 Open Journal of Psychiatry, 2013, 3, 46-139 OJPsych http://dx.doi.org/10.4236/ojpsych.2013.31008 Published Online January 2013 (http://www.scirp.org/journal/ojpsych/) Genomics of schizophrenia and pharmacogenomics of antipsychotic drugs Ramón Cacabelos1*, Pablo Cacabelos1, Gjumrakch Aliev1,2 1EuroEspes Biomedical Research Center, Institute for CNS Disorders and Genomic Medicine, EuroEspes Chair of Biotechnology and Genomics, Camilo José Cela University, Corunna, Spain 2Gally International Biomedical Research Consulting LLC, San Antonio, USA Email: *rcacabelos@euroespes.com Received 13 October 2012; revised 15 November 2012; accepted 24 November 2012 ABSTRACT Antipsychotic drugs are the neuroleptics currently used in the treatment of schizophrenia (SCZ) and psychotic disorders. SCZ has a heritability estimated at 70% - 90%; and pharmacogenomics accounts for 60% - 90% variability in the pharmacokinetics and pharmacodynamics of psychotropic drugs. Personal- ized therapeutics based on individual genomic pro- files in SCZ entails the characterization of 5 types of gene clusters and their related metabolomic profiles: 1) genes associated with disease pathogenesis; 2) genes associated with the mechanism of action of drugs; 3) genes associated with drug metabolism (phase I and II reactions); 4) genes associated with drug transporters; and 5) pleiotropic genes involved in multifaceted cascades and metabolic reactions. Genetic studies in SCZ have revealed the presence of chromosome anomalies, copy number variants, mul- tiple single-nucleotide polymorphisms of susceptibil- ity distributed across the human genome, aberrant single-nucleotide polymorphisms in microRNA genes, mitochondrial DNA mutations, and epigenetic phe- nomena. Pharmacogenetic studies of psychotropic drug response have focused on determining the rela- tionship between variation in specific candidate genes and the positive and adverse effects of drug treatment. Approximately 18% of neuroleptics are major sub- strates of CYP1A2 enzymes, 40% of CYP2D6, and 23% of CYP3A4. About 10% - 20% of Western populations are defective in genes of the CYP super- family. Only 26% of Southern Europeans are pure extensive metabolizers for the trigenic cluster inte- grated by the CYP2D6 + CYP2C19 + CYP2C9 genes. Efficacy and safety issues in the pharmacological treatment of SCZ are directly linked to genetic clus- ters involved in the pharmacogenomics of antipsy- chotic drugs and also to environmental factors. Con- sequently, the incorporation of pharmacogenomic procedures both to drugs under development and drugs on the market would help to optimize thera- peutics in SCZ and other central nervous system dis- orders. Keywords: Genomics; Antipsychotic Drugs; Schizophrenia 1. INTRODUCTION Central nervous system (CNS) disorders are the third greatest problem of health in developed countries, repre- senting 10% - 15% of deaths after cardiovascular disor- ders (25%) and cancer (20%). CNS disorders pose sev- eral challenges to our society and the scientific commu- nity: 1) they represent an epidemiological problem and a socio-economic, psychological and family burden; 2) most of them have an obscure/complex pathogenesis; 3) their diagnosis is not easy and lacks specific biomarkers; and 4) their treatment is difficult and inefficient. In terms of economic burden, approximately 10% - 20% of direct costs are associated with their pharmacological treatment, with a gradual increase in parallel with the severity of the disease [1,2]. Approximately 127 million Europeans suffer brain disorders. The total annual cost of brain disorders in Europe is about €386 billion, with €135 billion in direct medical expenditures (€78 billion, inpatients; €45 billion, outpatients; €13 billion, pharmacological treatment), €179 billion in indirect costs (lost workdays, loss of productivity, permanent disability), and €72 billion in direct non-medical costs. Mental disorders represent €240 billion (62% of the total cost, excluding dementia), followed by neurological diseases (€84 billion, 22%) [3]. Common features in CNS disorders include the fol- lowing: 1) polygenic/complex disorders in which genetic, epigenetic and environmental factors are involved; 2) deterioration of higher activities of the CNS; 3) multi- factorial dysfunctions in several metabolomic networks leading to functional damage to specific brain circuits; *Corresponding author. OPEN ACCESS
