes. JBBS 32 during the awakening period during sleep [(W) (17,593 ± 14,433)] was significantly longer than the average VLF recorded in 106 30-second signal epochs during S3 + S4 of NREM sleep (3179 ± 3665; P < 0.0007) (Table 2). The average VLF in 106 30-second epochs during S3 + S4 of NREM sleep (3179 ± 3665) was significantly shor- ter than that analyzed in 113 30-second epochs of REM sleep (15,765 ± 10,505; P < 0.05). Overall, the averaged data recorded during sleep at 3480 m demonstrate that during the deepening of syn- chronized sleep the thermoregulation-related component (VLF) of HRV decreased, with a physiological increase in vagal tone. During REM sleep the thermoregulation- related component of HRV approached that recorded during the awakening period during sleep (W) indicating an increase in the sympathetic tone . 3.2.3. Sea Level (122 m) and High Altitude (3480 m) The average of the total power of very low frequency (VLF, ms2) recorded during S1 + S2 of NREM sleep (5979 ± 6471) was significantly shorter at high altitude than that recorded sea level (10,545 ± 9710; P < 0.005) in only 1/5 (GM) mountain marathon runners (Table 2). 3.3. Total Power of Low Frequency [(LF, ms2) (0.04 - 0.15 Hz)] Range. The LF Appears to Have a Widespread Neuronal Genesis and Is Considered as a Marker of Sympathetic Modulation or a Marker of Both Sympathetic and Vagal Modulation 3.3.1. Sea Level (122 m) At 122 m, the averages of LF were: 7997 ms2 during W; 7400 ms2 during S1 + S2; 4881 ms2 during S3 + S4; and 8579 ms2 during REM sleep. The changes in the average total power in LF were significantly shorter during S3 + S4 than those observed during REM sleep (4881 ± 2041 vs 8579 ± 3473; P < 0.02) (Table 3). These data suggest that the average values of LF, a marker of sympathetic modulation, decreased during the deepening phases of NREM sleep. During REM sleep the average values of LF significantly increased, approaching a value similar to that observed during W. 3.3.2. High Altitude (3480 m) At 3480 m, the averages of LF were: 6272 ms2 during W; 7516 ms2 during S1 + S2; 3805 ms2 during S3 + S4; and 5715 ms2 during REM sleep (Table 3). The results dem- onstrate that the average values of LF during sleep at altitude did not change significantly between the differ- ent sleep stages (Table 3). 3.3.3. Sea Level (122 m) and High Altitude (3480 m) The averages of total power of low frequency (LF ms2) recorded at high altitude during W were significantly shorter than those recorded at sea level in 3/5 subjects [(CS: 3163 ± 1400 at 3480 m vs 5148 ± 3163 at 122 m; P < 0.0074); (GM: 6157 ± 2271 at 3480 m vs 9656 ± 2978 at 122 m; P < 0.0033); (DC: 2774 ± 2056 at 3480 m vs 5083 ± 4655 at 122 m; P < 0.0001)] (Table 3). The aver- age LF recorded during S1 + S2 was significantly longer at altitude than that recorded at sea level in 1/5 subjects (SS: 17,947 ± 12,895 at 3480 m vs 10,155 ± 6434 at 122 m; P < 0.0001); the LF was significantly shorter in 2/5 subjects [(CS: 3539 ± 1734 at 3480 m vs 5946 ± 2294 at 122 m; P < 0.0001); (MR: 6059 ± 1641 at 3480 m vs 10,118 ± 2699 at 122 m; P < 0.0001)]; the average LF recorded during S3 + S4 was significantly shorter at alti- tude than that recorded at 122 m in 2/5 [(CS: 2,276 ± 2427 at 3480 m vs 3760 ± 1098 at 122 m; P < 0.0455); (MR: 3917 ± 592 at 3480 m vs 8344 ± 222 at 122 m; P < 0.0003)]; the average LF recorded during REM sleep was significantly shorter at altitude than that recorded at sea level in 3/5 subjects [(MR: 5200 ± 1513 at 3480 m vs 10159 ± 2790 at 122 m; P < 0.0001); (DC: 3702 ± 1955 at 3480 m vs 6727 ± 4144 at 122 m; P < 0.0003); (SS: 5, 140 ± 4402 at 3480 m vs 11,509 ± 6775 at 122 m; P < 0.0001)]. These data demonstrate great variability in the sympathetic LF component of HRV recorded at low and high altitudes in the five mountain marathon runners. 3.4. Low-Frequency in Normalized Units [(LFRRNU) (0.04 - 0.15 Hz)] Range. LFRRNU Is Considered as a Marker of Sympathetic Modulation 3.4.1. Sea Level (122 m) In all 5 subjects, the average LFRRNU recorded in 190 30-second epochs during the awakening period during sleep (W: 73.41 ± 10.15) differed significantly from that recorded in 226 30-second epochs during S3 + S4 of NREM sleep (64.76 ± 11.45; P < 0.03). In all five moun- tain marathon runners, the average LFRRNU during REM sleep was significantly longer (74.32 ± 10.17) than that observed during S3 + S4 (64.76 ± 11.45; P < 0.0192) (Table 4). These data indicate that the marker of sympa- thetic modulation (LFRRNU) decreased during deepening of sleep. During REM sleep, the average value of LFRRNU was similar to that recorded during the awakening period during sleep (W), suggesting that both desynchronized states were supported by an increase in sympathetic tone. 3.4.2. High Altitude (3480 m) At 3480 m, the average LFRRNU values recorded during all four stages of sleep were similar and ranged from 75.77 during W, 76.25 during S1 + S2, 66.62 during S3 + S4, to 80.97 during REM sleep (Table 4).
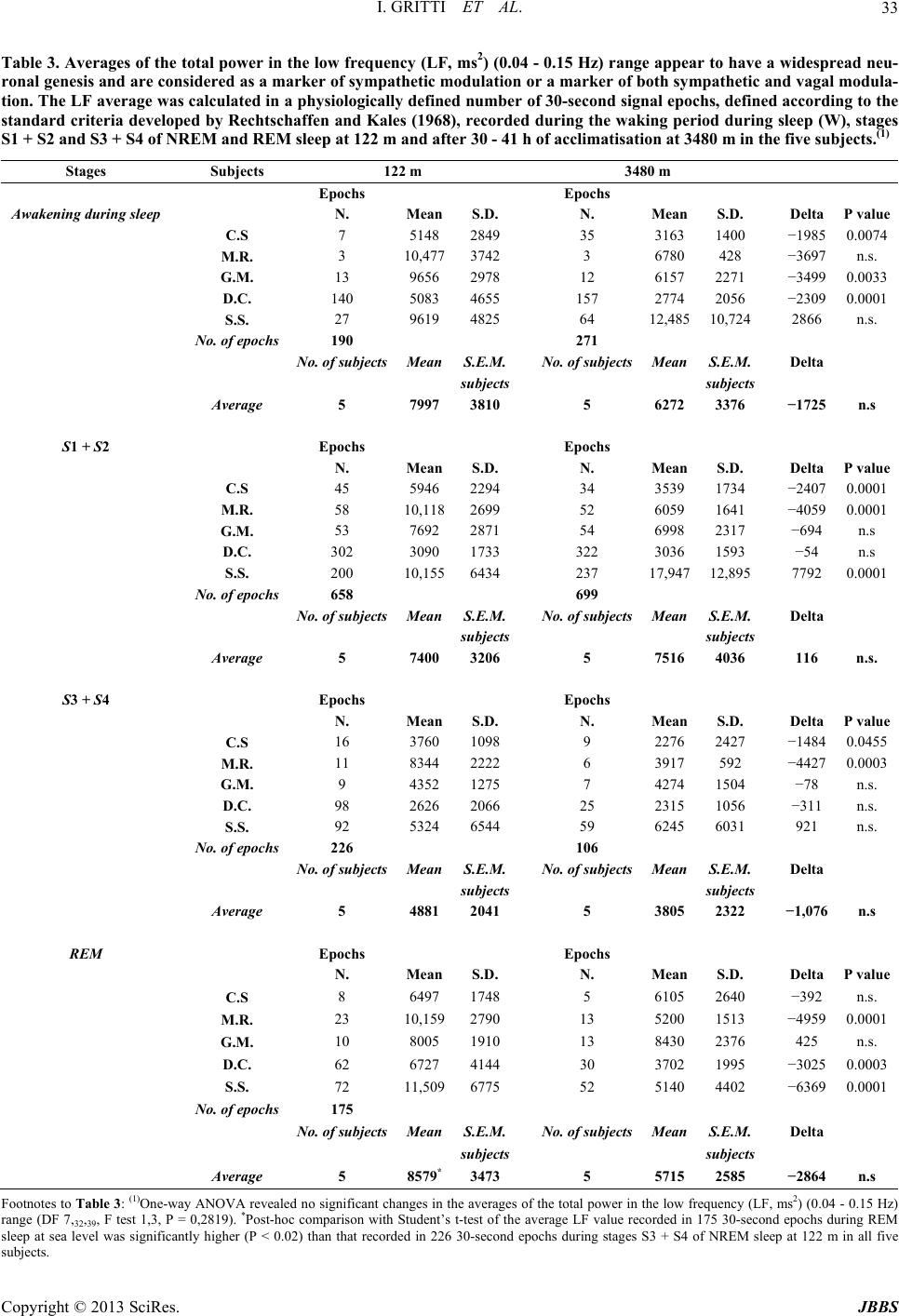 I. GRITTI ET AL. Copyright © 2013 SciRes. JBBS 33 Table 3. Averages of the total power in the low frequency (LF, ms2) (0.04 - 0.15 Hz) range appear to have a widespread neu- ronal genesis and are considered as a marker of sympathetic modulation or a marker of both sympathetic and vagal modula- tion. The LF average was calculated in a physiologically defined number of 30-second signal epochs, defined according to the standard criteria developed by Rechtschaffen and Kales (1968), recorded during the waking period during sleep (W), stages S1 + S2 and S3 + S4 of NREM and REM sleep at 122 m and after 30 - 41 h of acclimatisation at 3480 m in the five subjects.(1) Stages Subjects 122 m 3480 m Epochs Epochs Awakening during sleep N. MeanS.D. N. Mean S.D. DeltaP value C.S 7 51482849 35 3163 1400 −1985 0.0074 M.R. 3 10,4773742 3 6780 428 −3697n.s. G.M. 13 96562978 12 6157 2271 −3499 0.0033 D.C. 140 50834655 157 2774 2056 −2309 0.0001 S.S. 27 96194825 64 12,485 10,724 2866n.s. No. of epochs 190 271 No. of subjectsMeanS.E.M.No. of subjectsMean S. E.M. Delta subjects subjects Average 5 79973810 5 6272 3376 −1725n.s S1 + S2 Epochs Epochs N. MeanS.D. N. Mean S.D. DeltaP value C.S 45 59462294 34 3539 1734 −2407 0.0001 M.R. 58 10,1182699 52 6059 1641 −4059 0.0001 G.M. 53 76922871 54 6998 2317 −694n.s D.C. 302 30901733 322 3036 1593 −54 n.s S.S. 200 10,1556434 237 17,947 12,895 77920.0001 No. of epochs 658 699 No. of subjectsMeanS.E.M.No. of subjectsMean S.E.M. Delta subjects subjects Average 5 74003206 5 7516 4036 116 n.s. S3 + S4 Epochs Epochs N. MeanS.D. N. Mean S.D. DeltaP value C.S 16 37601098 9 2276 2427 −1484 0.0455 M.R. 11 83442222 6 3917 592 −4427 0.0003 G.M. 9 43521275 7 4274 1504 −78 n.s. D.C. 98 26262066 25 2315 1056 −311n.s. S.S. 92 53246544 59 6245 6031 921 n.s. No. of epochs 226 106 No. of subjectsMeanS.E.M.No. of subjectsMean S. E.M. Delta subjects subjects Average 5 48812041 5 3805 2322 −1,076n.s REM Epochs Epochs N. MeanS.D. N. Mean S.D. DeltaP value C.S 8 64971748 5 6105 2640 −392n.s. M.R. 23 10,1592790 13 5200 1513 −4959 0.0001 G.M. 10 80051910 13 8430 2376 425 n.s. D.C. 62 67274144 30 3702 1995 −3025 0.0003 S.S. 72 11,5096775 52 5140 4402 −6369 0.0001 No. of epochs 175 No. of subjectsMeanS.E.M.No. of subjectsMean S. E.M. Delta subjects subjects Average 5 8579*3473 5 5715 2585 −2864n.s Footnotes to Table 3: (1)One-way ANOVA revealed no significant changes in the averages of the total power in the low frequency (LF, ms2) (0.04 - 0.15 Hz) range (DF 7,32,39, F test 1,3, P = 0,2819). *Post-hoc comparison with Student’s t-test of the average LF value recorded in 175 30-second epochs during REM sleep at sea level was significantly higher (P < 0.02) than that recorded in 226 30-second epochs during stages S3 + S4 of NREM sleep at 122 m in all five subjects.
