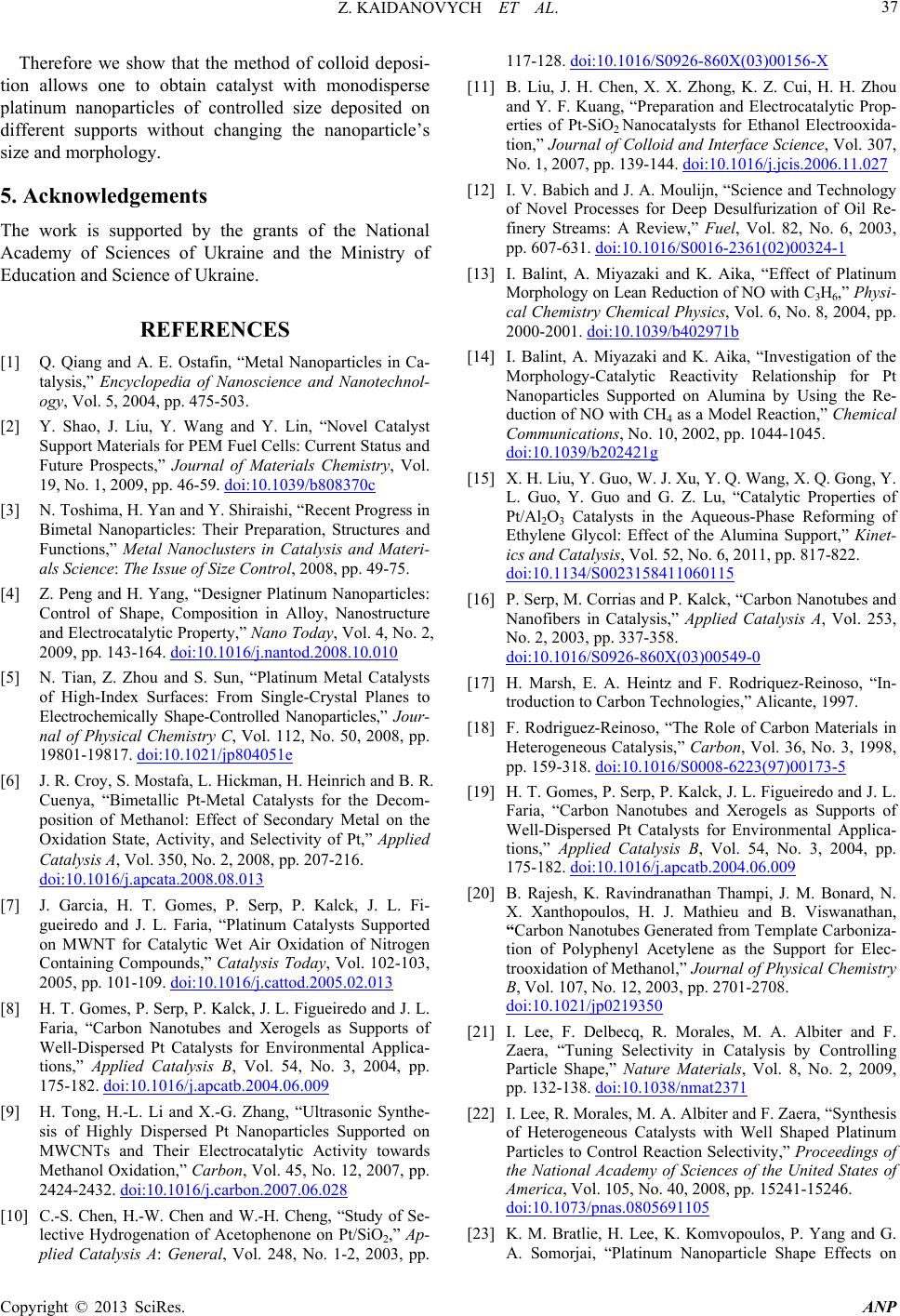
Z. KAIDANOVYCH ET AL. 37
Therefore we show that the method of colloid deposi-
tion allows one to obtain catalyst with monodisperse
platinum nanoparticles of controlled size deposited on
different supports without changing the nanoparticle’s
size and morphology.