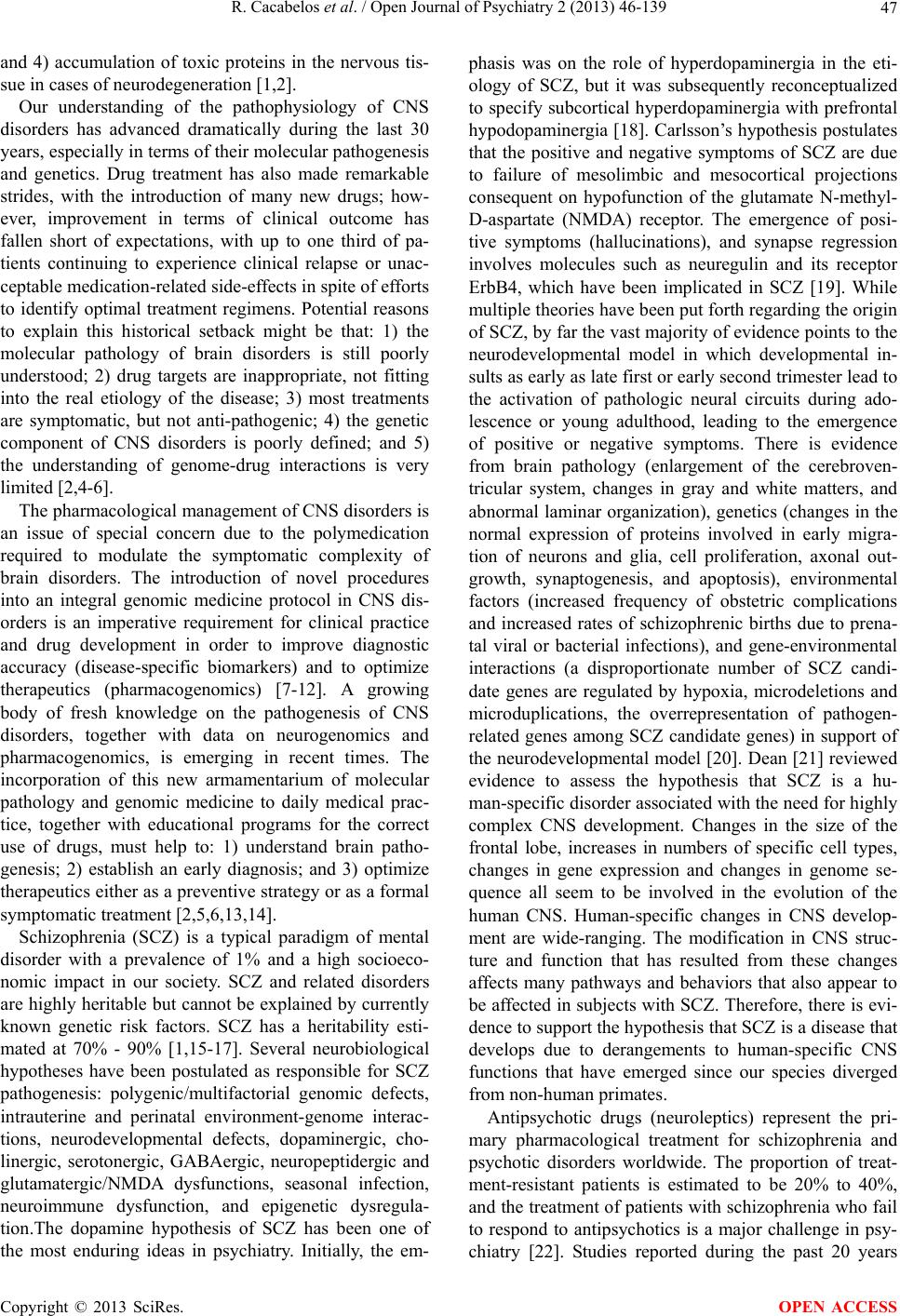 R. Cacabelos et al. / Open Journal of Psychiatry 2 (2013) 46-139 47 and 4) accumulation of toxic proteins in the nervous tis- sue in cases of neurodegeneration [1,2]. Our understanding of the pathophysiology of CNS disorders has advanced dramatically during the last 30 years, especially in terms of their molecular pathogenesis and genetics. Drug treatment has also made remarkable strides, with the introduction of many new drugs; how- ever, improvement in terms of clinical outcome has fallen short of expectations, with up to one third of pa- tients continuing to experience clinical relapse or unac- ceptable medication-related side-effects in spite of efforts to identify optimal treatment regimens. Potential reasons to explain this historical setback might be that: 1) the molecular pathology of brain disorders is still poorly understood; 2) drug targets are inappropriate, not fitting into the real etiology of the disease; 3) most treatments are symptomatic, but not anti-pathogenic; 4) the genetic component of CNS disorders is poorly defined; and 5) the understanding of genome-drug interactions is very limited [2,4-6]. The pharmacological management of CNS disorders is an issue of special concern due to the polymedication required to modulate the symptomatic complexity of brain disorders. The introduction of novel procedures into an integral genomic medicine protocol in CNS dis- orders is an imperative requirement for clinical practice and drug development in order to improve diagnostic accuracy (disease-specific biomarkers) and to optimize therapeutics (pharmacogenomics) [7-12]. A growing body of fresh knowledge on the pathogenesis of CNS disorders, together with data on neurogenomics and pharmacogenomics, is emerging in recent times. The incorporation of this new armamentarium of molecular pathology and genomic medicine to daily medical prac- tice, together with educational programs for the correct use of drugs, must help to: 1) understand brain patho- genesis; 2) establish an early diagnosis; and 3) optimize therapeutics either as a preventive strategy or as a formal symptomatic treatment [2,5,6,13,14]. Schizophrenia (SCZ) is a typical paradigm of mental disorder with a prevalence of 1% and a high socioeco- nomic impact in our society. SCZ and related disorders are highly heritable but cannot be explained by currently known genetic risk factors. SCZ has a heritability esti- mated at 70% - 90% [1,15-17]. Several neurobiological hypotheses have been postulated as responsible for SCZ pathogenesis: polygenic/multifactorial genomic defects, intrauterine and perinatal environment-genome interac- tions, neurodevelopmental defects, dopaminergic, cho- linergic, serotonergic, GABAergic, neuropeptidergic and glutamatergic/NMDA dysfunctions, seasonal infection, neuroimmune dysfunction, and epigenetic dysregula- tion.The dopamine hypothesis of SCZ has been one of the most enduring ideas in psychiatry. Initially, the em- phasis was on the role of hyperdopaminergia in the eti- ology of SCZ, but it was subsequently reconceptualized to specify subcortical hyperdopaminergia with prefrontal hypodopaminergia [18]. Carlsson’s hypothesis postulates that the positive and negative symptoms of SCZ are due to failure of mesolimbic and mesocortical projections consequent on hypofunction of the glutamate N-methyl- D-aspartate (NMDA) receptor. The emergence of posi- tive symptoms (hallucinations), and synapse regression involves molecules such as neuregulin and its receptor ErbB4, which have been implicated in SCZ [19]. While multiple theories have been put forth regarding the origin of SCZ, by far the vast majority of evidence