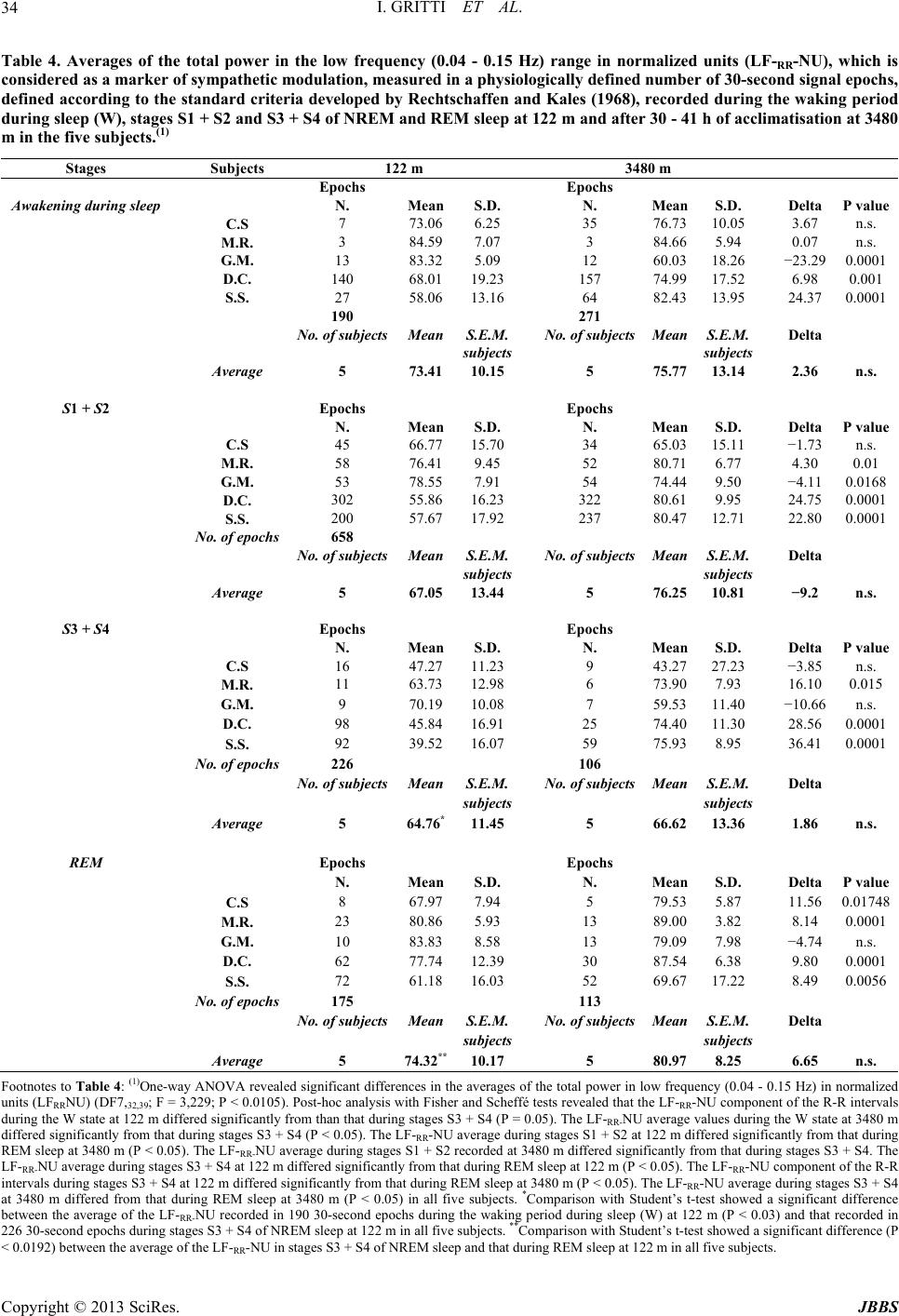 I. GRITTI ET AL. Copyright © 2013 SciRes. JBBS 34 Table 4. Averages of the total power in the low frequency (0.04 - 0.15 Hz) range in normalized units (LF-RR-NU), which is considered as a marker of sympathetic modulation, measured in a physiologically defined number of 30-second signal epochs, defined according to the standard criteria developed by Rechtschaffen and Kales (1968), recorded during the waking period during sleep (W), stages S1 + S2 and S3 + S4 of NREM and REM sleep at 122 m and after 30 - 41 h of acclimatisation at 3480 m in the five subjects.(1) Stages Subjects 122 m 3480 m Epochs Epochs Awakening during sleep N. MeanS.D. N. Mean S.D. DeltaP value C.S 7 73.066.25 35 76.73 10.05 3.67 n.s. M.R. 3 84.597.07 3 84.66 5.94 0.07 n.s. G.M. 13 83.325.09 12 60.03 18.26 −23.29 0.0001 D.C. 140 68.0119.23 157 74.99 17.52 6.98 0.001 S.S. 27 58.0613.16 64 82.43 13.95 24.370.0001 190 271 No. of subjectsMeanS.E.M.No. of subjectsMean S.E.M. Delta subjects subjects Average 5 73.4110.15 5 75.77 13.14 2.36 n.s. S1 + S2 Epochs Epochs N. MeanS.D. N. Mean S.D. DeltaP value C.S 45 66.7715.70 34 65.03 15.11 −1.73n.s. M.R. 58 76.419.45 52 80.71 6.77 4.30 0.01 G.M. 53 78.557.91 54 74.44 9.50 −4.11 0.0168 D.C. 302 55.8616.23 322 80.61 9.95 24.750.0001 S.S. 200 57.6717.92 237 80.47 12.71 22.800.0001 No. of epochs 658 No. of subjectsMeanS.E.M.No. of subjectsMean S.E.M. Delta subjects subjects Average 5 67.0513.44 5 76.25 10.81 −9.2 n.s. S3 + S4 Epochs Epochs N. MeanS.D. N. Mean S.D. DeltaP value C.S 16 47.2711.23 9 43.27 27.23 −3.85n.s. M.R. 11 63.7312.98 6 73.90 7.93 16.100.015 G.M. 9 70.1910.08 7 59.53 11.40 −10.66n.s. D.C. 98 45.8416.91 25 74.40 11.30 28.560.0001 S.S. 92 39.5216.07 59 75.93 8.95 36.410.0001 No. of epochs 226 106 No. of subjectsMeanS.E.M.No. of subjectsMean S.E.M. Delta subjects subjects Average 5 64.76*11.45 5 66.62 13.36 1.86 n.s. REM Epochs Epochs N. MeanS.D. N. Mean S.D. DeltaP value C.S 8 67.977.94 5 79.53 5.87 11.560.01748 M.R. 23 80.865.93 13 89.00 3.82 8.14 0.0001 G.M. 10 83.838.58 13 79.09 7.98 −4.74n.s. D.C. 62 77.7412.39 30 87.54 6.38 9.80 0.0001 S.S. 72 61.1816.03 52 69.67 17.22 8.49 0.0056 No. of epochs 175 113 No. of subjectsMeanS.E.M.No. of subjectsMean S.E.M. Delta subjects subjects Average 5 74.32** 10.17 5 80.97 8.25 6.65 n.s. Footnotes to Table 4: (1)One-way ANOVA revealed significant differences in the averages of the total power in low frequency (0.04 - 0.15 Hz) in normalized units (LFRRNU) (DF7,32,39; F = 3,229; P < 0.0105). Post-hoc analysis with Fisher and Scheffé tests revealed that the LF-RR-NU component of the R-R intervals during the W state at 122 m differed significantly from than that during stages S3 + S4 (P = 0.05). The LF-RR-NU average values during the W state at 3480 m differed significantly from that during stages S3 + S4 (P < 0.05). The LF-RR-NU average during stages S1 + S2 at 122 m differed significantly from that during REM sleep at 3480 m (P < 0.05). The LF-RR-NU average during stages S1 + S2 recorded at 3480 m differed significantly from that during stages S3 + S4. The LF-RR-NU average during stages S3 + S4 at 122 m differed significantly from that during REM sleep at 122 m (P < 0.05). The LF-RR-NU component of the R-R intervals during stages S3 + S4 at 122 m differed significantly from that during REM sleep at 3480 m (P < 0.05). The LF-RR-NU average during stages S3 + S4 at 3480 m differed from that during REM sleep at 3480 m (P < 0.05) in all five subjects. *Comparison with Student’s t-test showed a significant difference between the average of the LF-RR- NU recorded in 190 30-second epochs during the waking period during sleep (W) at 122 m (P < 0.03) and that recorded in 226 30-second epochs during stages S3 + S4 of NREM sleep at 122 m in all five subjects. **Comparison with Student’s t-test showed a significant difference (P < 0.0192) between the average of the LF-RR-NU in stages S3 + S4 of NREM sleep and that during REM sleep at 122 m in all five subjects.
 I. GRITTI ET AL. Copyright © 2013 SciRes. JBBS 35 3.4.3. Sea Level (122 m) and High Altitude (3480 m) The average LFRRNU (Table 4) recorded during W at al- titude was significantly shorter than that recorded at sea level in 1/5 subjects (GM: 60.03 ± 18.26 at 3480 m vs 83.32 ± 5.09 at 122 m; P < 0.0001) and significantly longer in 2/5 subjects [(DC: 74.99 ± 17.52 at 3480 m vs 68.01 ± 19.23 at 122 m; P < 0.001); (SS: 82.43 ± 13.95 at 3480 m vs 58.06 ± 13.16 at 122 m; P < 0.0001)]. The average LFRRNU recorded during S1 + S2 at high altitude was significantly longer than that recorded at sea level in 3/5 subjects [(MR: 80.71 ± 6.77 at 3480 m vs 76.41 ± 9.45 at 122 m; P < 0.01); (DC: 80.61 ± 9.95 at 3480 m vs 55.86 ± 16.23 at 122 m; P < 0.0001); (SS: 80.47 ± 12.71 at 3480 m vs 57.67 ± 17.92 at 122 m; P < 0.0001)], while in 1/5 the average LFRRNU was signifi- cantly shorter (GM: 59.53 ± 11.40 at 3480 m vs 70.19 ± 10.08 at 122 m; P < 0.0168) in S3 + S4. The average LFRRNU recorded during S3 + S4 at high altitude was significantly longer than that recorded at 122 m in 3/5 subjects [(MR: 73.90 ± 7.93 at 3480 m vs 63.73 ± 12.98 at 122 m; P < 0.0150); (DC: 74.40 ± 11.30 at 3480 m vs 45.84 ± 16.91 at 122 m; P < 0.0001); (SS: 75.93 ± 8.95 at 3480 m vs 39.52 ± 16.07 at 122 m; P < 0.0001)]. The average LFRRNU recorded during REM sleep at high altitude was significantly longer than that recorded at 122 m in 4/5 subjects [(CS: 79.53 ± 5.87 at 3480 m vs 67.97 ± 7.94 at 122 m; P < 0.01748); (MR: 89.00 ± 3.82 at 3480 m vs 80.86 ± 5.93 at 122 m; P < 0.0001); (DC: 87.54 ± 6.38 at 3480 m vs 77.74 ± 12.39 at 122 m; P < 0.0001); (SS: 69.67 ± 17.22 at 3480 m vs 61.18 ± 16.03 at 122 m; P < 0.0056)]. These data show a great variabil- ity between mountain marathon runners in LFRRNU when the data set recorded at 122 m is compared with that re- corded at 3480 m. Analysis of the data recorded in indi- vidual mountain marathon runners showed an increase in the average LFRRNU at 3480 m, demonstrating an in- crease in the marker for sympathetic modulation. 3.5. Total Power of High Frequency [(HF, ms2) (0.15 - 0.4 Hz)] Range. HF Primarily Reflects Respiratory-Driven Vagal Modulation of Sinus Rhythm 3.5.1. Sea Level (122 m) No significant differences in the averages of HF were observed between W (2223 ± 728), S1 + S2 (2945 ± 1080) and S3 + S4 (3891 ± 1134) and REM (2499 ± 879) stages at low altitude (Table 5). The averages of the HF recorded during the nocturnal sleep-wake cycle at 122 m suggested a prevalence of vagal tone during S3 + S4 stages in comparison with the other sleep stages. 3.5.2. High Altitude (3480 m) No significant differences in the averages of HF were observed between W (1572 ± 993), S1 + S2 (1610 ± 631) and S3 + S4 (1480 ± 511) and REM (1159 ± 555) stages at 3480 m (Table 5). The averages of the HF recorded during the nocturnal sleep-wake cycle at 3480 m sug- gested a reduction in vagal tone throughout all sleep stages and during awakening during sleep. 3.5.3. Sea Level (122 m) and High Altitude (3480 m) Compared with the values calculated at sea level, there was a significant decrease in the average of total power of high frequency (HF, ms2) (Table 5) recorded during W at altitude in 3/5 subjects [(CS: 1422 ± 384 at 122 m vs 743 ± 191 at 3480 m; P < 0.0001); (DC: 1487 ± 729 at 122 m vs 717 ± 789 at 3480 m; P < 0.0001); (SS: 4849 ± 1587 at 122 m vs 1674 ± 1179 at 3480 m; P < 0.0001)] and a significant increase in 1/5 subjects (GM: 1654 ± 400 at 122 m vs 3495 ± 2196 at 3480 m; P < 0.0072). Compared with the values calculated at sea level, there was a significant decrease in the average HF recorded at altitude during S1 + S2 of NREM sleep in 4/5 subjects [(CS: 2485 ± 1178 at 122 m vs 1513 ± 443 at 3480 m; P < 0.0001); (MR: 2892 ± 906 at 122 m vs 1382 ± 546 at 3480 m; P < 0.0001); (DC: 2167 ± 778 at 122 m vs 605 ± 297 at 3480 m; P < 0.0001); (SS: 5375 ± 1950 at 122 m vs 2640 ± 1385 at 3480 m; P < 0.0001)]. Compared with the averages calculated at 122 m, there was a significant decrease in the average HF recorded during S3 + S4 of NREM sleep at 3480 m in 4/5 subjects [(CS: 3776 ± 982 at 122 m vs 2044 ± 723 at 3480 m; P < 0.0001); (MR: 4624 ± 1629 at 122 m vs 975 ± 506 at 3480 m; P < 0.0001); (DC: 2617 ± 777 at 122 m vs 684 ± 249 at 3480 m; P < 0.0001); (SS: 6,866 ± 1904 at 122 m vs 1394 ± 681 at 3480 m; P < 0.0001)] and a significant increase in 1/5 subjects (GM: 1571 ± 376 at 122 m vs 2303 ± 396 at 3480 m; P < 0.0021). There was a significant decrease in the average HF recorded during REM sleep in 4/5 sub- jects [(CS: 2425 ± 531 at 122 m vs 1397 ± 774 at 3480 m; P < 0.0155); (MR: 2248 ± 680 at 122 m vs 615 ± 233 at 3480 m; P < 0.0001); (DC: 1530 ± 708 at 122 m vs 400 ± 149 at 3480 m; P < 0.0001); (SS: 4885 ± 1559 at 122 m vs 1671 ± 1147 at 3480 m; P < 0.0001)]. The average HF at 3480 m recorded in individual mountain marathon runners was generally lower than that recorded at 122 m, suggesting a decrease in the marker of vagal tone at altitude. 3.6. High Frequency in Normalized Units [(HFRRNU) (0.04 - 0.15 Hz)]. HFRRNU Is Considered as a Marker of Vagal Modulation 3.6.1. Sea Level (122 m) In all five subjects, the average of the HFRRNU analyzed