5. Acknowledgements
The work is supported by the grants of the National
Academy of Sciences of Ukraine and the Ministry of
Education and Science of Ukraine.
REFERENCES
[1] Q. Qiang and A. E. Ostafin, “Metal Nanoparticles in Ca-
talysis,” Encyclopedia of Nanoscience and Nanotechnol-
ogy, Vol. 5, 2004, pp. 475-503.
[2] Y. Shao, J. Liu, Y. Wang and Y. Lin, “Novel Catalyst
Support Materials for PEM Fuel Cells: Current Status and
Future Prospects,” Journal of Materials Chemistry, Vol.
19, No. 1, 2009, pp. 46-59. doi:10.1039/b808370c
[3] N. Toshima, H. Yan and Y. Shiraishi, “Recent Progress in
Bimetal Nanoparticles: Their Preparation, Structures and
Functions,” Metal Nanoclusters in Catalysis and Materi-
als Science: The Issue of Size Control, 2008, pp. 49-75.
[4] Z. Peng and H. Yang, “Designer Platinum Nanoparticles:
Control of Shape, Composition in Alloy, Nanostructure
and Electrocatalytic Property,” Nano Today, Vol. 4, No. 2,
2009, pp. 143-164. doi:10.1016/j.nantod.2008.10.010
[5] N. Tian, Z. Zhou and S. Sun, “Platinum Metal Catalysts
of High-Index Surfaces: From Single-Crystal Planes to
Electrochemically Shape-Controlled Nanoparticles,” Jour-
nal of Physical Chemistry C, Vol. 112, No. 50, 2008, pp.
19801-19817. doi:10.1021/jp804051e
[6] J. R. Croy, S. Mostafa, L. Hickman, H. Heinrich and B. R.
Cuenya, “Bimetallic Pt-Metal Catalysts for the Decom-
position of Methanol: Effect of Secondary Metal on the
Oxidation State, Activity, and Selectivity of Pt,” Applied
Catalysis A, Vol. 350, No. 2, 2008, pp. 207-216.
doi:10.1016/j.apcata.2008.08.013
[7] J. Garcia, H. T. Gomes, P. Serp, P. Kalck, J. L. Fi-
gueiredo and J. L. Faria, “Platinum Catalysts Supported
on MWNT for Catalytic Wet Air Oxidation of Nitrogen
Containing Compounds,” Catalysis Today, Vol. 102-103,
2005, pp. 101-109. doi:10.1016/j.cattod.2005.02.013
[8] H. T. Gomes, P. Serp, P. Kalck, J. L. Figueiredo and J. L.
Faria, “Carbon Nanotubes and Xerogels as Supports of
Well-Dispersed Pt Catalysts for Environmental Applica-
tions,” Applied Catalysis B, Vol. 54, No. 3, 2004, pp.
175-182. doi:10.1016/j.apcatb.2004.06.009
[9] H. Tong, H.-L. Li and X.-G. Zhang, “Ultrasonic Synthe-
sis of Highly Dispersed Pt Nanoparticles Supported on
MWCNTs and Their Electrocatalytic Activity towards
Methanol Oxidation,” Carbon, Vol. 45, No. 12, 2007, pp.
2424-2432. doi:10.1016/j.carbon.2007.06.028
[10] C.-S. Chen, H.-W. Chen and W.-H. Cheng, “Study of Se-
lective Hydrogenation of Acetophenone on Pt/SiO2,” Ap-
plied Catalysis A: General, Vol. 248, No. 1-2, 2003, pp.