points to the neurodevelopmental model in which developmental in- sults as early as late first or early second trimester lead to the activation of pathologic neural circuits during ado- lescence or young adulthood, leading to the emergence of positive or negative symptoms. There is evidence from brain pathology (enlargement of the cerebroven- tricular system, changes in gray and white matters, and abnormal laminar organization), genetics (changes in the normal expression of proteins involved in early migra- tion of neurons and glia, cell proliferation, axonal out- growth, synaptogenesis, and apoptosis), environmental factors (increased frequency of obstetric complications and increased rates of schizophrenic births due to prena- tal viral or bacterial infections), and gene-environmental interactions (a disproportionate number of SCZ candi- date genes are regulated by hypoxia, microdeletions and microduplications, the overrepresentation of pathogen- related genes among SCZ candidate genes) in support of the neurodevelopmental model [20]. Dean [21] reviewed evidence to assess the hypothesis that SCZ is a hu- man-specific disorder associated with the need for highly complex CNS development. Changes in the size of the frontal lobe, increases in numbers of specific cell types, changes in gene expression and changes in genome se- quence all seem to be involved in the evolution of the human CNS. Human-specific changes in CNS develop- ment are wide-ranging. The modification in CNS struc- ture and function that has resulted from these changes affects many pathways and behaviors that also appear to be affected in subjects with SCZ. Therefore, there is evi- dence to support the hypothesis that SCZ is a disease that develops due to derangements to human-specific CNS functions that have emerged since our species diverged from non-human primates. Antipsychotic drugs (neuroleptics) represent the pri- mary pharmacological treatment for schizophrenia and psychotic disorders worldwide. The proportion of treat- ment-resistant patients is estimated to be 20% to 40%, and the treatment of patients with schizophrenia who fail to respond to antipsychotics is a major challenge in psy- chiatry [22]. Studies reported during the past 20 years Copyright © 2013 SciRes. OPEN ACCESS
 R. Cacabelos et al. / Open Journal of Psychiatry 2 (2013) 46-139 Copyright © 2013 SciRes. 48 2.2. Genes Associated with the Mechanism of Action of Drugs have demonstrated that the efficacy and safety of anti- psychotics are closely related to the pharmacogenomic profiles of schizophrenic patients [1,17,22]. Most genes associated with the mechanism of action of CNS drugs encode receptors, enzymes, and neurotrans- mitters (Tables 1 an d 2) on which psychotropic drugs act as ligands (agonists, antagonists), enzyme modulators (substrates, inhibitors, inducers) or neurotransmitter re- gulators (releasers, reuptake inhibitors) [25]. 2. GENES INVOLVED IN PHARMACOGENOMICS The genes involved in the pharmacogenomic response to drugs in CNS disorders may fall into five major catego- ries: 1) genes associated with CNS pathogenesis (dis- ease-specific genes); 2) genes associated with the mechanism of action of drugs; 3) genes associated with drug metabolism; 4) genes associated with drug trans- porters; and 5) pleiotropic genes involved in multifaceted cascades and metabolic reactions [2]. The therapeutic outcome (efficacy and safety) is the result of the inter- play of drugs with these different categories of gene products and epigenetic factors to reverse or modify the phenotypic expression of a given disease [23]. Pharma- cogenomics accounts for 30% - 90% variability in phar- macokinetics and pharmacodynamics. 