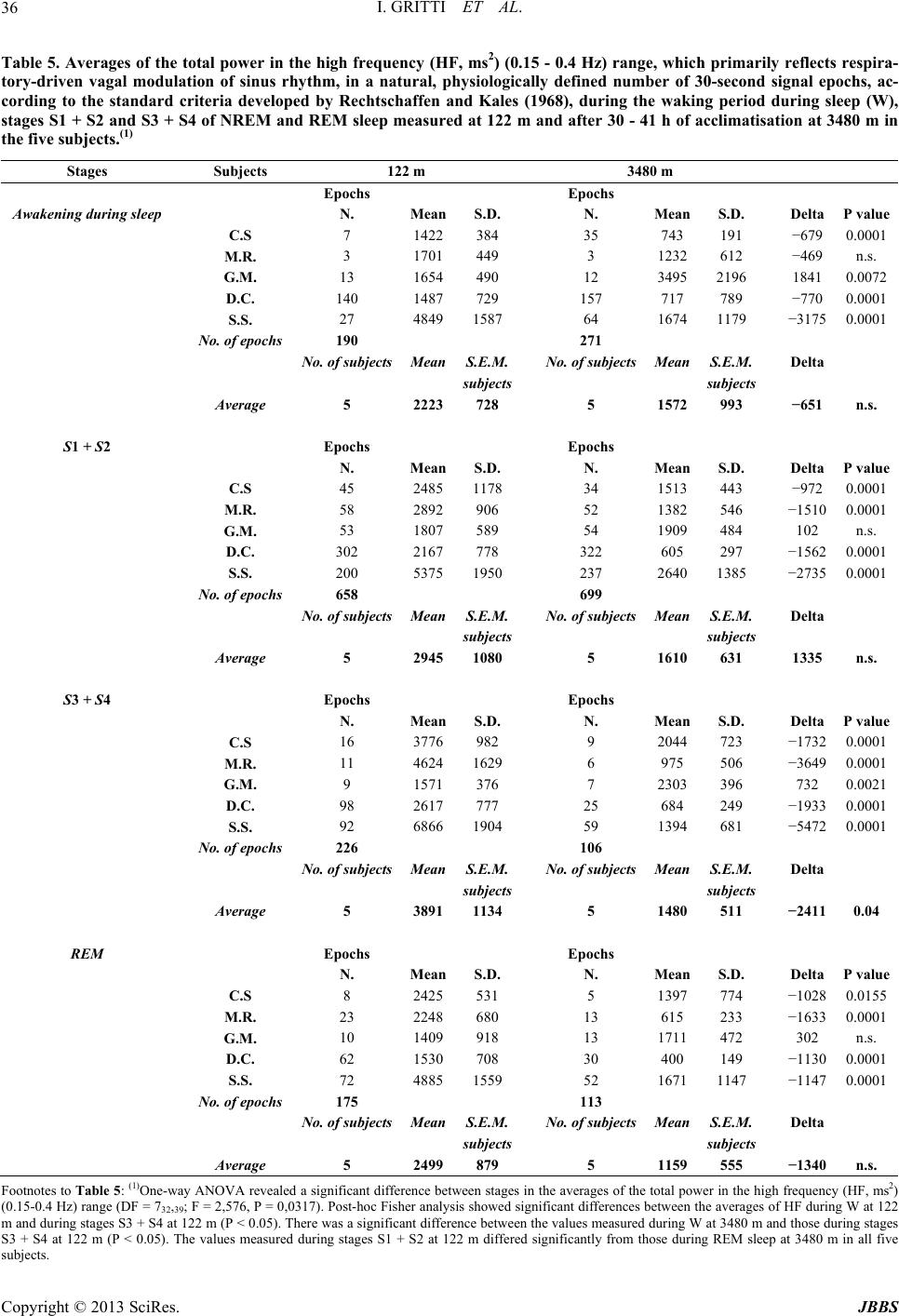 I. GRITTI ET AL. Copyright © 2013 SciRes. JBBS 36 Table 5. Averages of the total power in the high frequency (HF, ms2) (0.15 - 0.4 Hz) range, which primarily reflects respira- tory-driven vagal modulation of sinus rhythm, in a natural, physiologically defined number of 30-second signal epochs, ac- cording to the standard criteria developed by Rechtschaffen and Kales (1968), during the waking period during sleep (W), stages S1 + S2 and S3 + S4 of NREM and REM sleep measured at 122 m and after 30 - 41 h of acclimatisation at 3480 m in the five subjects.(1) Stages Subjects 122 m 3480 m Epochs Epochs Awakening during sleep N. MeanS.D. N. Mean S.D. DeltaP value C.S 7 1422384 35 743 191 −679 0.0001 M.R. 3 1701449 3 1232 612 −469n.s. G.M. 13 1654490 12 3495 2196 18410.0072 D.C. 140 1487729 157 717 789 −770 0.0001 S.S. 27 48491587 64 1674 1179 −3175 0.0001 No. of epochs 190 271 No. of subjectsMeanS.E.M.No. of subjectsMean S.E.M. Delta subjects subjects Average 5 2223728 5 1572 993 −651n.s. S1 + S2 Epochs Epochs N. MeanS.D. N. Mean S.D. DeltaP value C.S 45 24851178 34 1513 443 −972 0.0001 M.R. 58 2892906 52 1382 546 −1510 0.0001 G.M. 53 1807589 54 1909 484 102 n.s. D.C. 302 2167778 322 605 297 −1562 0.0001 S.S. 200 53751950 237 2640 1385 −2735 0.0001 No. of epochs 658 699 No. of subjectsMeanS.E.M.No. of subjectsMean S.E.M. Delta subjects subjects Average 5 29451080 5 1610 631 1335n.s. S3 + S4 Epochs Epochs N. MeanS.D. N. Mean S.D. DeltaP value C.S 16 3776982 9 2044 723 −1732 0.0001 M.R. 11 46241629 6 975 506 −3649 0.0001 G.M. 9 1571376 7 2303 396 732 0.0021 D.C. 98 2617777 25 684 249 −1933 0.0001 S.S. 92 68661904 59 1394 681 −5472 0.0001 No. of epochs 226 106 No. of subjectsMeanS.E.M.No. of subjectsMean S.E.M. Delta subjects subjects Average 5 38911134 5 1480 511 −24110.04 REM Epochs Epochs N. MeanS.D. N. Mean S.D. DeltaP value C.S 8 2425531 5 1397 774 −1028 0.0155 M.R. 23 2248680 13 615 233 −1633 0.0001 G.M. 10 1409918 13 1711 472 302 n.s. D.C. 62 1530708 30 400 149 −1130 0.0001 S.S. 72 48851559 52 1671 1147 −1147 0.0001 No. of epochs 175 113 No. of subjectsMeanS.E.M.No. of subjectsMean S.E.M. Delta subjects subjects Average 5 2499879 5 1159 555 −1340n.s. Footnotes to Table 5: (1)One-way ANOVA revealed a significant difference between stages in the averages of the total power in the high frequency (HF, ms2) (0.15-0.4 Hz) range (DF = 732,39; F = 2,576, P = 0,0317). Post-hoc Fisher analysis showed significant differences between the averages of HF during W at 122 m and during stages S3 + S4 at 122 m (P < 0.05). There was a significant difference between the values measured during W at 3480 m and those during stages S3 + S4 at 122 m (P < 0.05). The values measured during stages S1 + S2 at 122 m differed significantly from those during REM sleep at 3480 m in all five subjects.
 I. GRITTI ET AL. Copyright © 2013 SciRes. JBBS 37 in a natural, physiologically defined number of 30-sec- ond signal epochs, according to standard criteria de- veloped by Rechtschaffen and Kales (1968), during the awakening period during sleep (W: 23.47 ± 9.46) was significantly shorter than that observed during S3 + S4 of NREM at 122 m (56.91 ± 31.14; P < 0.0327). In all five subjects, the average of the HFRRNU during S3 + S4 of NREM sleep (56.91 ± 31.14) was significantly longer (P < 0.01004) than that observed during REM sleep (22.64 ± 9.44; P < 0.01004) at 122 m (Table 6). These data sug- gest an increase in vagal modulation during the deepen- ing of slow-wave NREM sleep and a decrease in vagal modulation during the desynchronized awakening period during sleep (W) and REM sleep. 3.6.2. High Altitude (3480 m) The average of the HFRRNU analyzed between W (21.31 ± 11.08), S1 + S2 (21.52 ± 9.76), S3 + S4 (31.11 ± 12.81) of NREM sleep and REM sleep (16.74 ± 6.99) was simi- lar (Table 6). The data suggest an increase in vagal mo- dulation during S3 + S4 of slow-wave NREM sleep and a decrease in vagal modulation during the desynchronized awakening period during sleep (W), S1 + S2 of NREM sleep and during REM sleep. 3.6.3. Sea Level (122 m) and High Altitude (3480 m) The mean of the averages of the HFRRNU (Table 6) re- corded during W at altitude was significantly shorter than that recorded at sea level in 2/5 subjects (DC: 22.29 ± 14.47 at 3480 m vs 30.87 ± 18.75 at 122 m; P < 0.0001); (SS: 15.33 ± 11.65 at 3480 m vs 33.49 ± 11.57 at 122 m; P < 0.0001)] and significantly longer in 1/5 subjects (GM: 33.15 ± 13.39 at 3480 m vs 15.15 ± 4.6 at 122 m; P < 0.0001). The mean of the averages of the HFRRNU re- corded during S1 + S2 at altitude was significantly shor- ter than that recorded at sea level in 3/5 subjects (MR: 18.81 ± 6.71 at 3480 m vs 23.17 ± 9.48 at 122 m; P < 0.01); (DC: 18.32 ± 9.43 at 3480 m vs 43.57 ± 16.23 at 122 m; P < 0.001); (SS: 16.38 ± 10.36 at 3480 m vs 36.86 ± 17.23 at 122 m; P < 0.0001). The mean of the averages of the HFRR-NU recorded during S3 + S4 at al- titude was significantly shorter than that recorded at sea level in 2/5 subjects [(DC: 24.42 ± 11.04 at 3480 m vs 53.53 ± 16.73 at 122 m; P < 0.0001); (SS: 22.29 ± 8.30 at 3480 m vs 55.54 ± 15.78 at 122 m; P < 0.0001)]. The mean of the averages of the HFRR-NU recorded during REM sleep at altitude was significantly shorter than that at sea level in 3/5 subjects [(MR: 10.57 ± 3.76 at 3480 m vs 18.50 ± 5.82 at 122 m: P < 0.0001); (DC: 11.56 ± 6.14 at 3480 m vs 21.80 ± 12.24 at 122 m; P < 0.0001); (SS: 26.81 ± 14.55 at 3480 m vs 32.15 ± 14.57 at 122 m; P < 0.04)]. 3.7. LF:HF Ratio Mirrors Sympathovagal Balance or Reflects Sympathetic Modulation 3.7.1. Sea Level (122 m) In all five subjects, the average of the LF:HF ratio during W at 122 m was longer (4.6446 ± 2.8655) than that ob- served during S3 + S4 (1.6733 ± 1.0847; P < 0.0110) (Table 7); the average of the LF:HF ratio during S3+S4 (1.6733 ± 1.0847) was significantly shorter than that ob- served in 175 epochs of REM sleep (4.4739 ± 2.1405; P < 0.0113) (Table 7). The data suggest an increase of sympathetic modulation during W and REM sleep in comparison of synchronized S1 − S4 sleep. 3.7.2. High Altitude (3480 m) The average of the LF:HF ratio during S3 + S4 at 3480 m (3.2109 ± 1.0873) was significantly lower than that ob- served during REM sleep (6.9132 ± 3.6806; P < 0.036835). The data suggest an increase of sympathetic modulation during REM sleep in comparison to synchro- nized S3 + S4 sleep. 3.7.3. Sea Level (122 m) and High Altitude (3480 m) The average of the LF:HF ratio (Table 7) during W was significantly shorter at 3480 m than at 122 m in 1/5 sub- jects (GM: 6.1615 ± 2.4214 at 122 m vs 2.3017 ± 1.3728 at 3480 m; P < 0.0001) and significantly longer at 3480 m than at 122 m in 2/5 subjects [(DC: 4.9300 ± 6.2275 at 122 m vs 7.2334 ± 10.049 at 3480 m; P < 0.0235); (SS: 2.1570 ± 1.4672 at 122 m vs 9.4181 ± 8.1271 at 3480 m; P < 0.0001)]. The average LF:HF ratio during S1 + S2 was significantly longer at 3480 m than at 122 m in 3/5 subjects [(MR: 5.0512 ± 2.3613 at 3480 m vs 3.9624 ± 1.8413 at 122 m; P < 0.0078); (DC: 6.1907 ± 4.3641 at 3480 m vs 1.7193 ± 1.3779 at 122 m; P < 0.0001); (SS: 7.9224 ± 6.7405 at 3480 m vs 2.3667 ± 2.16997 at 122 m; P < 0.0001)] and significantly shorter in 1/5 subjects (GM: 3.9050 ± 1.6255 at 3480 m vs 4.6160 ± 2.0671 at 122 m; P < 0.0503). The average LF:HF ratio was sig- nificantly longer at high altitude than at sea level during S3 + S4 in 3/5 subjects [(MR: 4.6867 ± 1.8516 at 3480 m vs 2.1655 ± 1.2576 at 122 m; P < 0.0043); (DC: 3.7484 ± 1.7785 at 3480 m vs 1.2203 ± 1.4342 at 122 m; P < 0.0001); (SS: 4.0205 ± 2.3448 at 3480 m vs 0.9542 ± 1.0418 at 122 m; P < 0.0001)]. The average LF:HF ratio was significantly longer (P < 0.0102 - 0.0001) during REM sleep at high altitude than at sea level in 4/5 sub- jects [(CS: 4.7380 ± 1.4928 at 3480 m vs 2.8163 ± 0.9832 at 122 m); (MR: 9.8554 ± 4.3032 at 3480 m vs 4.8217 ± 1.5891 at 122 m); (DC: 10.520 ± 6.6211 at 3480 m vs 5.2563 ± 3.7020 at 122 m); (SS: 4.1387 ± 3.9490 at 3480 m vs 2.7194 ± 2.0375 at 122 m)]. At 122 m and at 3480 m there was a decrease in the LF:HF ratio during deepening of NREM sleep, indicating
