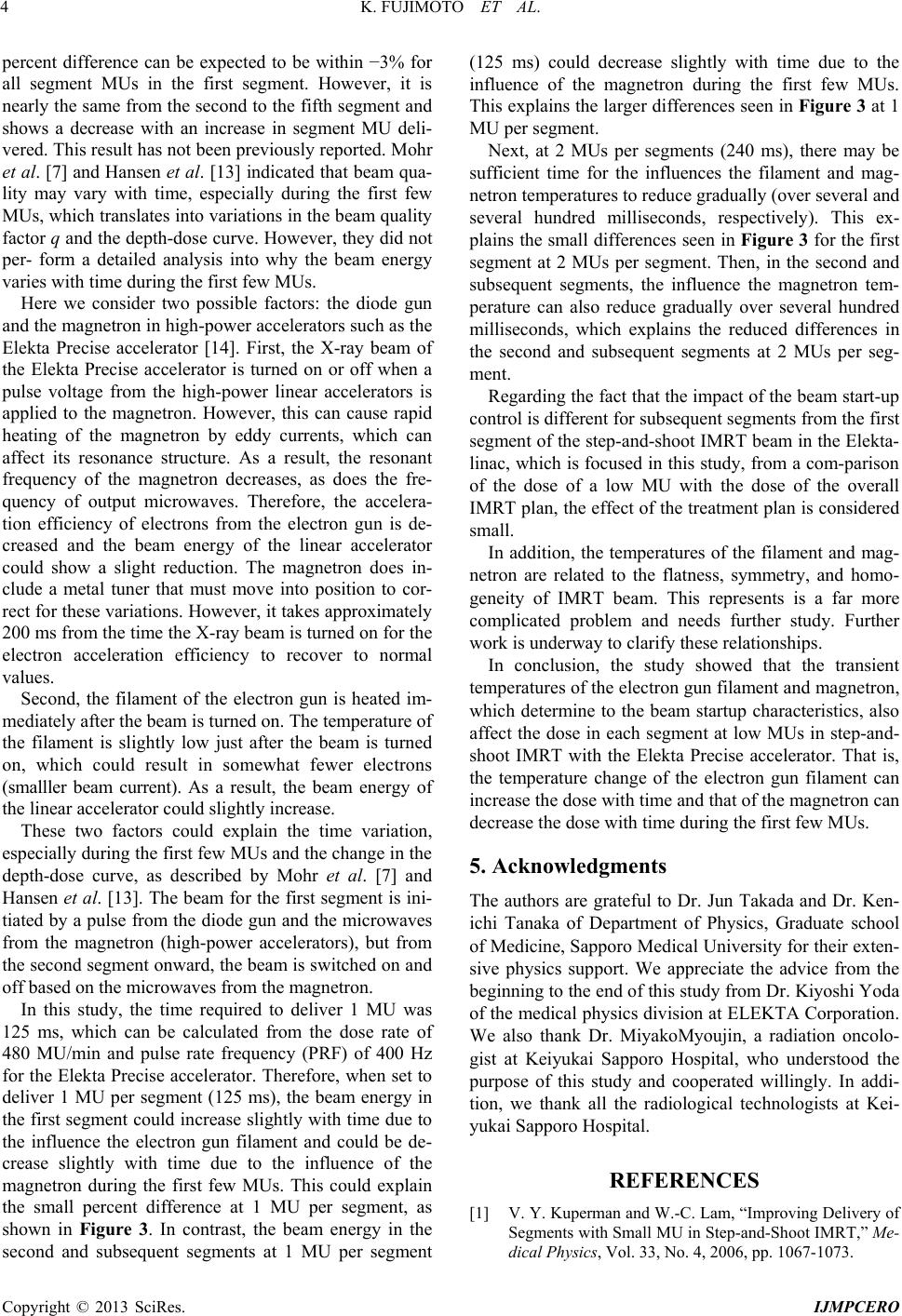
K. FUJIMOTO ET AL.
4
percent difference can be expected to be within −3% for
all segment MUs in the first segment. However, it is
nearly the same from the second to the fifth segment and
shows a decrease with an increase in segment MU deli-
vered. This result has not been previously reported. Mohr
et al. [7] and Hansen et al. [13] indicated that beam qua-
lity may vary with time, especially during the first few
MUs, which translates into variations in the beam quality
factor q and the depth-dose curve. However, they did not
per- form a detailed analysis into why the beam energy
varies with time during the first few MUs.