2.3. Genes Involved in Drug Metabolism Drug metabolism includes phase I reactions (i.e. oxida- tion, reduction, hydrolysis) and phase II conjugation re- actions (i.e. acetylation, glucuronidation, sulphation, methylation). The principal enzymes with polymorphic variants involved in phase I reactions are the following: Cytochrome P450 monooxygenases (CYP3A4/5/7, CYP- 2E1, CYP2D6, CYP2C19, CYP2C9, CYP2C8, CYP2B6, CYP2A6, CYP1B1, CYP1A1/2), epoxide hydrolase, esterases, NQO1 (NADPH-quinone oxidoreductase), DPD (dihydropyrimidine dehydrogenase), ADH (alcohol dehydrogenase), and ALDH (aldehyde dehydrogenase); and major enzymes involved in phase II reactions in- clude UGTs (uridine 5’-triphosphate glucuronosyl trans- ferases), TPMT (thiopurine methyltransferase), COMT (catechol-O-methyltransferase), HMT (histamine methyl- transferase), STs (sulfotransferases), GST-A (glutathione S-transferase A), GST-P, GST-T, GST-M, NAT1 (N-ace- tyl transferase 1), NAT2, and others. Among these en- zymes, CYP2D6, CYP2C9, CYP2C19, and CYP3A4/5 are the most relevant in the pharmacogenetics of CNS drugs [14,25] (Tabl e 2 ). Approximately, 18% of neuro- leptics are major substrates of CYP1A2 enzymes, 40% 2.1. Pathogenic Genes Over 6000 genes distributed across the human genome have been screened for associations with CNS disorders during the past 30 years [1,24]. Studies of many candi- date genes potentially associated with a particular CNS disorder could not be replicated in different settings, co- horts, and geographical contexts due to methodological problems, sample selection and multi-ethnic genetic variation. In the case of SCZ and related disorders, over 200 genes have been associated with psychotic disorders [1,7] (Figure 1, Table 1). Figure 1. Distribution of genes potentially associated with psychosis and dementia in the human genome. OPEN ACCESS
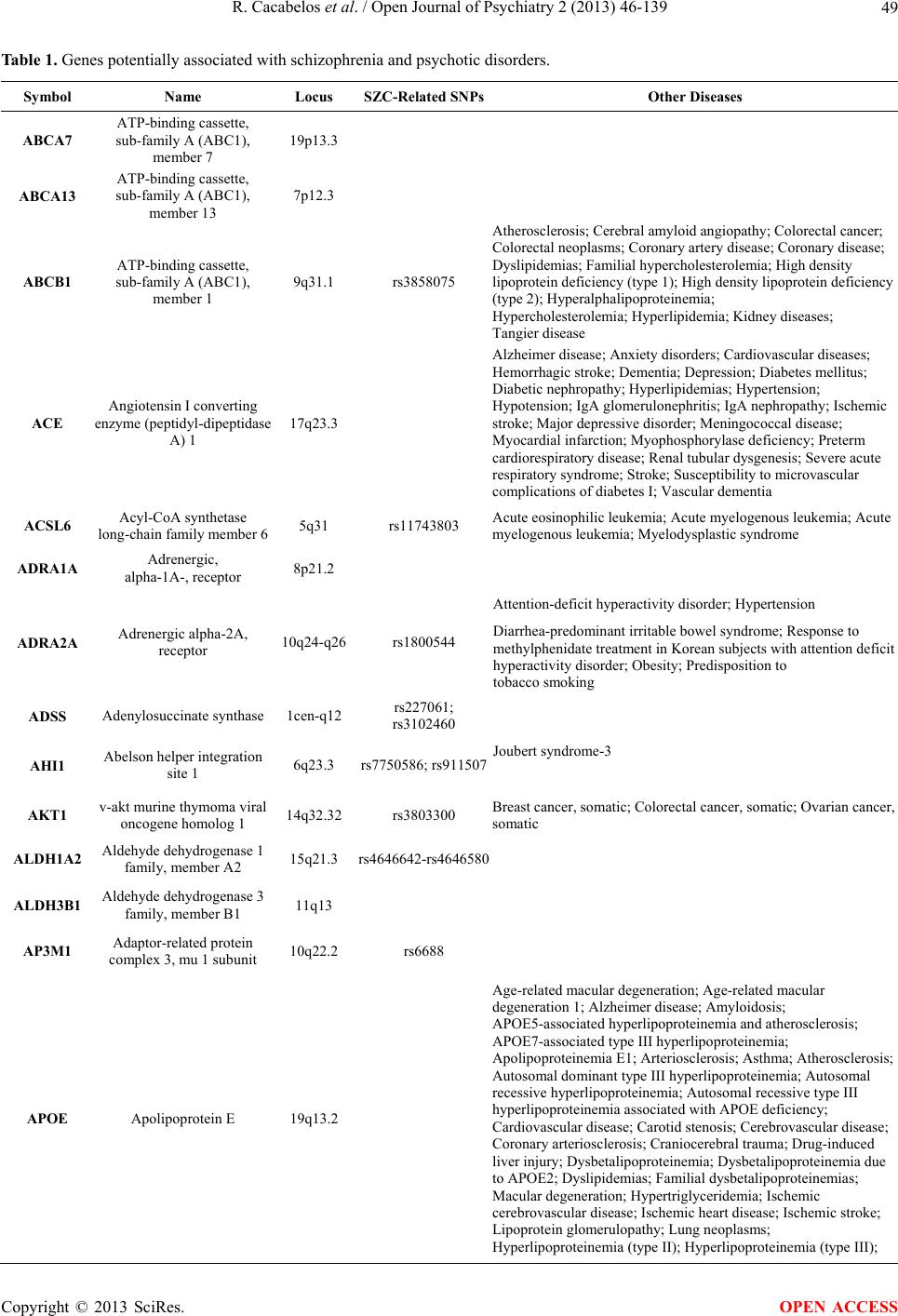 R. Cacabelos et al. / Open Journal of Psychiatry 2 (2013) 46-139 49 Table 1. Genes potentially associated with schizophrenia and psychotic disorders. Symbol Name Locus SZC-Related SNPsOther Diseases ABCA7 ATP-binding cassette, sub-family A (ABC1), member 7 19p13.3 ABCA13 ATP-binding cassette, sub-family A (ABC1), member 13 7p12.3 ABCB1 ATP-binding cassette, sub-family A (ABC1), member 1 9q31.1 rs3858075 Atherosclerosis; Cerebral amyloid angiopathy; Colorectal cancer; Colorectal neoplasms; Coronary artery disease; Coronary disease; Dyslipidemias; Familial hypercholesterolemia; High density lipoprotein deficiency (type 1); High density lipoprotein deficiency (type 2); Hyperalphalipoproteinemia; Hypercholesterolemia; Hyperlipidemia; Kidney diseases; Tangier disease ACE Angiotensin I converting enzyme (peptidyl-dipeptidase A) 1 17q23.3 Alzheimer disease; Anxiety disorders; Cardiovascular diseases; Hemorrhagic stroke; Dementia; Depression; Diabetes mellitus; Diabetic nephropathy; Hyperlipidemias; Hypertension; Hypotension; IgA glomerulonephritis; IgA nephropathy; Ischemic stroke; Major depressive disorder; Meningococcal disease; Myocardial infarction; Myophosphorylase deficiency; Preterm cardiorespiratory disease; Renal tubular dysgenesis; Severe acute respiratory syndrome; Stroke; Susceptibility to microvascular complications of diabetes I; Vascular dementia ACSL6 Acyl-CoA synthetase long-chain family member 6 5q31 rs11743803 Acute eosinophilic leukemia; Acute myelogenous leukemia; Acute myelogenous leukemia; Myelodysplastic syndrome ADRA1A Adrenergic, alpha-1A-, receptor 8p21.2 Attention-deficit hyperactivity disorder; Hypertension ADRA2A Adrenergic alpha-2A, receptor 10q24-q26 rs1800544 Diarrhea-predominant irritable bowel syndrome; Response to methylphenidate treatment in Korean subjects with attention deficit hyperactivity disorder; Obesity; Predisposition to tobacco smoking ADSS Adenylosuccinate synthase 1cen-q12 rs227061; rs3102460 Joubert syndrome-3 AHI1 Abelson helper integration site 1 6q23.3 rs7750586; rs911507 AKT1 v-akt murine thymoma viral oncogene homolog 1 14q32.32 rs3803300 Breast cancer, somatic; Colorectal cancer, somatic; Ovarian cancer, somatic ALDH1A2 Aldehyde dehydrogenase 1 family, member A2 15q21.3 rs4646642-rs4646580 ALDH3B1 Aldehyde dehydrogenase 3 family, member B1 11q13 AP3M1 Adaptor-related protein complex 3, mu 1 subunit 10q22.2 rs6688 APOE Apolipoprotein E 19q13.2 Age-related macular degeneration; Age-related macular degeneration 1; Alzheimer