 I. GRITTI ET AL. Copyright © 2013 SciRes. JBBS 38 Table 6. Averages of the total power in the high frequency (0.04 - 0.15 Hz) range in normalized units (HFRRNU), which is considered as a marker of vagal modulation, in a natural, physiologically defined number of 30-second signal epochs, ac- cording to standard criteria developed by Rechtschaffen and Kales (1968), during the waking period during sleep (W), stages S1 + S2 and S3 + S4 of NREM and REM sleep recorded at 122 m and after 30 - 41 h of acclimatisation at 3480 m in the five subjects.(1) Stages Subjects 122 m 3480 m Epochs Epochs Awakening during sleep N. MeanS.D. N. Mean S.D. DeltaP value C.S 7 23.106.10 35 21.05 9.79 −2.05n.s M.R. 3 14.756.28 3 15.00 6.08 0.25 n.s. G.M. 13 15.154.60 12 33.15 13.39 18.000.0001 D.C. 140 30.8718.75 157 22.29 14.47 −8.58 0.0001 S.S. 27 33.4911.57 64 15.33 11.65 −18.160.0001 No. of epochs 190 No. of subjectsMeanS.E.M.No. of subjectsMean S.E.M. Delta subjects subjects Average 5 23.479.46 5 21.31 11.08 −2.11n.s. S1 + S2 Epochs Epochs N. MeanS.D. N. Mean S.D. DeltaP value C.S 45 29.2915.37 34 32.35 14.89 −1.74n.s M.R. 58 23.179.48 52 18.81 6.71 −4.360.01 G.M. 53 19.977.45 54 21.72 7.39 1.75 n.s. D.C. 302 43.5716.23 322 18.32 9.43 −25.240.001 S.S. 200 36.8617.23 237 16.38 10.36 −20.480.0001 No. of epochs 658 699 No. of subjectsMeanS.E.M.No. of subjectsMean S.E.M. Delta subjects subjects Average 5 30.5713.15 5 21.52 9.76 −9.06 S3 + S4 Epochs Epochs N. MeanS.D. N. Mean S.D. DeltaP value C.S 16 47.9911.84 9 55.07 26.98 7.08 n.s M.R. 11 35.9012.94 6 19.53 8.02 −16.38n.s G.M. 9 26.507.55 7 34.26 9.97 7.76 n.s D.C. 98 53.5316.73 25 24.42 11.04 −29.110.0001 S.S. 92 55.5415.78 59 22.29 8.30 −36.4 0.0001 No. of epochs 226 106 No. of subjectsMeanS.E.M.No. of subjectsMean S.E.M. Delta subjects subjects Average 5 56.91*31.14 5 31.11 12.81 −25.80n.s REM Epochs Epochs N. MeanS.D. N. Mean S.D. DeltaP value C.S 8 26.438.12 5 17.87 4.31 −8.56n.s. M.R. 23 18.505.82 13 10.57 3.76 −7.930.001 G.M. 10 14.316.46 13 16.91 6.18 2.60 n.s. D.C. 62 21.8012.24 30 11.56 6.14 −10.240.0001 S.S. 72 32.1514.57 52 26.81 14.55 −5.34 0.0461 No. of epochs 175 113 No. of subjectsMeanS.E.M.No. of subjectsMean S.E.M. Delta subjects subjects Average 5 22.64** 9.44 5 16.74 6.99 −5.90n.s. Footnotes to Table 6: (1)One-way ANOVA of the averages of the total power in the high frequency (0.04 - 0.15 Hz) range in normalized units (HF-RR-NU) showed significant differences between conditions (DF7,32,39; F 4071; P < 0.0027). Post-hoc analysis demonstrated a significant difference between the average HF-RR-NU during W at 122 m and that during stages S3 + S4 at 122 m (P < 0.05). The averages of HF-RR-NU during W at 3480 m differed significantly from those during stages S3 + S4 at 122 (P < 0.05). The averages of HF-RR-NU during W at 122 m differed significantly from those during stages S3 + S4 at 122 m (P < 0.05). The average of HF-RR-NU during stages S1 + S2 at 122 m differed significantly from that during stages S3 + S4 at 3480 m (P < 0.05). The average of HF-RR-NU during stages S1 + S2 at 3480 m differed significantly from that during stages S3 + S4 at 122 m (P < 0.05). The average of HF-RR-NU during stages S3 + S4 at 122 m differed significantly from that during stages S3 + S4 at 3480 m (P < 0.05). The average of HF-RR-NU during stages S3 + S4 at 122 m differed significantly from that during REM sleep at 122 m (P < 0.05); The average of HF-RR-NU during stages S3 + S4 at 122 m differed significantly from that during stages S3 + S4 at 3480 m (P < 0.05); The average of HF-RR-NU during stages S3 + S4 at 3480 m differed significantly from that during REM sleep at 3480 m (P < 0.05) in all five subjects. *Comparison with Student’s t-test of the average of the HF-RR-NU, in a natural, physiologically defined number of 30-second signal epochs, according to standard criteria developed by Rechtschaffen and Kales (1968), during the waking period during sleep (W) differed significantly from that during stages S3 + S4 of NREM sleep at 122 m in all five subjects (P < 0.0327). **Comparison with Student’s t-test of the average of the HF-RR-NU, in a natural, physiologically defined number of 30-second signal epochs, according to standard criteria developed by Rechtschaffen and Kales (1968), during stages S3 + S4 of NREM sleep differed significantly from that during REM sleep at 122 m in all five subjects (P < 0.01004).
 I. GRITTI ET AL. Copyright © 2013 SciRes. JBBS 39 Table 7. Averages of the ratio of low frequency/high frequency (LF, ms2/HF, ms2) range, which mirrors sympathovagal bal- ance or reflects sympathetic modulation, in a physiologically defined number of 30-second signal epochs, according to stan- dard criteria developed by Rechtschaffen and Kales (1968), during the waking period during sleep (W), stages S1 + S2 and S3 + S4 of NREM sleep and REM sleep at 122 m and after 30 - 41 h of acclimatisation at 3480 m in the five subjects.(1) Stages Subjects 122 m 3480 m Epochs Epochs Awakening during sleep N. Mean S.D. N. Mean S.D. Delta P value C.S 7 3.47 1.4415 35 4.5477 2.2645 1.0777 n.s. M.R. 3 6.513 2.77 3 6.517 3.324 0.004 n.s. G.M. 13 6.1615 2.4214 12 2.3017 1.3728 −3.8598 0.0001 D.C. 140 4.93 6.2275 157 7.2334 10.049 2.3034 0.0235 S.S. 27 2.157 1.4672 64 9.4181 8.1271 7.2611 0.0001 No. of epochs 190 271 No. of subjectsMean S.E.M.No. of subjectsMean S.E.M. Delta subjects subjects Average 5 4.6446 2.8655 5 6.0036 5.0276 1.357 n.s. S1+ S2 Epochs Epochs N. Mean S.D. N. Mean S.D. Delta P value C.S 45 2.9293 1.5299 34 2.5515 1.3856 −0.3778n.s. M.R. 58 3.9624 1.8413 52 5.0512 2.3613 1.0888 0.0078 G.M. 53 4.616 2.0671 54 3.905 1.6255 −0.711 0.0503 D.C. 302 1.7193 1.3779 322 6.1907 4.3641 4.4714 0.0001 S.S. 200 2.3667 2.1697 220 7.9224 6.7405 5.5557 0.0001 No. of epochs 658 683 No. of subjectsMean S.E.M.No. of subjectsMean S.E.M. Delta subjects subjects Average 5 3.1187 1.7972 5 5.1242 3.2954 2.007 n.s. S3+ S4 Epochs Epochs N. Mean S.D. N. Mean S.D. Delta P value C.S 16 1.0919 0.477 9 1.6633 2.2308 0.5714 n.s. M.R. 11 2.1655 1.2576 6 4.6867 1.8516 2.5212 0.0043 G.M. 9 2.9344 1.2127 7 1.9357 0.831 −0.9987n.s. D.C. 98 1.2203 1.4342 25 3.7484 1.7785 2.5281 0.0001 S.S. 92 0.9542 1.0418 59 4.0205 2.3448 3.25 0.0001 No. of epochs 226 No. of subjectsMean S.E.M.No. of subjectsMean S.E.M. Delta subjects subjects Average 5 1.633* 1.0847 5 3.2109 1.8073 1.538 n.s. REM Epochs Epochs N. Mean S.D. N. Mean S.D. Delta P value C.S 8 2.81163 5 4.738 1.4928 1.9217 0.0166 M.R. 23 4.82217 13 9.8554 4.3032 5.0337 0.0001 G.M. 10 6.756 13 5.3138 2.0368 −1.4422n.s. D.C. 62 5.2563 30 10.52 6.6211 5.264 0.0001 S.S. 72 2.7194 52 4.1387 3.949 1.493 0.0102 No. of epochs 175 113 No. of subjectsMean S.E.M.No. of subjectsMean S.E.M. Delta subjects subjects Average 5 4.4739** 5 6.9132*** 3.6806 2.439 n.s. Footnotes to Table 7: (1)One-way ANOVA showed no significant differences in the LF/HF ratio in the different conditions. *Student’s t-test showed that the average of the total power of the LF/HF ratio during the waking state during sleep (W) (190 epochs) at 122 m was generally higher than the average LF/HF ratio during stages S3 + S4 (226 epochs) at the same altitude in the five subjects (P < 0.0110). **Student’s t-test showed that the average of the total power of the LF/HF ratio during stages S3 + S4 in a total of 226 epochs of 30 seconds at low altitude was significantly lower than that in 175 epochs of REM sleep at 122 m in the five subjects (P < 0.011335). ***Student’s t-test showed that the average of the total power of the LF/HF ratio during stages S3 + S4 (106 epochs) at 3480 m was significantly lower than that during REM sleep (113 epochs) at high altitude in the five subjects (P < 0.036835).
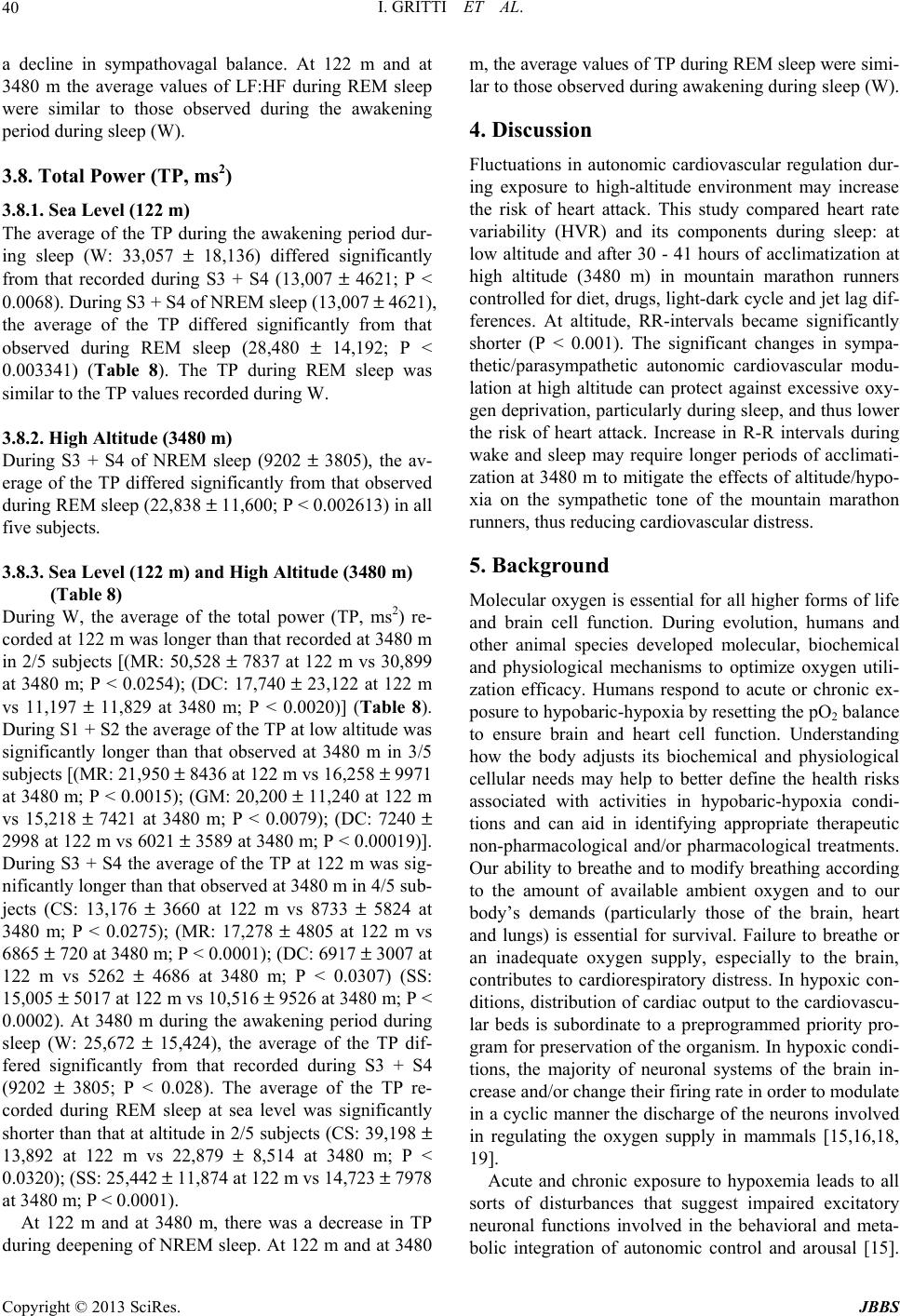 I. GRITTI ET AL. Copyright © 2013 SciRes. JBBS 40 a decline in sympathovagal balance. At 122 m and at 3480 m the average values of LF:HF during REM sleep were similar to those observed during the awakening period during sleep (W). 3.8. Total Power (TP, ms2) 3.8.1. Sea Level (122 m) The average of the TP during the awakening period dur- ing sleep (W: 33,057 ± 18,136) differed significantly from that recorded during S3 + S4 (13,007 ± 4621; P < 0.0068). During S3 + S4 of NREM sleep (13,007 ± 4621), the average of the TP differed significantly from that observed during REM sleep (28,480 ± 14,192; P < 0.003341) (Table 8). The TP during REM sleep was similar to the TP values recorded during W. 3.8.2. High Altitude (3480 m) During S3 + S4 of NREM sleep (9202 ± 3805), the av- erage of the TP differed significantly from that observed during REM sleep (22,838 ± 11,600; P < 0.002613) in all five subjects. 3.8.3. Sea Level (122 m) and High Altitude (3480 m) (Table 8) During W, the average of the total power (TP, ms2) re- corded at 122 m was longer than that recorded at 3480 m in 2/5 subjects [(MR: 50,528 ± 7837 at 122 m vs 30,899 at 3480 m; P < 0.0254); (DC: 17,740 ± 23,122 at 122 m vs 11,197 ± 11,829 at 3480 m; P < 0.0020)] (Table 8). During S1 + S2 the average of the TP at low altitude was significantly longer than that observed at 3480 m in 3/5 subjects [(MR: 21,950 ± 8436 at 122 m vs 16,258 ± 9971 at 3480 m; P < 0.0015); (GM: 20,200 ± 11,240 at 122 m vs 15,218 ± 7421 at 3480 m; P < 0.0079); (DC: 7240 ± 2998 at 122 m vs 6021 ± 3589 at 3480 m; P < 0.00019)]. During S3 + S4 the average of the TP at 122 m was sig- nificantly longer than that observed at 3480 m in 4/5 sub- jects (CS: 13,176 ± 3660 at 122 m vs 8733 ± 5824 at 3480 m; P < 0.0275); (MR: 17,278 ± 4805 at 122 m vs 6865 ± 720 at 3480 m; P < 0.0001); (DC: 6917 ± 3007 at 122 m vs 5262 ± 4686 at 3480 m; P < 0.0307) (SS: 15,005 ± 5017 at 122 m vs 10,516 ± 9526 at 3480 m; P < 0.0002). At 3480 m during the awakening period during sleep (W: 25,672 ± 15,424), the average of the TP dif- fered significantly from that recorded during S3 + S4 (9202 ± 3805; P < 0.028). The average of the TP re- corded during REM sleep at sea level was significantly shorter than that at altitude in 2/5 subjects (CS: 39,198 ± 13,892 at 122 m vs 22,879 ± 8,514 at 3480 m; P < 0.0320); (SS: 25,442 ± 11,874 at 122 m vs 14,723 ± 7978 at 3480 m; P < 0.0001). At 122 m and at 3480 m, there was a decrease in TP during deepening of NREM sleep. At 122 m and at 3480 m, the average values of TP during REM sleep were simi- lar to those observed during awakening during sleep (W). 