117-128. doi:10.1016/S0926-860X(03)00156-X
[11] B. Liu, J. H. Chen, X. X. Zhong, K. Z. Cui, H. H. Zhou
and Y. F. Kuang, “Preparation and Electrocatalytic Prop-
erties of Pt-SiO2 Nanocatalysts for Ethanol Electrooxida-
tion,” Journal of Colloid and Interface Science, Vol. 307,
No. 1, 2007, pp. 139-144. doi:10.1016/j.jcis.2006.11.027
[12] I. V. Babich and J. A. Moulijn, “Science and Technology
of Novel Processes for Deep Desulfurization of Oil Re-
finery Streams: A Review,” Fuel, Vol. 82, No. 6, 2003,
pp. 607-631. doi:10.1016/S0016-2361(02)00324-1
[13] I. Balint, A. Miyazaki and K. Aika, “Effect of Platinum
Morphology on Lean Reductio n of NO with C3H6,” Physi-
cal Chemistry Chemical Physics, Vol. 6, No. 8, 2004, pp.
2000-2001. doi:10.1039/b402971b
[14] I. Balint, A. Miyazaki and K. Aika, “Investigation of the
Morphology-Catalytic Reactivity Relationship for Pt
Nanoparticles Supported on Alumina by Using the Re-
duction of NO with CH4 as a Model Reaction,” Chemical
Communications, No. 10, 2002, pp. 1044-1045.
doi:10.1039/b202421g
[15] X. H. Liu, Y. Guo, W. J. Xu, Y. Q. Wang, X. Q. Gong, Y.
L. Guo, Y. Guo and G. Z. Lu, “Catalytic Properties of
Pt/Al2O3 Catalysts in the Aqueous-Phase Reforming of
Ethylene Glycol: Effect of the Alumina Support,” Kinet-
ics and Catalysis, Vol. 52, No. 6, 2011, pp. 817-822.
doi:10.1134/S0023158411060115
[16] P. Serp, M. Corrias and P. Kalck, “Carbon Nanotubes and
Nanofibers in Catalysis,” Applied Catalysis A, Vol. 253,
No. 2, 2003, pp. 337-358.
doi:10.1016/S0926-860X(03)00549-0
[17] H. Marsh, E. A. Heintz and F. Rodriquez-Reinoso, “In-
troduction to Carbon Technologies,” Alicante, 1997.
[18] F. Rodriguez-Reinoso, “The Role of Carbon Materials in
Heterogeneous Catalysis,” Carbon, Vol. 36, No. 3, 1998,
pp. 159-318. doi:10.1016/S0008-6223(97)00173-5
[19] H. T. Gomes, P. Serp, P. Kalck, J. L. Figueiredo and J. L.
Faria, “Carbon Nanotubes and Xerogels as Supports of
Well-Dispersed Pt Catalysts for Environmental Applica-
tions,” Applied Catalysis B, Vol. 54, No. 3, 2004, pp.
175-182. doi:10.1016/j.apcatb.2004.06.009
[20] B. Rajesh, K. Ravindranathan Thampi, J. M. Bonard, N.
X. Xanthopoulos, H. J. Mathieu and B. Viswanathan,
“Carbon Nanotubes Generated from Template Carboniza-
tion of Polyphenyl Acetylene as the Support for Elec-
trooxidation of Methanol,” Journal of Physical Chemistry
B, Vol. 107, No. 12, 2003, pp. 2701-2708.
doi:10.1021/jp0219350
[21] I. Lee, F. Delbecq, R. Morales, M. A. Albiter and F.
Zaera, “Tuning Selectivity in Catalysis by Controlling
Particle Shape,” Nature Materials, Vol. 8, No. 2, 2009,
pp. 132-138. doi:10.1038/nmat2371
[22] I. Lee, R. Morales, M. A. Albiter and F. Zaera, “Synthesis
of Heterogeneous Catalysts with Well Shaped Platinum
Particles to Control Reaction Selectivity,” Proceedings of
the National Academy of Sciences of the United States of
America, Vol. 105, No. 40, 2008, pp. 15241-15246.
doi:10.1073/pnas.0805691105
[23] K. M. Bratlie, H. Lee, K. Komvopoulos, P. Yang and G.
A. Somorjai, “Platinum Nanoparticle Shape Effects on
Copyright © 2013 SciRes. ANP