disease; Amyloidosis; APOE5-associated hyperlipoproteinemia and atherosclerosis; APOE7-associated type III hyperlipoproteinemia; Apolipoproteinemia E1; Arteriosclerosis; Asthma; Atherosclerosis; Autosomal dominant type III hyperlipoproteinemia; Autosomal recessive hyperlipoproteinemia; Autosomal recessive type III hyperlipoproteinemia associated with APOE deficiency; Cardiovascular disease; Carotid stenosis; Cerebrovascular disease; Coronary arteriosclerosis; Craniocerebral trauma; Drug-induced liver injury; Dysbetalipoproteinemia; Dysbetalipoproteinemia due to APOE2; Dyslipidemias; Familial dysbetalipoproteinemias; Macular degeneration; Hypertriglyceridemia; Ischemic cerebrovascular disease; Ischemic heart disease; Ischemic stroke; Lipoprotein glomerulopathy; Lung neoplasms; Hyperlipoproteinemia (type II); Hyperlipoproteinemia (type III); Copyright © 2013 SciRes. OPEN ACCESS
 R. Cacabelos et al. / Open Journal of Psychiatry 2 (2013) 46-139 50 Continued Hyperlipoproteinemias; Metabolic syndrome; Multiple sclerosis; Myocardial infarction; Myocardial ischemia; Neurodegenerative diseases; Psoriasis; Sea-blue histiocyte syndrome; Type III hypercholesterolemia and hypertriglyceridemia; Type III hyperlipoproteinemia; Type III hyperlipoproteinemia associated with APOE deficiency; Type III hyperlipoproteinemia associated with APOE Leiden; Type III hyperlipoproteinemia associated with APOE2; Type III hyperlipoproteinemia associated with APOE2-Fukuoka; Type III hyperlipoproteinemia associated with APOE4; Type III hyperlipoproteinemia due to APOE1-Harrisburg; Type III hyperlipoproteinemia due to APOE4-Philadelphia; Type III hyperliporpoteinemia due to APOE2-Christchurch; Type V hyperlipoproteinemia; Vascular dementia; Vascular disease APOL1 Apolipoprotein L, 1 22q13.1 APOL2 Apolipoprotein l, 2 22q12 APOL4 Apolipoprotein l, 4 22q11.2-q13.2 ARRB2 Arrestin, beta 2 17p13 rs1045280 Ser280Ser ARVCF Armadillo repeat gene deleted in velocardiofacial syndrome 22q11.21 ATM Ataxia telangiectasia Mutated 11q22-q23 rs664143 rs227061 AVPR1A Arginine vasopressin receptor 1A 12q14-q15 AVPR1B Arginine vasopressin receptor 1B 1q32 BDNF Brain-derived neurotrophic factor 11p13 rs6265 Val66Met C270T Aniridia; Anorexia nervosa; Bipolar disorder; Bulimia nervosa; Congenital central hypoventilation syndrome; Depressive disorder; Genitourinary anomalies, Lithium response; Major depressive disorder; Memory impairment; Mental retardation; Methadone response; Obesity; Obsessive-compulsive disorder; Parkinson’s disease; Protection against obsessive-compulsive disorder; Tardive dyskinesia; WAGR syndrome; Wilms’ tumor BIK BCL2-interacting killer (apoptosis-inducing) 22q13.31 rs926328; rs2235316 BLOC1S3 Biogenesis of lysosomal organelles complex-1, subunit 3 19q13.32 BRD1 Bromodomain containing 1 22q13.33 C3 Complement component 3 19p13.3-p13.2 Age-related macular degeneration 9; C3 deficiency; Susceptibility to atypical hemolytic uremic syndrome 5 C4B Complement component 4B (Chido blood group) 6p21.3 C4 deficiency C6orf217 Chromosome 6 open reading frame 217 6q23.3 rs1475069 C10orf26, OPAL1 Chromosome 10 open reading frame 26 10q24.32 C22DDEL S Chromosome 22q11.2 deletion syndrome, distal 22q11.2 CACNA1C Calcium channel, voltage-dependent, L type, alpha 1C subunit 12p13.3 rs1006737; rs22512119 CALR Calreticulin 19p13.3-p13.2 CCKAR Cholecystokinin A receptor 4p15.1-p15.2 rs1800857; 779T/C Copyright © 2013 SciRes. OPEN ACCESS
 R. Cacabelos et al. / Open Journal of Psychiatry 2 (2013) 46-139 51 Continued CDC42SE2 CDC42 small effector 2 5q31.1 CFB Complement factor B 6p21.3 Reduced risk of age-related macular degeneration; Susceptibility to atypical hemolytic uremic syndrome 4 CHAT Choline O-acetyltransferase 10q11.2 Alzheimer disease; Congenital myasthenic syndrome associated with episodic apnea; Myasthenic syndrome; Neurodegenerative diseases; Neurotoxicity syndromes; Parkinson’s disease (secondary); Peripheral nervous system diseases CHGA Chromogranin A (parathyroid secretory protein 1) 14q32 CHGB Chromogranin B (secretogranin 1) 20pter-p12 CHI3L1 Chitinase 