4. Discussion Fluctuations in autonomic cardiovascular regulation dur- ing exposure to high-altitude environment may increase the risk of heart attack. This study compared heart rate variability (HVR) and its components during sleep: at low altitude and after 30 - 41 hours of acclimatization at high altitude (3480 m) in mountain marathon runners controlled for diet, drugs, light-dark cycle and jet lag dif- ferences. At altitude, RR-intervals became significantly shorter (P < 0.001). The significant changes in sympa- thetic/parasympathetic autonomic cardiovascular modu- lation at high altitude can protect against excessive oxy- gen deprivation, particularly during sleep, and thus lower the risk of heart attack. Increase in R-R intervals during wake and sleep may require longer periods of acclimati- zation at 3480 m to mitigate the effects of altitude/hypo- xia on the sympathetic tone of the mountain marathon runners, thus reducing cardiovascular distress. 5. Background Molecular oxygen is essential for all higher forms of life and brain cell function. During evolution, humans and other animal species developed molecular, biochemical and physiological mechanisms to optimize oxygen utili- zation efficacy. Humans respond to acute or chronic ex- posure to hypobaric-hypoxia by resetting the pO2 balance to ensure brain and heart cell function. Understanding how the body adjusts its biochemical and physiological cellular needs may help to better define the health risks associated with activities in hypobaric-hypoxia condi- tions and can aid in identifying appropriate therapeutic non-pharmacological and/or pharmacological treatments. Our ability to breathe and to modify breathing according to the amount of available ambient oxygen and to our body’s demands (particularly those of the brain, heart and lungs) is essential for survival. Failure to breathe or an inadequate oxygen supply, especially to the brain, contributes to cardiorespiratory distress. In hypoxic con- ditions, distribution of cardiac output to the cardiovascu- lar beds is subordinate to a preprogrammed priority pro- gram for preservation of the organism. In hypoxic condi- tions, the majority of neuronal systems of the brain in- crease and/or change their firing rate in order to modulate in a cyclic manner the discharge of the neurons involved in regulating the oxygen supply in mammals [15,16,18, 19]. Acute and chronic exposure to hypoxemia leads to all sorts of disturbances that suggest impaired excitatory neuronal functions involved in the behavioral and meta- bolic integration of autonomic control and arousal [15].
 I. GRITTI ET AL. Copyright © 2013 SciRes. JBBS 41 Table 8. Averages of the total power (TP, ms2) in a physiologically defined number of 30-second EEG signal epochs, accord- ing to standard criteria developed by Rechtschaffen and Kales (1968), during the waking state during sleep (W), stages S1 + S2 and S3 + S4 of NREM sleep and REM sleep at 122 m and after 30 - 41 h of acclimatisation at 3480 m in the five subjects. Stages Subjects 122 m 3480 m Epochs Epochs Awakening during sleep N. Mean S.D. N. Mean S.D. Delta P value C.S 7 33,449 24,25735 25,486 16,753 −7963n.s. M.R. 3 50,528 7837 3 30,899 5845 −19,629 0.0254 G.M. 13 29,277 9809 12 28,573 22,336 −704 n.s. D.C. 140 17,740 23,122157 11,197 11,829 −65430.002 S.S. 27 34,293 25,65564 32,203 20,358 −2090n.s. No. of epochs 190 271 No. of subjectsMean S.E.M.No. of subjectsMean S.E.M. Delta subjects subjects Average 5 33,057 18,1365 25,672 15,424 −7385n.s. S1+ S2 Epochs Epochs N. Mean S.D. N. Mean S.D. Delta P value C.S 45 29,292 16,38034 22,533 13,712 −6758 M.R. 58 21,950 8436 52 16,258 9971 −5692n.s. G.M. 53 20,200 11,24054 15,218 7421 −4982 0.0015 D.C. 302 7420 2998 322 6021 3589 −1398 0.0079 S.S. 200 25,316 18,423237 29,006 16,917 3690 0.0001 No. of epochs 658 683 0.0297 No. of subjectsMean S.E.M.No. of subjectsMean S.E.M. Delta subjects subjects Average 5 20,836 11,4955 17,807 10,322 −3028n.s S3+ S4 Epochs Epochs N. Mean S.D. N. Mean S.D. Delta P value C.S 16 13,176 3660 9 8733 5824 −4443 0.0275 M.R. 11 17,278 4805 6 6865 720 −10,412 0.0001 G.M. 9 12,659 6614 7 11,634 6801 −1025n.s. D.C. 98 6917 3007 25 5262 4686 −1655 0.0307 S.S. 92 15,005 5017 59 10,516 9526 −4489 0.0002 No. of epochs 226 106 No. of subjectsMean S.E.M.No. of subjectsMean S.E.M. Delta subjects subjects Average 5 13,0007*4621 5 9202** 3805 −3798n.s. REM Epochs Epochs N. Mean S.D. N. Mean S.D. Delta P value C.S 8 39,198 13,8925 29,459 13,908 −938 n.s. M.R. 23 33,035 14,90713 22,879 8514 −10,1560.032 G.M. 10 23,552 6159 13 29,734 9673 6182 n.s. D.C. 62 21,174 24,12830 17,396 17,929 −3778n.s. S.S. 72 25,442 11,87452 14,723 7978 10,7190.0001 No. of epochs 175 113 No. of subjectsMean S.E.M.No. of subjectsMean S.E.M. Delta subjects subjects Average 5 28,480***14,1925 22,838**** 116,000 −5642n.s. Footnotes to Table 8: (1)One-way ANOVA showed a significant difference in the average of the total power (TP) between conditions (DF 7,32,39; F = 5433; P = 0.0004). Post-hoc Fisher analysis revealed a significant difference between the average of the TP recorded at 122 m during waking (W) and that recorded at 122 m during stages S1 + S2 (P < 0.05)and during stages S3 + S4 (P < 0.05). Post-hoc Fisher analysis revealed a significant difference between the average of the TP recorded at 122 m during waking (W) and that at 3480 m during stages S1 + S2 (P < 0.05) and stages S3 + S4 (P < 0.05). Post-hoc Fisher analysis revealed a significant difference between the average of the TP recorded at 122 m during waking (W) and that at 3480 m during REM sleep (P < 0.05). Post-hoc Fisher analysis revealed a significant difference between the average of the TP recorded at 3480 m during stages S3 + S4 and that at 122 m during stages S3 + S4 (P < 0.05). Post-hoc Fisher analysis revealed a significant difference between the average of the TP recorded at 122 m during stages S1 + S2 and that at 3480 m dur- ing REM sleep (P < 0.05). Post-hoc Fisher analysis revealed a significant difference between the average of the TP recorded at 3480 m during stages S1 + S2 and that at 122 m during REM sleep (P < 0.05). Post-hoc Fisher analysis revealed a significant difference between the average of the TP recorded at 122 m during stages S3 + S4 and that at 122 m during REM sleep (P < 0.05). Post-hoc Fisher revealed a significant difference between the average of the TP recorded at 3480 m during stages S3 + S4 and that at 122 m during REM sleep (P < 0.05). Post-hoc Fisher analysis revealed a significant difference between the average of the TP recorded at 3480 m during stages S3 + S4 and that at 3480 m during REM sleep (P < 0.05) in the five subjects. *Post-hoc analysis with Student’s t-test showed a significant difference (P < 0.05) between the average of the total power (TP, ms2), in a physiologically defined number of 30-second signal epochs, according to standard criteria developed by Rechtschaffen and Kales (1968), recorded during the waking state during sleep (W) (190 epochs) and that during stages S3 + S4 (226 epochs) at 122 m (P < 0.0068) in the five subjects. **Post-hoc analysis with Student’s t-test showed a significant difference (P < 0.028) between the average of the total power (TP, ms2), in a physiologically defined number of 30-second signal epochs, according to standard criteria developed by Rechtschaffen and Kales (1968), recorded during the waking state during sleep (W) (271 epochs) and that during stages S3 + S4 (106 epochs) at 3480 m in the five subjects. ***Post-hoc analysis with Student’s t-test showed a significant difference (P < 0.003341) between the average of the total power (TP, ms2), in a physio- logically defined number of 30-second signal epochs, according to standard criteria developed by Rechtschaffen and Kales (1968), recorded during stages S3 + S4 (226 epochs) and that during REM sleep (175 epochs) at 122 m in the five subjects. ****Post-hoc analysis with Student’s t-test showed a significant difference (P < 0.002613) between the average of the total power (TP, ms2), in a physiologically defined number of 30-second signal epochs, according to standard criteria devel- oped by Rechtschaffen and Kales (1968), recorded during stages S3 + S4 (106 epochs) and that during REM sleep (113 epochs) at 3480 m in the five subjects.