Here we consider two possible factors: the diode gun
and the magnetron in high-power accelerators such as the
Elekta Precise accelerator [14]. First, the X-ray beam of
the Elekta Precise accelerator is turned on or off when a
pulse voltage from the high-power linear accelerators is
applied to the magnetron. However, this can cause rapid
heating of the magnetron by eddy currents, which can
affect its resonance structure. As a result, the resonant
frequency of the magnetron decreases, as does the fre-
quency of output microwaves. Therefore, the accelera-
tion efficiency of electrons from the electron gun is de-
creased and the beam energy of the linear accelerator
could show a slight reduction. The magnetron does in-
clude a metal tuner that must move into position to cor-
rect for these variations. However, it takes approximately
200 ms from the time the X-ray beam is turned on for the
electron acceleration efficiency to recover to normal
values.
Second, the filament of the electron gun is heated im-
mediately after the beam is turned on. The temperature of
the filament is slightly low just after the beam is turned
on, which could result in somewhat fewer electrons
(smalller beam current). As a result, the beam energy of
the linear accelerator could slightly increase.
These two factors could explain the time variation,
especially during the first few MUs and the change in the
depth-dose curve, as described by Mohr et al. [7] and
Hansen et al. [13]. The beam for the first segment is ini-
tiated by a pulse from the diode gun and the microwaves
from the magnetron (high-power accelerators), but from
the second segment onward, the beam is switched on and
off based on the microwaves from the magnetron.
In this study, the time required to deliver 1 MU was
125 ms, which can be calculated from the dose rate of
480 MU/min and pulse rate frequency (PRF) of 400 Hz
for the Elekta Precise accelerator. Therefore, when set to
deliver 1 MU per segment (125 ms), the beam energy in
the first segment could increase slightly with time due to
the influence the electron gun filament and could be de-
crease slightly with time due to the influence of the
magnetron during the first few MUs. This could explain
the small percent difference at 1 MU per segment, as
shown in Figure 3. In contrast, the beam energy in the
second and subsequent segments at 1 MU per segment
(125 ms) could decrease slightly with time due to the
influence of the magnetron during the first few MUs.
This explains the larger differences seen in Figure 3 at 1
MU per segment.
Next, at 2 MUs per segments (240 ms), there may be
sufficient time for the influences the filament and mag-
netron temperatures to reduce gradually (over several and
several hundred milliseconds, respectively). This ex-
plains the small differences seen in Figure 3 for the first
segment at 2 MUs per segment. Then, in the second and
subsequent segments, the influence the magnetron tem-
perature can also reduce gradually over several hundred
milliseconds, which explains the reduced differences in
the second and subsequent segments at 2 MUs per seg-
ment.
Regarding the fact that the impact of the beam start-up
control is different for subsequent segments from the first
segment of the step-and-shoot IMRT beam in the Elekta-
linac, which is focused in this study, from a com-parison
of the dose of a low MU with the dose of the overall
IMRT plan, the effect of the treatment plan is considered
small.
In addition, the temperatures of the filament and mag-
netron are related to the flatness, symmetry, and homo-
geneity of IMRT beam. This represents is a far more
complicated problem and needs further study. Further
work is underway to clarify these relationships.
In conclusion, the study showed that the transient
temperatures of the electron gun filament and magnetron,
which determine to the beam startup characteristics, also
affect the dose in each segment at low MUs in step-and-
shoot IMRT with the Elekta Precise accelerator. That is,
the temperature change of the electron gun filament can
increase the dose with time and that of the magnetron can
decrease the dose with time during the first few MUs.
5. Acknowledgments
The authors are grateful to Dr. Jun Takada and Dr. Ken-
ichi Tanaka of Department of Physics, Graduate school
of Medicine, Sapporo Medical University for their exten-
sive physics support. We appreciate the advice from the
beginning to the end of this study from Dr. Kiyoshi Yoda
of the medical physics division at ELEKTA Corporation.
We also thank Dr. MiyakoMyoujin, a radiation oncolo-
gist at Keiyukai Sapporo Hospital, who understood the
purpose of this study and cooperated willingly. In addi-
tion, we thank all the radiological technologists at Kei-
yukai Sapporo Hospital.
REFERENCES
[1] V. Y. Kuperman and W.-C. Lam, “Improving Delivery of
Segments with Small MU in Step-and-Shoot IMRT,” Me-
dical Physics, Vol. 33, No. 4, 2006, pp. 1067-1073.
Copyright © 2013 SciRes. IJMPCERO