3-like 1 (cartilage glycoprotein-39) 1q32.1 Effect of variation in CHI3L1 on serum YKL-40 level, risk of asthma, and lung function; Susceptibility to asthma-related traits 7 CHRFAM7 A CHRNA7 (cholinergic receptor, nicotinic, alpha 7, exons 5 - 10) and FAM7A (family with sequence similarity 7A, exons A-E) fusion 15q13.1 CHRNA2 Cholinergic receptor, nicotinic, alpha 2 (neuronal) 8p21 Nocturnal frontal lobe epilepsy type 4 CHRNA6 Cholinergic receptor, nicotinic, alpha 6 8p11.21 CHRNA7 Cholinergic receptor, nicotinic, alpha 7 15q14 rs3087454 CHRNB3 Cholinergic receptor, nicotinic, beta 3 8p11.2 CLDN5 Claudin 5 22q11.21 CLINT1 Clathrin interactor 1 5q33.3 CLOCK Clock homolog (mouse) 4q12 CLU Clusterin 8p21-p12 rs11136000 CMYA5 Cardiomyopathy associated 5 5q14.1 rs10043986; rs4704591 CNP 2’,3’-cyclic nucleotide 3’ phosphodiesterase 17q21 CNR1 Cannabinoid receptor 1 (brain) 6q14-q15 Alzheimer disease; Central nervous system diseases; Cocaine-related disorders; Muscle spasticity; Neurodegenerative diseases; Neurotoxicity syndromes; Substance withdrawal syndrome CNR2 Cannabinoid receptor 2 (macrophage) 1p36.11 CNTNAP2 Contactin associated protein-like 2 7q35 Cortical dysplasia focal epilepsy syndrome; Autism (susceptibility); Pitt-Hopkins-like syndrome Anxiety disorders; Autistic disorder; Bipolar disorder; Depressive disorder; DiGeorge syndrome; Migraine; Mood disorders; Nervous system diseases; Panic disorder; Obsessive-compulsive disorder; Parkinson’s disease; Prostatic neoplasms COMT Catechol-O-methyltransferase 22q11.21 rs4680; rs6267; rs737865 rs7378-rs4680- rs165599 rs2075507 rs362204 Susceptibility to panic disorder CPLX2 Complexin 2 5q35.2 rs3822674 CRP C-reactive protein, pentraxin-related 1q21-q23 Atherosclerosis; Cardiovascular disease; Cerebrovascular disease; Coronary disease; Diabetes mellitus (type 2); Heart diseases; Heart failure; Heart injuries; Hepatocellular carcinoma; Inflammation; Lung neoplasms; Lupus erythematosus; Malaria; Pasteurellaceae infections; Stroke; Systemic lupus erythematosus; Thrombosis CSF2RA Colony stimulating factor 2 receptor, alpha, low-affinity (granulocyte-macrophage) Xp22.32 and Yp11.3 Acute M2 type myeloid leukemia; Pulmonary alveolar proteinosis Copyright © 2013 SciRes. OPEN ACCESS
 R. Cacabelos et al. / Open Journal of Psychiatry 2 (2013) 46-139 52 Continued CSF2RB Colony stimulating factor 2 receptor, beta, low-affinity (granulocyte-macrophage) 22q13.1 Pulmonary alveolar proteinosis CSMD1 KIAA1890 Cub and Sushi multiple domains 1 8p23.2 CTL4 cytotoxic T-lymphocyte-associated protein 4 2q33 rs231779; rs16840252 CTSK cathepsin K 1q21 Pycnodysostosis CYBB Cytochrome b-245, beta polypeptide Xp21.1 CYP1A2 Cytochrome P450, family 1, subfamily A, polypeptide 2 15q24.1 Experimental liver cirrhosis; Experimental liver neoplasms; Experimental neoplasms; Gastric adenocarcinoma; Gastrointestinal neoplasms; Hepatocellular carcinoma; Leflunomide-induced toxicity; Methemoglobinemia; Myocardial infarction; Liver diseases; Neoplasms; Susceptibility to tobacco addiction; Tardive dyskinesia; Tobacco use disorder CYP3A4/5 Cytochrome P450, family 3, subfamily A, polypeptide 4/5 7q21.1 Acute hypoxia; Acute lymphoblastic leukemia; Acute myeloid leukemia; Age-related metabolism variation; Alcoholism; Allergic rhinitis; Alveolar echinococcosis; Antiplatelet drug resistance; Antiretroviral-antineoplastic drug interactions; Atherosclerosis; Azoospermia; Balkan endemic nephropathy; Barrett’s metaplasia; Benign prostate hyperplasia; Bile acid metabolism; Bladder cancer; Blood pressure; Brain tumors; Breast cancer; Breast neoplasms; Cancer; Celiac disease; Cerebrotendinous xanthomatosis; Cholestasis; Cholesterol homeostasis; Chronic hepatitis C; Cirrhosis; Colon adenoma; Colorectal cancer; Colorectal liver metastases; Crohn’s disease; Cushing’s syndrome; Cystic fibrosis; Depression; Depressive disorder; Diabetes mellitus (type 2); Diffuse panbronchiolitis; Drug-induced liver injury; Drug-virus interaction; Ehrlichiosis; Endometrial cancer; Epilepsy; Erectile dysfunction; Esophageal squamous