 I. GRITTI ET AL. Copyright © 2013 SciRes. JBBS 42 Research into the causes of cardiovascular mortality, ex- perimental evidence for a predisposition to fatal arrhyth- mias, signs of tonic or phasic increased sympathetic ac- tivity, and reduced parasympathetic-cholinergic-vagal ac- tivity has advanced efforts for the development of quan- titative markers of heart rate variability (HRV) (Task Force, [1]). 5.1. Heart Rate Variability during Wake and Sleep HRV has been established as a non-invasive tool to study cardiac autonomic activity and proposed as a predictor for evaluating the increased risk of cardiac death. Inter- actions of changes in cardiac autonomic nervous modu- lation in various types of subjects are under study by the Task Force [1]. An overnight declining trend of HRV has been found to increase during sleep [14]. Otzenberger and co-workers [14] demonstrated that overnight profiles of the R-R intervals are related to changes in the sleep EEG mean frequency sign, which reflects the depth of sleep. During attentive-to-quiet waking and from the lighter to the deeper phases of sleep, HRV has been shown to be affected by vagal/sympathetic modulation and control. 5.2. Heart Rate Variability and Mountain Marathon Runners Past studies showed that the high-altitude endurance per- formance of mountain marathoners is appreciably re- duced at 5200 m. Based on their clinical characteristics, mountain marathoners can be considered as a physio- logical model for studying cardiovascular alterations, of long-lasting stay and exercise at high altitude. Over the last 12 years, the time course of cardiovascular changes in the mountain marathon runners has been assessed be- fore, during, and after the end of races at altitude. In sea- level native mountain marathoners, long-lasting training —from sea level to altitude—can lower the HR, during rest and exercise, associated with a decrease in sympa- thetic modulation and a rise in parasympathetic activity [20]. As in normal subjects, so too in well-trained moun- tain marathoners cardiovascular modifications can change continuously over a 24-h period during acclimatization and the nocturnal sleep-wake-cycle at low and high alti- tudes. In mountain marathoners, just as in normal sub- jects, HRV alterations at altitude may include altered resting HR due to changes in the vagal and sympathetic components, besides the appearance of sinus arrhythmia during waking and periodically during nocturnal S1 + S2 NREM sleep and REM sleep breathing. As suggested by the Task Force [1], the present study data were obtained during sleep and as such may add valuable insights for research into HRV. With this study we also wanted to determine whether 20 years of training between 122 m and 5200 m altitude could have exerted, in addition to the changes reported elsewhere [9,12], a high impact on the autonomic/cardiovascular systems of the mountain marathon runners during the nocturnal sleep- wake cycle at low and at high altitudes. Our study documents that, in mountain marathoners, the nocturnal sleep-wake cycle at 122 m is highly influ- ence by autonomic parasympathetic activity and by in- creased autonomic parasympathetic activity during the deepening of NREM sleep. The results also show a phy- siological decrease in parasympathetic activity versus an increase in sympathetic activity during all phases of the nocturnal sleep-wake cycle at 3480 m. Surprisingly, our study also documents that the nocturnal sleep-wake cycle at 3480 m is still characterized by an increase in auto- nomic parasympathetic activity during light S1 + S2 NREM sleep. At both altitudes, the trend of the balance of parasympathetic/sympathetic activity recorded during REM sleep is similar to that recorded during the awak- ening state (W). 5.3. R-R Intervals (ms) 5.3.1. Sea Level (122 m) The averages of the R-R intervals recorded at 122 m did not change significantly between nocturnal awakenings during sleep and sleep stages (R-R interval range, 1200 - 1300 ms). The average R-R interval at 122 m, during the awaking state of sleep, was similar to that recorded dur- ing the wake state in 12 trained endurance athletes who performed a minimum of 3 h of aerobic activity per week [21]. 5.3.2. High Altitude (3480 m) The majority of the ECG alterations in the five mountain runners, during sleep at an altitude of 3480 m and at a barometric pressure (PB) of 495 mm Hg, and between 30 and 41 h of acclimatization were: signs of sinus arrhyth- mia during the early stages (S1 + S2) of NREM sleep and during REM sleep. At 3480 m, the average of the R-R intervals we recorded during S1 + S2 of NREM sleep were significantly longer than those observed during the awaking period (W) during sleep. The longest average R-R intervals were recorded during S1 + S2 at 3480 m. These results suggest that, at altitude, there was still a significant elevation of parasympathetic tone during S1 + S2 of slow-wave sleep versus the waking state during sleep at 3480 m. 5.3.3. Sea Level (122 m) and High Altitude (3480 m) There was a nonpathological, significant reduction in the R-R intervals during the awakening period during sleep (W), S1 + S2, S3 + S4 of NREM and REM sleep stages, and after 30 - 41 h of acclimatization to an altitude of
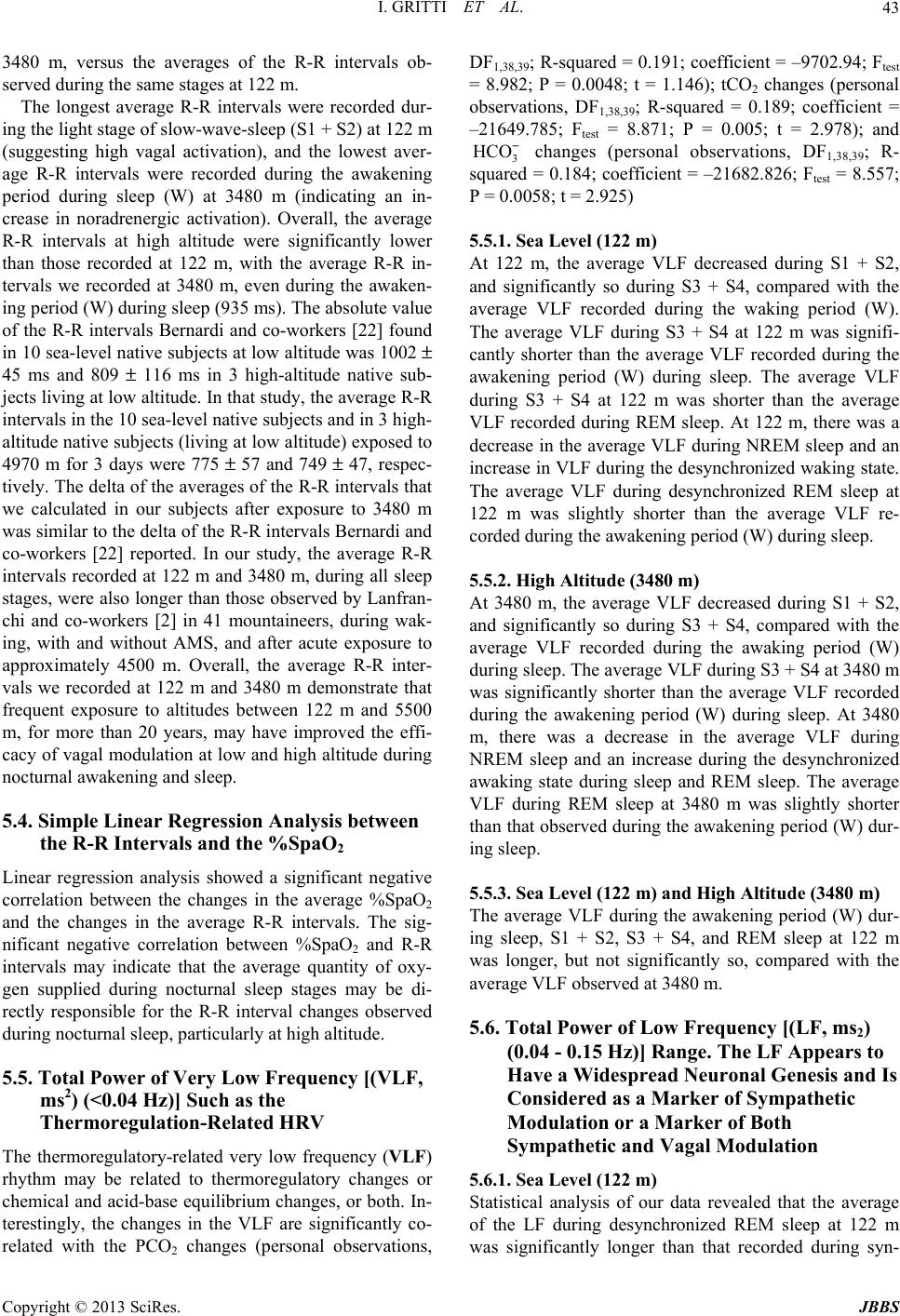 I. GRITTI ET AL. Copyright © 2013 SciRes. JBBS 43 3480 m, versus the averages of the R-R intervals ob- served during the same stages at 122 m. The longest average R-R intervals were recorded dur- ing the light stage of slow-wave-sleep (S1 + S2) at 122 m (suggesting high vagal activation), and the lowest aver- age R-R intervals were recorded during the awakening period during sleep (W) at 3480 m (indicating an in- crease in noradrenergic activation). Overall, the average R-R intervals at high altitude were significantly lower than those recorded at 122 m, with the average R-R in- tervals we recorded at 3480 m, even during the awaken- ing period (W) during sleep (935 ms). The absolute value of the R-R intervals Bernardi and co-workers [22] found in 10 sea-level native subjects at low altitude was 1002 ± 45 ms and 809 ± 116 ms in 3 high-altitude native sub- jects living at low altitude. In that study, the average R-R intervals in the 10 sea-level native subjects and in 3 high- altitude native subjects (living at low altitude) exposed to 4970 m for 3 days were 775 ± 57 and 749 ± 47, respec- tively. The delta of the averages of the R-R intervals that we calculated in our subjects after exposure to 3480 m was similar to the delta of the R-R intervals Bernardi and co-workers [22] reported. In our study, the average R-R intervals recorded at 122 m and 3480 m, during all sleep stages, were also longer than those observed by Lanfran- chi and co-workers [2] in 41 mountaineers, during wak- ing, with and without AMS, and after acute exposure to approximately 4500 m. Overall, the average R-R inter- vals we recorded at 122 m and 3480 m demonstrate that frequent exposure to altitudes between 122 m and 5500 m, for more than 20 years, may have improved the effi- cacy of vagal modulation at low and high altitude during nocturnal awakening and sleep. 5.4. Simple Linear Regression Analysis between the R-R Intervals and the %SpaO2 Linear regression analysis showed a significant negative correlation between the changes in the average %SpaO2 and the changes in the average R-R intervals. The sig- nificant negative correlation between %SpaO2 and R-R intervals may indicate that the average quantity of oxy- gen supplied during nocturnal sleep stages may be di- rectly responsible for the R-R interval changes observed during nocturnal sleep, particularly at high altitude. 5.5. Total Power of Very Low Frequency [(VLF, ms2) (<0.04 Hz)] Such as the Thermoregulation-Related HRV The thermoregulatory-related very low frequency (VLF) rhythm may be related to thermoregulatory changes or chemical and acid-base equilibrium changes, or both. In- terestingly, the changes in the VLF are significantly co- related with the PCO2 changes (personal observations, DF1,38,39; R-squared = 0.191; coefficient = –9702.94; Ftest = 8.982; P = 0.0048; t = 1.146); tCO2 changes (personal observations, DF1,38,39; R-squared = 0.189; coefficient = –21649.785; Ftest = 8.871; P = 0.005; t = 2.978); and 3 HCO − changes (personal observations, DF1,38,39; R- squared = 0.184; coefficient = –21682.826; Ftest = 8.557; P = 0.0058; t = 2.925) 5.5.1. Sea Level (122 m) At 122 m, the average VLF decreased during S1 + S2, and significantly so during S3 + S4, compared with the average VLF recorded during the waking period (W). The average VLF during S3 + S4 at 122 m was signifi- cantly shorter than the average VLF recorded during the awakening period (W) during sleep. The average VLF during S3 + S4 at 122 m was shorter than the average VLF recorded during REM sleep. At 122 m, there was a decrease in the average VLF during NREM sleep and an increase in VLF during the desynchronized waking state. The average VLF during desynchronized REM sleep at 122 m was slightly shorter than the average VLF re- corded during the awakening period (W) during sleep. 5.5.2. High Altitude (3480 m) At 3480 m, the average VLF decreased during S1 + S2, and significantly so during S3 + S4, compared with the average VLF recorded during the awaking period (W) during sleep. The average VLF during S3 + S4 at 3480 m was significantly shorter than the average VLF recorded during the awakening period (W) during sleep. At 3480 m, there was a decrease in the average VLF during NREM sleep and an increase during the desynchronized awaking state during sleep and REM sleep. The average VLF during REM sleep at 3480 m was slightly shorter than that observed during the awakening period (W) dur- ing sleep. 5.5.3. Sea Level (122 m) and High Altitude (3480 m) The average VLF during the awakening period (W) dur- ing sleep, S1 + S2, S3 + S4, and REM sleep at 122 m was longer, but not significantly so, compared with the average VLF observed at 3480 m. 5.6. Total Power of Low Frequency [(LF, ms2) (0.04 - 0.15 Hz)] Range. The LF Appears to Have a Widespread Neuronal Genesis and Is Considered as a Marker of Sympathetic Modulation or a Marker of Both Sympathetic and Vagal Modulation 5.6.1. Sea Level (122 m) Statistical analysis of our data revealed that the average of the LF during desynchronized REM sleep at 122 m was significantly longer than that recorded during syn-
 I. GRITTI ET AL. Copyright © 2013 SciRes. JBBS 44 chronized S3 + S4 sleep. These data demonstrate an in- crease in parasympathetic tone during REM sleep. 5.6.2. High Altitude (3480 m) In altitude there was a significant increase in sympathetic tone throughout the awakening period (W) during sleep, the S1 + S2, and the REM sleep. The sympathetic tone decreased during the deep phase of slow wave sleep (S3 + S4). 5.6.3. Sea Level (122 m) and High Altitude (3480 m) No significant changes were found between the average LF recorded at 122 m and that recorded at 3480 m. How- ever, in some subjects, a significant decrease emerged between the average of LF recorded during the awaken- ing period (W) during sleep, S1 + S2, S3 + S4, and REM sleep at 122 m versus the average of LF recorded at 3480 m. The average LF recorded in all 5 mountain marathon- ers and in all 4 stages of desynchronization and synchro- nization at 122 m and at 3480 m (Table 3) were longer than the average LF Ako and co-workers [3] observed in 7 subjects during sleep at sea level. We also found a decrease in the average LF during S3 + S4 at 122 m and 3480 m compared with the average LF recorded during S1 + S2. The average LF recorded in our study during the desynchronized REM sleep state, at 122 m and 3480 m, returned to similar values we recorded during the desynchronized awakening period (W) during sleep. A comparison of LF indices in the different sleep stages recorded at 122 m and at 3480 m revealed a de- crease in the LF from the waking to the deep stages of sleep. The average LF during REM sleep was similar to the average LF recorded during waking. Like those of Ako and co-workers [3], our data suggest that sympa- thetic nervous activity decreases as sleep deepens and concomitantly increases during desynchronization of the awakening period (W) during sleep and even more so during desynchronization during REM sleep. In our study, the average LF, a marker of sympathetic modulation, during the awakening period (W) during sleep, S1 + S2, S3 + S4, and REM sleep at 122 m and at 3480 m was lower. 5.7. LFRRNU The aim of this study was also to verify whether auto- nomic variables at low altitude could predict prodromic signs of AMS when subjects were exposed to high alti- tude or whether subjects experienced AMS at high alti- tude. Autonomic cardiovascular function was also ex- plored by measuring widespread neuronal genesis rhythm low frequency in normalized unit (LFRRNU) to study sympathetic modulation. Lanfranchi and co-workers [2] reported a substantial increase in the LFRRNU component at altitude, suggest- ing an increase in sympathetic modulation in response to hypoxia at 4500 m. During exposure to high altitude, besides shorter R-R intervals, we also found a relative, though not significant, increase in the LFRRNU compo- nent. We agree with Lanfranchi and co-workers [2] that this increase in the LFRRNU component may reflect an in- crease in the sympathetic modulation of HR. During ex- posure to 3480 m, R-R interval variability decreased as the LF component increased, suggesting an increase in sympathetic modulation in response to hypobaric-hypo- xia. The average LFRRNU Lanfranchi and co-workers [2] recorded in 24 subjects without AMS after exposure to approximately 4500 m during waking was similar to the average we recorded in our subjects during the awaken- ing period (W) during sleep at 3480 m (73 vs 75, respec- tively). LFRRNU has been said to be a quantitative marker of the cardiac vigil (Vanderwalle et al. [23]) and sympa- thetic outflow of the autonomic nervous system. By means of spectral analysis of subjects in supine position, the Task Force [1] calculated an average LFRRNU of 54, which is fairly similar to the average values in 4/5 of our subjects: 47 in CS, 45 in DC, and 39 in SS in supine po- sition during S3 + S4 at 122 m. Overall, the average LFRRNU reported by the Task Force [1] is somewhat lower than our data (54 vs 39 - 89, respectively). From a steady-state HRV analysis, Murrel and co- workers [24] found an average LFRRNU of 77 in the standing position and premarathon conditions, an average between 72 and 92 immediately after a marathon, and an average of 73 in the standing position at 48 h postmara- thon. These data appear similar to the data we recorded at 122 m and 3480 m during the awakening, S1 + S2, S3 + S4, and REM stages of sleep. Specifically, at 122 m there was a decrease in LFRRNU as sleep depth increased; during the desynchronized phase of sleep (the awakening state), the LFRRNU reached the values observed during REM sleep. At 3480 m there was a decrease in LFRRNU as sleep deepened; during the de- synchronized phase of sleep (the awakening state) the LFRRNU reached the values recorded during the REM phases at 3480 m. 5.8. High Frequency Efferent vagal activity is a major contributor to the high frequency (HF) component (Task Force, [1]) which pri- marily reflects respiration-driven vigil modulation of the sinus rhythm. Other factors such as voluntary controlled respiration, cold stimulation of the face or rotational mo- tor stimuli (see above) can occur physiologically during
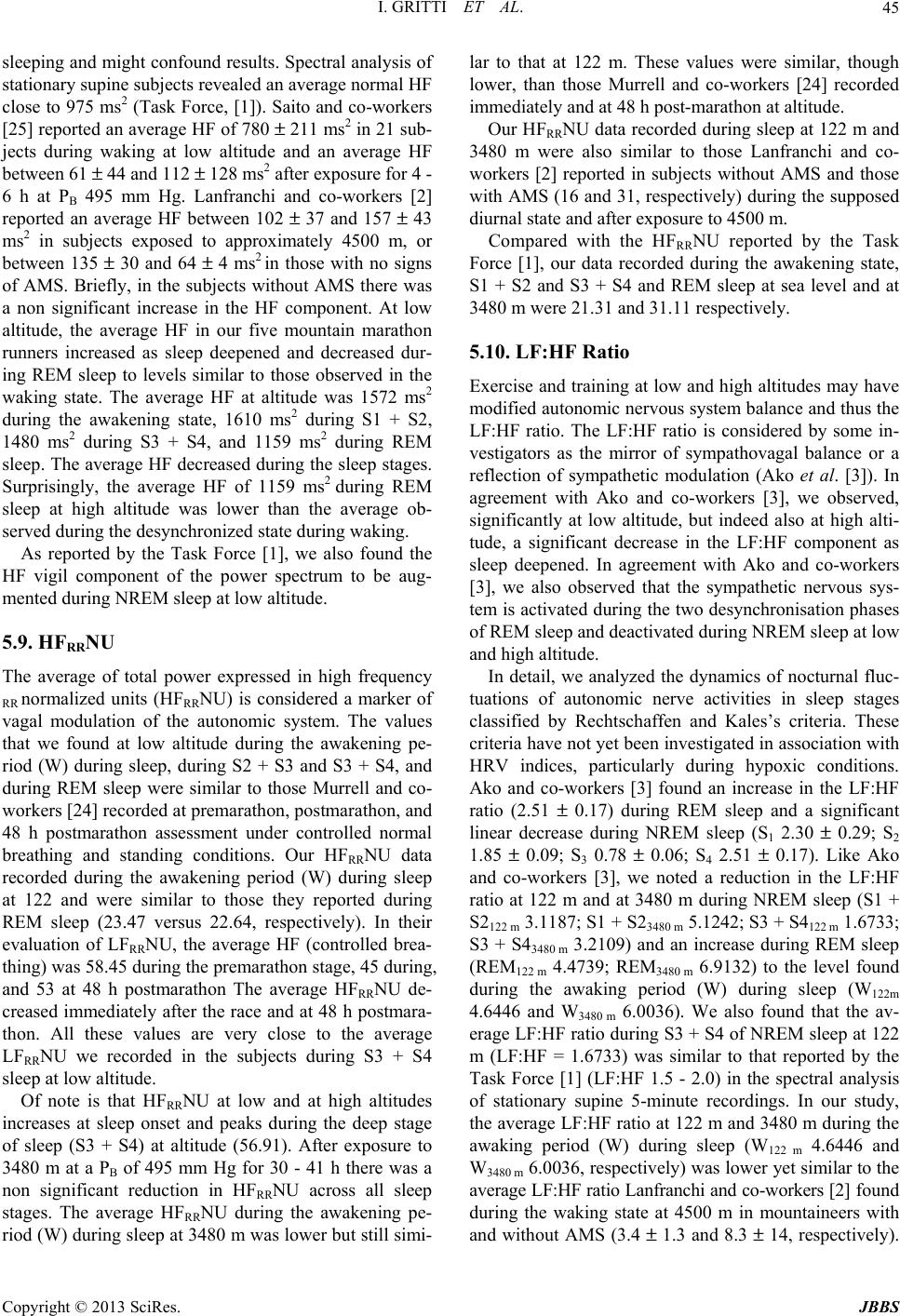 I. GRITTI ET AL. Copyright © 2013 SciRes. JBBS 45 sleeping and might confound results. Spectral analysis of stationary supine subjects revealed an average normal HF close to 975 ms2 (Task Force, [1]). Saito and co-workers [25] reported an average HF of 780 ± 211 ms2 in 21 sub- jects during waking at low altitude and an average HF between 61 ± 44 and 112 ± 128 ms2 after exposure for 4 - 6 h at PB 495 mm Hg. Lanfranchi and co-workers [2] reported an average HF between 102 ± 37 and 157 ± 43 ms2 in subjects exposed to approximately 4500 m, or between 135 ± 30 and 64 ± 4 ms2 in those with no signs of AMS. Briefly, in the subjects without AMS there was a non significant increase in the HF component. At low altitude, the average HF in our five mountain marathon runners increased as sleep deepened and decreased dur- ing REM sleep to levels similar to those observed in the waking state. The average HF at altitude was 1572 ms2 during the awakening state, 1610 ms2 during S1 + S2, 1480 ms2 during S3 + S4, and 1159 ms2 during REM sleep. The average HF decreased during the sleep stages. Surprisingly, the average HF of 1159 ms2 during REM sleep at high altitude was lower than the average ob- served during the desynchronized state during waking. As reported by the Task Force [1], we also found the HF vigil component of the power spectrum to be aug- mented during NREM sleep at low altitude. 5.9. HFRRNU The average of total power expressed in high frequency RR normalized units (HFRRNU) is considered a marker of vagal modulation of the autonomic system. The values that we found at low altitude during the awakening pe- riod (W) during sleep, during S2 + S3 and S3 + S4, and during REM sleep were similar to those Murrell and co- workers [24] recorded at premarathon, postmarathon, and 48 h postmarathon assessment under controlled normal breathing and standing conditions. Our HFRRNU data recorded during the awakening period (W) during sleep at 122 and were similar to those they reported during REM sleep (23.47 versus 22.64, respectively). In their evaluation of LFRRNU, the average HF (controlled brea- thing) was 58.45 during the premarathon stage, 45 during, and 53 at 48 h postmarathon The average HFRRNU de- creased immediately after the race and at 48 h postmara- thon. All these values are very close to the average LFRRNU we recorded in the subjects during S3 + S4 sleep at low altitude. Of note is that HFRRNU at low and at high altitudes increases at sleep onset and peaks during the deep stage of sleep (S3 + S4) at altitude (56.91). After exposure to 3480 m at a PB of 495 mm Hg for 30 - 41 h there was a non significant reduction in HFRRNU across all sleep stages. The average HFRRNU during the awakening pe- riod (W) during sleep at 3480 m was lower but still simi- lar to that at 122 m. These values were similar, though lower, than those Murrell and co-workers [24] recorded immediately and at 48 h post-marathon at altitude. Our HFRRNU data recorded during sleep at 122 m and 3480 m were also similar to those Lanfranchi and co- workers [2] reported in subjects without AMS and those with AMS (16 and 31, respectively) during the supposed diurnal state and after exposure to 4500 m. Compared with the HFRRNU reported by the Task Force [1], our data recorded during the awakening state, S1 + S2 and S3 + S4 and REM sleep at sea level and at 3480 m were 21.31 and 31.11 respectively. 5.10. LF:HF Ratio Exercise and training at low and high altitudes may have modified autonomic nervous system balance and thus the LF:HF ratio. The LF:HF ratio is considered by some in- vestigators as the mirror of sympathovagal balance or a reflection of sympathetic modulation (Ako et al. [3]). In agreement with Ako and co-workers [3], we observed, significantly at low altitude, but indeed also at high alti- tude, a significant decrease in the LF:HF component as sleep deepened. In agreement with Ako and co-workers [3], we also observed that the sympathetic nervous sys- tem is activated during the two desynchronisation phases of REM sleep and deactivated during NREM sleep at low and high altitude. In detail, we analyzed the dynamics of nocturnal fluc- tuations of autonomic nerve activities in sleep stages classified by Rechtschaffen and Kales’s criteria. These criteria have not yet been investigated in association with HRV indices, particularly during hypoxic conditions. Ako and co-workers [3] found an increase in the LF:HF ratio (2.51 ± 0.17) during REM sleep and a significant linear decrease during NREM sleep (S1 2.30 ± 0.29; S2 1.85 ± 0.09; S3 0.78 ± 0.06; S4 2.51 ± 0.17). Like Ako and co-workers [3], we noted a reduction in the LF:HF ratio at 122 m and at 3480 m during NREM sleep (S1 + S2122 m 3.1187; S1 + S23480 m 5.1242; S3 + S4122 m 1.6733; S3 + S43480 m 3.2109) and an increase during REM sleep (REM122 m 4.4739; REM3480 m 6.9132) to the level found during the awaking period (W) during sleep (W122m 4.6446 and W3480 m 6.0036). We also found that the av- erage LF:HF ratio during S3 + S4 of NREM sleep at 122 m (LF:HF = 1.6733) was similar to that reported by the Task Force [1] (LF:HF 1.5 - 2.0) in the spectral analysis of stationary supine 5-minute recordings. In our study, the average LF:HF ratio at 122 m and 3480 m during the awaking period (W) during sleep (W122 m 4.6446 and W3480 m 6.0036, respectively) was lower yet similar to the average LF:HF ratio Lanfranchi and co-workers [2] found during the waking state at 4500 m in mountaineers with and without AMS (3.4 ± 1.3 and 8.3 ± 14, respectively).