cell carcinoma; Food-drug interactions; Gastric tumors; Gingival hyperplasia; Glucocorticoid-induced hypertension in children with acute lymphoblastic leukemia; Headache; Helicobacter pylori; Hepatic steatosis; Hepatitis; Hepatitis B infection; Hepatocellular tumors; Hodgkin’s disease; Hodgkin’s lymphoma; Hypertension; Hypothermia; Inflammatory bowel diseases; Lactobacillus rhamnosus; Leukemia; Liver cancer; Liver diseases; Liver neoplasms; Lung cancer; Lung neoplasms; Lymphocytes; Malaria; Menopause; Menstruation; Metabolic syndrome; Metabolic syndrome X; Migraine; Multiple myeloma; Myocardial infarction; Myocardial ischemia; Nasopharyngeal carcinoma; Neuropathic pain; Non-alcoholic fatty liver disease; Non-small cell lung cancer; Non-small cell lung carcinoma; Onychomycosis; Osteomalacia; Osteonecrosis; Osteosarcoma; Ovarian cancer; Ovarian neoplasms; Oxidative stress; Parkinson’s disease; Porphyrias; Precocious puberty; Pregnancy; Pregnancy complications; Primary biliary cirrhosis; Proctitis; Prostate cancer; Prostatic hyperplasia; Prostatic neoplasms; QT interval prolongation; Renal cancer; Renal cell carcinoma; Rhabdomyolysis; Schizophrenia; Squamous cell carcinoma of the larynx and hypopharynx; Stroke; T-cell lymphomas; Testicular germ cell cancer; Thalassemia; Torsades de pointes; Tuberculosis; Veno-occlusive disease; Venous thromboembolism; Venous thrombosis DAAM2 Dishevelled associated activator of morphogenesis 2 6p21.2 Copyright © 2013 SciRes. OPEN ACCESS
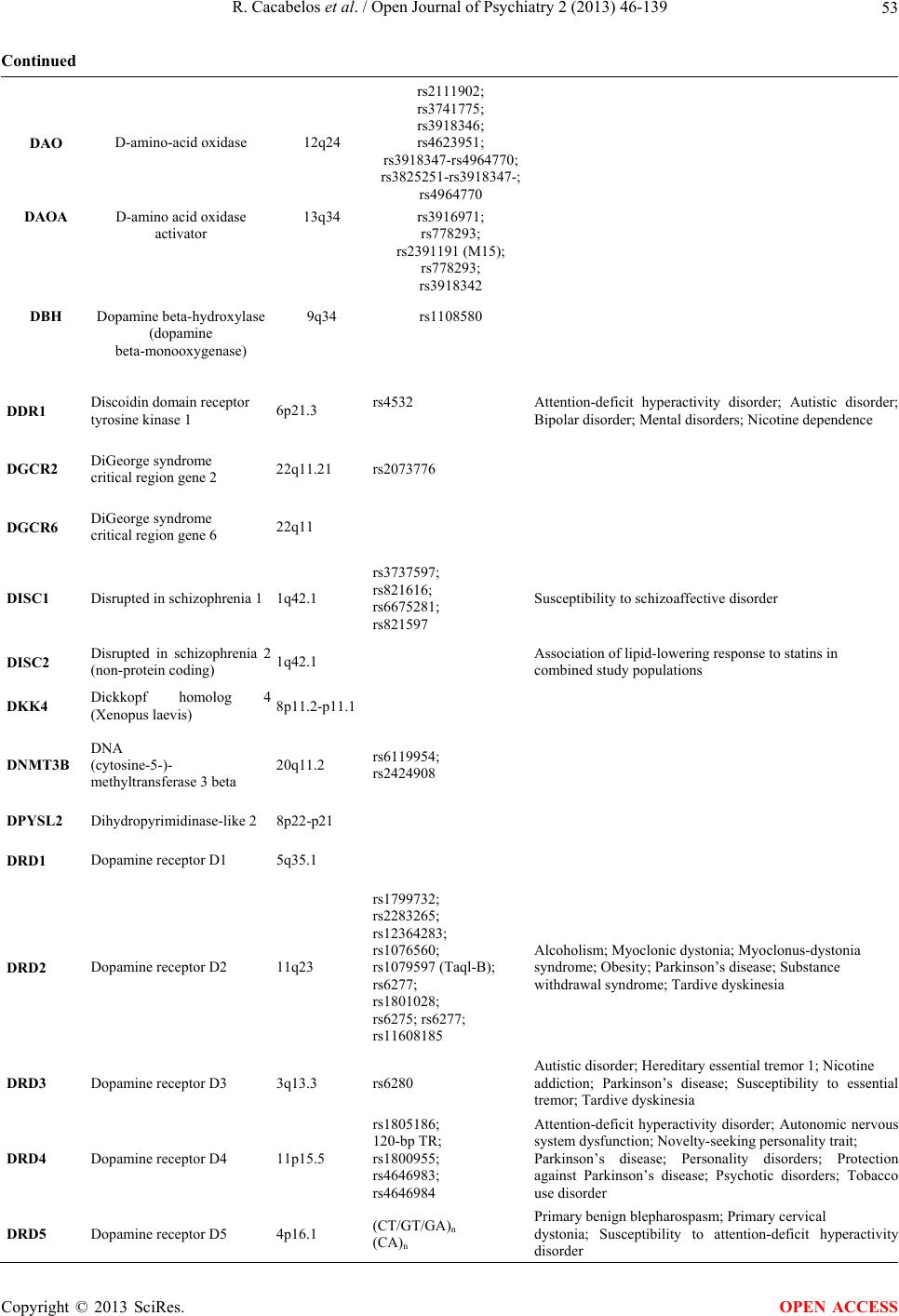 R. Cacabelos et al. / Open Journal of Psychiatry 2 (2013) 46-139 53 Continued DAO D-amino-acid oxidase 12q24 rs2111902; rs3741775; rs3918346; rs4623951; rs3918347-rs4964770; rs3825251-rs3918347-; rs4964770 DAOA D-amino acid oxidase activator 13q34 rs3916971; rs778293; rs2391191 (M15); rs778293; rs3918342 DBH Dopamine beta-hydroxylase (dopamine beta-monooxygenase) 9q34 rs1108580 DDR1 Discoidin domain receptor tyrosine kinase 1 6p21.3 rs4532 Attention-deficit hyperactivity disorder; Autistic disorder; Bipolar disorder; Mental disorders; Nicotine dependence DGCR2 DiGeorge syndrome critical region gene 2 22q11.21 rs2073776 DGCR6 DiGeorge syndrome critical region gene 6 22q11 DISC1 Disrupted in schizophrenia 1 1q42.1 rs3737597; rs821616; rs6675281; rs821597 Susceptibility to schizoaffective disorder DISC2 Disrupted in schizophrenia 2 (non-protein coding) 1q42.1 Association of lipid-lowering response to statins in combined study populations DKK4 Dickkopf homolog (Xenopus laevis) 8p11.2-p11.1 DNMT3B DNA (cytosine-5-)- methyltransferase 3 beta 20q11.2 rs6119954; rs2424908 DPYSL2 Dihydropyrimidinase-like 2 8p22-p21 DRD1 Dopamine receptor D1 5q35.1 DRD2 Dopamine receptor D2 11q23 rs1799732; rs2283265; rs12364283; rs1076560; rs1079597 (Taql-B); rs6277; rs1801028; rs6275; rs6277; rs11608185 Alcoholism; Myoclonic dystonia; Myoclonus-dystonia syndrome; Obesity; Parkinson’s disease; Substance withdrawal syndrome; Tardive dyskinesia DRD3 Dopamine receptor D3 3q13.3 rs6280 Autistic disorder; Hereditary essential tremor 1; Nicotine addiction; Parkinson’s disease; Susceptibility to essential tremor; Tardive dyskinesia DRD4 Dopamine receptor D4 11p15.5 rs1805186; 120-bp TR; rs1800955; rs4646983; rs4646984 Attention-deficit hyperactivity disorder; Autonomic nervous system dysfunction; Novelty-seeking personality trait; Parkinson’s disease; Personality disorders; Protection against Parkinson’s disease; Psychotic disorders; Tobacco use disorder DRD5 Dopamine receptor D5 4p16.1 (CT/GT/GA)n (CA)n Primary benign blepharospasm; Primary cervical dystonia; Susceptibility to attention-deficit hyperactivit disorder Copyright © 2013 SciRes. OPEN ACCESS