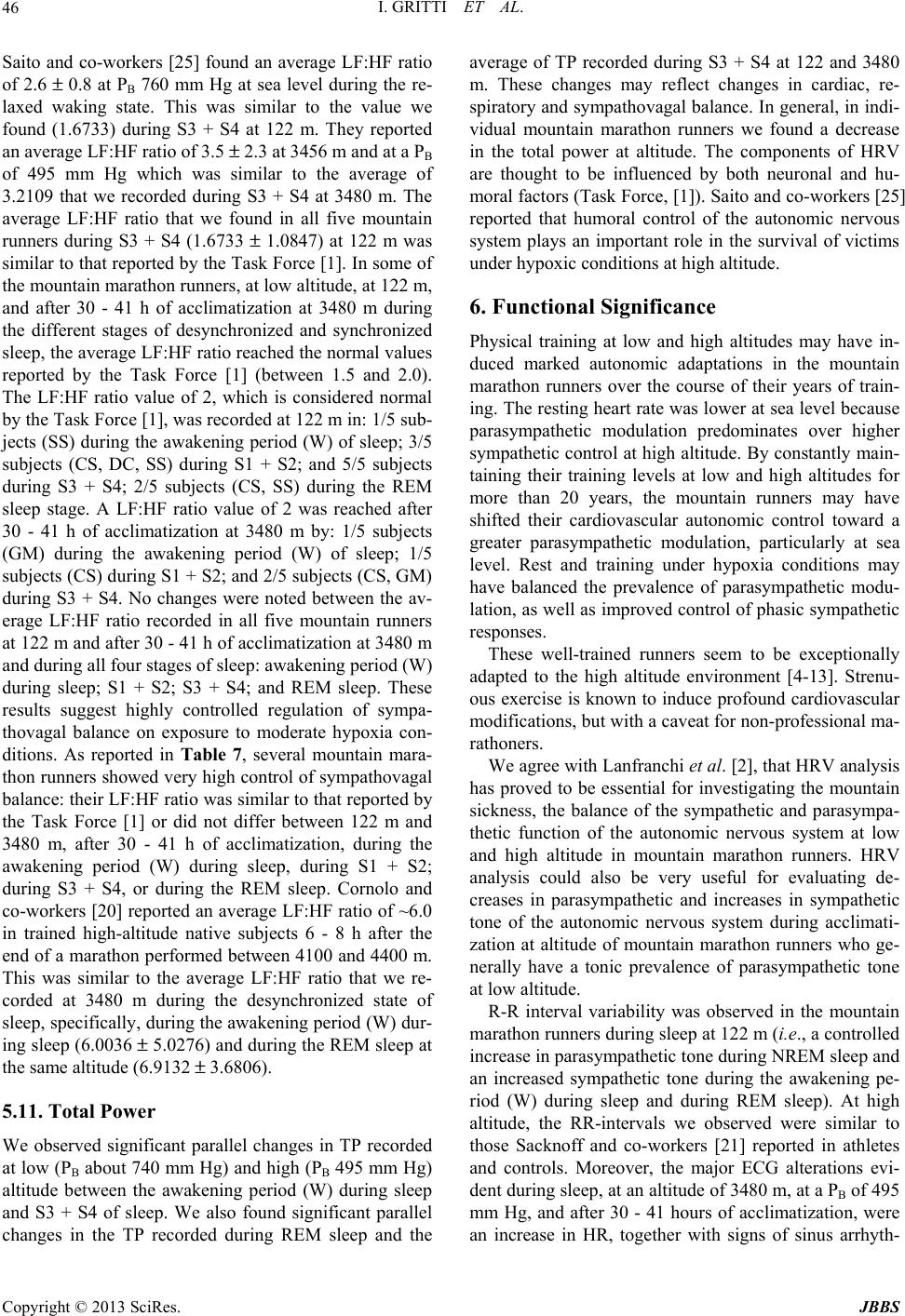 I. GRITTI ET AL. Copyright © 2013 SciRes. JBBS 46 Saito and co-workers [25] found an average LF:HF ratio of 2.6 ± 0.8 at PB 760 mm Hg at sea level during the re- laxed waking state. This was similar to the value we found (1.6733) during S3 + S4 at 122 m. They reported an average LF:HF ratio of 3.5 ± 2.3 at 3456 m and at a PB of 495 mm Hg which was similar to the average of 3.2109 that we recorded during S3 + S4 at 3480 m. The average LF:HF ratio that we found in all five mountain runners during S3 + S4 (1.6733 ± 1.0847) at 122 m was similar to that reported by the Task Force [1]. In some of the mountain marathon runners, at low altitude, at 122 m, and after 30 - 41 h of acclimatization at 3480 m during the different stages of desynchronized and synchronized sleep, the average LF:HF ratio reached the normal values reported by the Task Force [1] (between 1.5 and 2.0). The LF:HF ratio value of 2, which is considered normal by the Task Force [1], was recorded at 122 m in: 1/5 sub- jects (SS) during the awakening period (W) of sleep; 3/5 subjects (CS, DC, SS) during S1 + S2; and 5/5 subjects during S3 + S4; 2/5 subjects (CS, SS) during the REM sleep stage. A LF:HF ratio value of 2 was reached after 30 - 41 h of acclimatization at 3480 m by: 1/5 subjects (GM) during the awakening period (W) of sleep; 1/5 subjects (CS) during S1 + S2; and 2/5 subjects (CS, GM) during S3 + S4. No changes were noted between the av- erage LF:HF ratio recorded in all five mountain runners at 122 m and after 30 - 41 h of acclimatization at 3480 m and during all four stages of sleep: awakening period (W) during sleep; S1 + S2; S3 + S4; and REM sleep. These results suggest highly controlled regulation of sympa- thovagal balance on exposure to moderate hypoxia con- ditions. As reported in Table 7, several mountain mara- thon runners showed very high control of sympathovagal balance: their LF:HF ratio was similar to that reported by the Task Force [1] or did not differ between 122 m and 3480 m, after 30 - 41 h of acclimatization, during the awakening period (W) during sleep, during S1 + S2; during S3 + S4, or during the REM sleep. Cornolo and co-workers [20] reported an average LF:HF ratio of ~6.0 in trained high-altitude native subjects 6 - 8 h after the end of a marathon performed between 4100 and 4400 m. This was similar to the average LF:HF ratio that we re- corded at 3480 m during the desynchronized state of sleep, specifically, during the awakening period (W) dur- ing sleep (6.0036 ± 5.0276) and during the REM sleep at the same altitude (6.9132 ± 3.6806). 5.11. Total Power We observed significant parallel changes in TP recorded at low (PB about 740 mm Hg) and high (PB 495 mm Hg) altitude between the awakening period (W) during sleep and S3 + S4 of sleep. We also found significant parallel changes in the TP recorded during REM sleep and the average of TP recorded during S3 + S4 at 122 and 3480 m. These changes may reflect changes in cardiac, re- spiratory and sympathovagal balance. In general, in indi- vidual mountain marathon runners we found a decrease in the total power at altitude. The components of HRV are thought to be influenced by both neuronal and hu- moral factors (Task Force, [1]). Saito and co-workers [25] reported that humoral control of the autonomic nervous system plays an important role in the survival of victims under hypoxic conditions at high altitude. 6. Functional Significance Physical training at low and high altitudes may have in- duced marked autonomic adaptations in the mountain marathon runners over the course of their years of train- ing. The resting heart rate was lower at sea level because parasympathetic modulation predominates over higher sympathetic control at high altitude. By constantly main- taining their training levels at low and high altitudes for more than 20 years, the mountain runners may have shifted their cardiovascular autonomic control toward a greater parasympathetic modulation, particularly at sea level. Rest and training under hypoxia conditions may have balanced the prevalence of parasympathetic modu- lation, as well as improved control of phasic sympathetic responses. These well-trained runners seem to be exceptionally adapted to the high altitude environment [4-13]. Strenu- ous exercise is known to induce profound cardiovascular modifications, but with a caveat for non-professional ma- rathoners. We agree with Lanfranchi et al. [2], that HRV analysis has proved to be essential for investigating the mountain sickness, the balance of the sympathetic and parasympa- thetic function of the autonomic nervous system at low and high altitude in mountain marathon runners. HRV analysis could also be very useful for evaluating de- creases in parasympathetic and increases in sympathetic tone of the autonomic nervous system during acclimati- zation at altitude of mountain marathon runners who ge- nerally have a tonic prevalence of parasympathetic tone at low altitude. R-R interval variability was observed in the mountain marathon runners during sleep at 122 m (i.e., a controlled increase in parasympathetic tone during NREM sleep and an increased sympathetic tone during the awakening pe- riod (W) during sleep and during REM sleep). At high altitude, the RR-intervals we observed were similar to those Sacknoff and co-workers [21] reported in athletes and controls. Moreover, the major ECG alterations evi- dent during sleep, at an altitude of 3480 m, at a PB of 495 mm Hg, and after 30 - 41 hours of acclimatization, were an increase in HR, together with signs of sinus arrhyth-
 I. GRITTI ET AL. Copyright © 2013 SciRes. JBBS 47 mia during periodic breathing in S1 + S2 of NREM sleep and in REM sleep. This study did not find abnormal reductions in the R-R interval during the awakening period (W) during sleep, S1 + S2, S3 + S4 and REM sleep, between 30 and 41 h of acclimatization at 3480 m in these well-trained moun- tain marathon runners. The HRV data observed at altitude suggest that the runners possess an intrinsic high parasympathetic tone that at high altitude promptly compensates the physio- logical increase in sympathetic tone due to a periodic, significant decrease in peripheral oxygen saturation dur- ing sleep. Not in group average but in several individual runners we found a significant reduction in the LF component at high altitude which may be due to the rhythmic increase of sympathetic discharge of the central brain stem center. In comparison to the average calculated at low altitude, we also found a significant decrease in the average of the power of HF during S3 + S4 of NREM sleep at 3480 m. This significant reduction probably indicates a decrease in the vagal parasympathetic components of the brain- stem generator. The study demonstrates that cardiovascular modifi- cations during sleep and after 30 - 41 h of acclimatization at high altitude can occur even in mountain marathoners who have benefited from 20 years of training at low and high altitude. Physicians should therefore take the results of HRV analysis into account to improve the training and performance, particularly at altitude. 7. Acknowledgements Thanks are due to K. Britsch for reading the manuscript. We thank the FILA Sky Marathon for economical sup- port from 1998 up to 2003. REFERENCES [1] Task Force of the European Society of Cardiology and North America Society of Pacing Electrophysiology, “Heart Rate Variability, Standard of Measurement, Phy- siological Interpretation and Clinical Use,” European Heart Journal, Vol. 17, 1996, pp. 354-381. doi:10.1093/oxfordjournals.eurheartj.a014868 [2] P. A. Lanfranchi, R. Colombo, G. Cremona, P. Baderna, L. Spagnolati, G. Mazzuero, P. Wagner, L. Perini, H. Wagner, C. Cavallaro and P. Giannuzzi, “Autonomic Car- diovascular Regulation in Subjects with Acute Mountain Sickness,” American Physiology Heart Circulation Physi- ology, Vol. 289, No. 6, 2005, pp. H2364-H2372. doi:10.1152/ajpheart.00004.2005 [3] M. Ako, K. Tokuhiro, S. Uchida, S. Miyazaky, K. Nishi- hara, J. Mukai, K. Hirao J. Ako and Y. Okubo, “Correla- tion between Electrophysiography and Heart Rate Vari- ability during Sleep,” Psychiatry and Clinical Neurosci- ence, Vol. 57, No. 1, 2003, pp. 59-65. doi:10.1046/j.1440-1819.2003.01080.x [4] G. Banfi, M. Marinelli, G. S. Roi, A. Colombini, M. Pon- tillo, M. Giacometti and S. Wade, “Growth Hormone and Insulin-Like Growth Factor I in Athletes Performing a Marathon at 4000 m of Altitude,” Growth Regulation, Vol. 4, 1994, pp. 82-86. [5] M. Marinelli, G. S. Roi, M. Giacometti, P. Bonini and G. Banfi, “Cortisol, Testosterone and Free Testosterone in Athletes Performing a Marathon at 4000 m of Altitude,” Hormon Research, Vol. 41, No. 5-6, 1994, pp. 225-229. doi:10.1159/000183929 [6] G. Banfi, M. Marinelli, G. S. Roi and M. Giacometti, “Platelet Indices in Athletes Performing a Race in Alti- tude Environment,” Journal of Clinical Laboratory Ana- lysis, Vol. 9, No. 1, 1995, pp. 34-36. doi:10.1002/jcla.1860090106 [7] G. Banfi, M. Marinelli, P. Bonini, I. Gritti and G. S. Roi, “Pepsinogens and Gastrointestinal Symptoms in Moun- tain Marathon Runners,” International Journal Sports Me- dicine, Vol. 17, No. 8, 1996, pp. 554-558. doi:10.1055/s-2007-972894 [8] G. Banfi, M. Pontillo, M. Marinelli, A. Dolci and G. S. Roi, “Thyrotropin and Free Thyroid Hormones in Ath- letes during and after Ultra Endurance Sport Perform- ances,” Journal Clinical of Ligand Assay, Vol. 21, No. 3, 1998, pp. 331-334. [9] G. S. Roi, M. Giacometti and S. P. von Duvillard, “Mara- thons in Altitude,” Medicine & Science in Sports & Exer- cise, Vol. 31, No. 5, 1999, pp. 723-728. doi:10.1097/00005768-199905000-00016 [10] G. Banfi and A. Dolci, “Preanalytical Phase of Sport Bio- chemistry and Haematology,” The Journal of Sports Me- dicine and Physical Fitness, Vol. 43, No. 2, 2003, pp. 223-230. [11] G. Pelamatti, M. Pascotto and C. Semenza, “Verbal Free Recall in High Altitude: Proper Names vs Common Names,” Cortex, Vol. 39, No. 1, 2003, pp. 97-103. doi:10.1016/S0010-9452(08)70077-7 [12] G. Fragasso, A. Palloshi, S. Roi, E. Rossetti, G. Monaco, A. Dolci, A. Margonato and S. Chierchia, “Transient Left Ventricular Diastolic Dysfunction Following Strenuous Exercise,” Sport Science Health, Vol. 1, No. 1, 2004, pp. 31-35. doi:10.1007/s11332-004-0007-7 [13] I. Gritti, M. Martignoni, R. Calcaterra and G. S. Roi, “Electroencephalographic Changes after a Marathon at 4300 m of Altitude,” Journal of Behavioral and Brain Science, Vol. 2, No. 3, 2012, pp. 380-386. doi:10.4236/jbbs.2012.23043 [14] H. Otzenberger, C. Simon, C. Gronfier and G. Branden- berger, “Temporal Relationship between Dynamic Heart Rate Variability and Electroencephalographic Activity during Sleep in Man,” Neuroscience Letters, Vol. 229, No. 3, 1997, pp. 173-176. doi:10.1016/S0304-3940(97)00448-5 [15] J. H. Coote, “Medicine and Mechanisms in Altitude Sick- ness. Recommendations,” Sports Medicine, Vol. 20, No. 3, 1995, pp. 148-159.
 I. GRITTI ET AL. Copyright © 2013 SciRes. JBBS 48 doi:10.2165/00007256-199520030-00003 [16] M. A. Conney, R. W. van Arts, L. A. A. Kollée, J. C. W. Hopman, G. B. A. Stoelinga, P. Herman and H. P. van Geijn, “Heart Rate Variability,” Annals of Internal Medi- cine, Vol. 118, No. 6, 1993, pp. 436-447. [17] A. Rechtschaffen and A. Kales, “A Manual of Standard- ized Terminology, Techniques and Scoring System for Sleep Stages of Human Subjects,” BIS/BRI UCLA, Los Angeles, 1968. [18] F. R. Calaresu, A. A. Faiers and G. J. Mogenson, “Central Neuronal Regulation of the Heart and Blood Vessels in Mammals,” Progress Neurobiology, Vol. 5, No. 1, 1975, pp. 1-35. doi:10.1016/0301-0082(75)90006-4 [19] H. Scholz, “Adaptational Responses to Hypoxia,” Ameri- can Journal of Physiology and Regulatory Integrative Comparative Physiology, Vol. 282, No. 6, 2002, pp. R1541-R1543. [20] J. Cornolo, J. V. Brugniaux, J. L. Marcarlupu, C. Privat, F. Leon-Velarde and J. P. Richalet, “Autonomic Adaptations in Andean-Trained Participants to 4220 m Altitude. Mara- thon,” Medicine and Science in Sports and Exercise, Vol. 37, No. 12, 2005, pp. 2148-2153. doi:10.1249/01.mss.0000179901.19280.85 [21] D. M. Sacknoff, G. W. Gleim, N. Stachenfeld and N. L. Complan, “Effect of Athletic Training on Heart Variabil- ity,” American Heart Journal, Vol. 127, No. 5, 1994, pp. 1275-1278. doi:10.1016/0002-8703(94)90046-9 [22] L. Bernardi, C. Passino, G. Spadaccini, A. Calciati, R. Robergs, R. Green, E. Martignoni, I. Anand and O. Ap- penzeller, “Cardiovascular Autonomic Modulation and Activity of Carotid Baroreceptors at Altitude,” Clinical Science, Vol. 95, 1998, pp. 565-573. doi:10.1042/CS19980046 [23] G. Vandewalle, B. Middleton, S. W. R. Rajaratnam, B. M. Stone, B. Thorleifsdottir, J. Aredent and D. J. Dijk, “Ro- bust Circadian Rhythm in Heart Rate and Its Variability: Influence of Melatonin and Photoperiod,” Journal of S lee p Research, Vol. 16, No. 2, 2007, pp. 148-150. doi:10.1111/j.1365-2869.2007.00581.x [24] C. Murrell, L. Wilson, J. D. Cotter, S. Lucas, S. Ogoh, G. Keith and N. Ainslie, “Alteration in Autonomic Function and Cerebral Hemodynamics to Orthostatic Challenge Fol- lowing a Mountain Marathon,” Journal Applied Physiol- ogy, Vol. 103, No. 1, 2007, pp. 88-96. doi:10.1152/japplphysiol.01396.2006 [25] S. Saito, K. Tanobe, M. Yamada and F. Nishihara, “Rela- tionship between Arterial Oxygen Saturation and Heart Rate Variability at High Altitudes,” American Journal of Emergency Medicine, Vol. 23, No. 1, 2005, pp. 8-12. doi:10.1016/j.ajem.2004.09.023
|