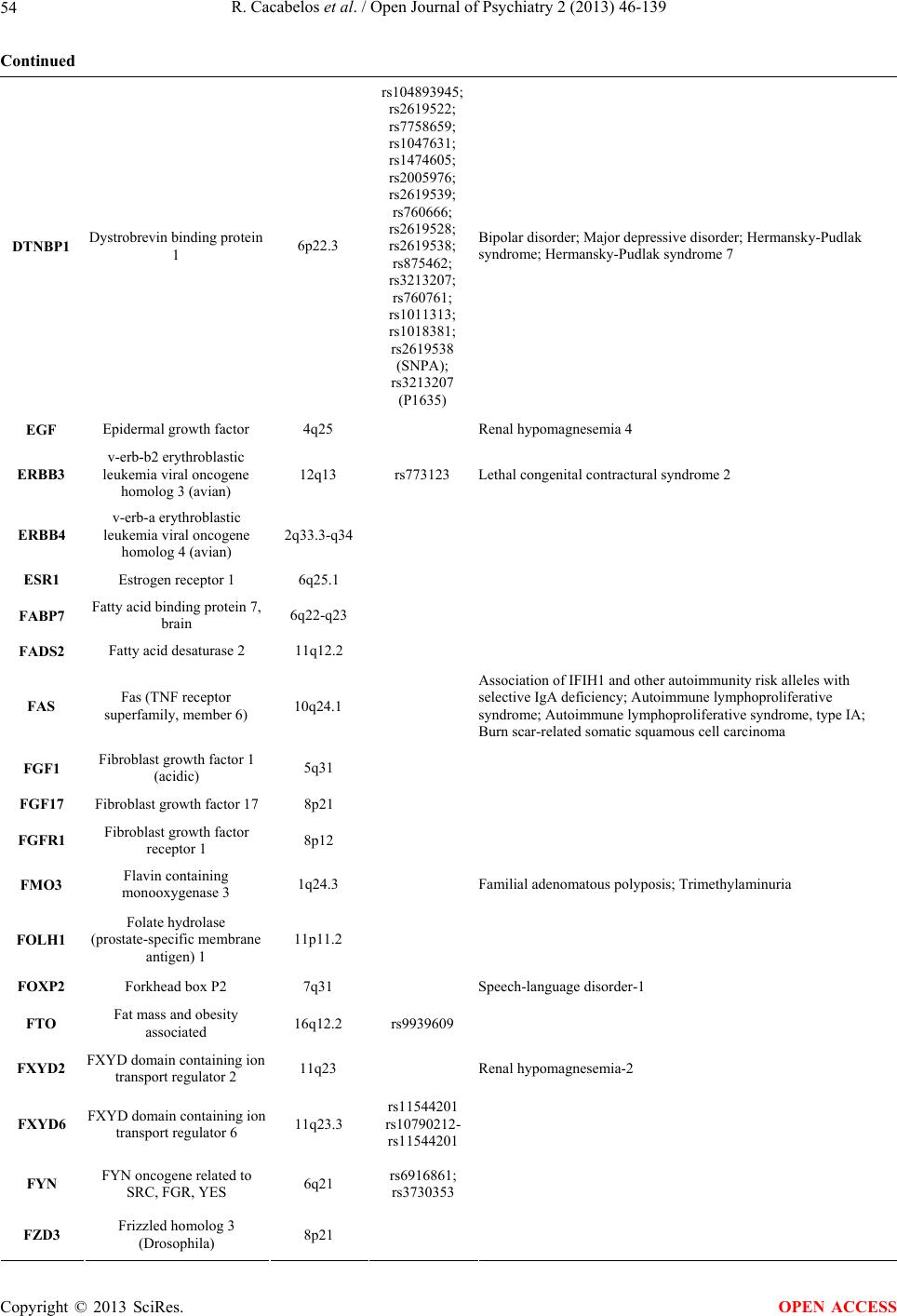 R. Cacabelos et al. / Open Journal of Psychiatry 2 (2013) 46-139 54 Continued DTNBP1 Dystrobrevin binding protein 1 6p22.3 rs104893945; rs2619522; rs7758659; rs1047631; rs1474605; rs2005976; rs2619539; rs760666; rs2619528; rs2619538; rs875462; rs3213207; rs760761; rs1011313; rs1018381; rs2619538 (SNPA); rs3213207 (P1635) Bipolar disorder; Major depressive disorder; Hermansky-Pudlak syndrome; Hermansky-Pudlak syndrome 7 EGF Epidermal growth factor 4q25 Renal hypomagnesemia 4 ERBB3 v-erb-b2 erythroblastic leukemia viral oncogene homolog 3 (avian) 12q13 rs773123 Lethal congenital contractural syndrome 2 ERBB4 v-erb-a erythroblastic leukemia viral oncogene homolog 4 (avian) 2q33.3-q34 ESR1 Estrogen receptor 1 6q25.1 FABP7 Fatty acid binding protein 7, brain 6q22-q23 FADS2 Fatty acid desaturase 2 11q12.2 FAS Fas (TNF receptor superfamily, member 6) 10q24.1 Association of IFIH1 and other autoimmunity risk alleles with selective IgA deficiency; Autoimmune lymphoproliferative syndrome; Autoimmune lymphoproliferative syndrome, type IA; Burn scar-related somatic squamous cell carcinoma FGF1 Fibroblast growth factor 1 (acidic) 5q31 FGF17 Fibroblast growth factor 17 8p21 FGFR1 Fibroblast growth factor receptor 1 8p12 FMO3 Flavin containing monooxygenase 3 1q24.3 Familial adenomatous polyposis; Trimethylaminuria FOLH1 Folate hydrolase (prostate-specific membrane antigen) 1 11p11.2 FOXP2 Forkhead box P2 7q31 Speech-language disorder-1 FTO Fat mass and obesity associated 16q12.2 rs9939609 FXYD2 FXYD domain containing ion transport regulator 2 11q23 Renal hypomagnesemia-2 FXYD6 FXYD domain containing ion transport regulator 6 11q23.3 rs11544201 rs10790212- rs11544201 FYN FYN oncogene related to SRC, FGR, YES 6q21 rs6916861; rs3730353 FZD3 Frizzled homolog 3 (Drosophila) 8p21 Copyright © 2013 SciRes. OPEN ACCESS
 R. Cacabelos et al. / Open Journal of Psychiatry 2 (2013) 46-139 55 Continued GABBR1 Gamma-aminobutyric acid (GABA) B receptor, 1 6p21.31 GABRB1 Gamma-aminobutyric acid (GABA) A receptor, beta 1 4p12 GABRB2 Gamma-aminobutyric acid (GABA) A receptor, beta 2 5q34 rs1816072 GABRG2 Gamma-aminobutyric acid (GABA) A receptor, gamma 2 5q34 Childhood absence epilepsy; Childhood absence epilepsy 2; Familial febrile convulsions 8; Generalized epilepsy with febrile seizures plus; Generalized epilepsy with febrile seizures plus, type 3; Severe myoclonic epilepsy of infancy; Susceptibility to childhood absence epilepsy 2 GAD1 Glutamate decarboxylase 1 (brain, 67 kDa) 2q31 Autosomal recessive symmetric spastic cerebral palsy GAD2 Glutamate decarboxylase 2 (pancreatic islets and brain, 65 kDa) 10p11.23 GC Group-specific component (vitamin D binding protein) 4q12-q13 Susceptibility to Graves’ disease 3 GCLM Glutamate-cysteine ligase, modifier subunit 1p22.1 Susceptibility to myocardial infarction GJA8 Gap junction protein, alpha 8, 50 kDa 1q21.1 Cataract-microcornea syndrome; Nuclear progressive cataract; Nuclear pulverulent cataract; Zonular pulverulent cataract 1 GLS Glutaminase 2q32-q34 GLUL Glutamate-ammonia ligase 1q31 GNB1L Guanine nucleotide binding protein (G protein), beta polypeptide 1-like 22q11.2 GRIA1 Glutamate receptor, ionotropic, AMPA 1 5q31.1 GRIA4 Glutamate receptor, ionotrophic, AMPA 4 11q22 GRID1 Glutamate receptor, ionotropic, delta 1 10q22 rs3814614; rs10749535; rs11201985 GRIK3 Glutamate receptor, ionotropic, kainate 3 1p34-p33 rs6691840 GRIK4 Glutamate receptor, ionotropic, kainate 4 11q22.3 rs4935752; rs6589846; rs4430518 Bipolar disorder; Depression GRIK5 Glutamate receptor, ionotropic, kainate 5 19q13.2 GRIN1 Glutamate receptor, iono- tropic, N-methyl D-aspartate 1 9q34.3 12p12 rs1019385 GRIN2B Glutamate receptor, ionotropic, N-methyl D-aspartate 2B rs7301328 GRIN2D Glutamate receptor, ionotropic, N-methyl D-aspartate 2D 19q13.1-qter GRM3 Glutamate receptor, metabotropic 3 7q21.1-q21.2 GRM4 Glutamate receptor, metabotropic 4 6p21.3 Copyright © 2013 SciRes. OPEN ACCESS
 R. Cacabelos et al. / Open Journal of Psychiatry 2 (2013) 46-139 56 Continued GRM7 Glutamate receptor, metabotropic 7 3p26.1-p25.1 rs12491620; rs1450099 GPR109A G protein-coupled receptor 109A 12q24.31 GPR109B G protein-coupled receptor 109B 12q24.31 GSK3B Glycogen synthase kinase 3 beta 3q13.3 (CAA)n repeat polymorphisms; -1727 A/T Alzheimer disease; Bipolar disorder; Cancer; Colonic neoplasms; Colorectal neoplasms; Diabetes (type 2); Diabetes mellitus; Depression; Insulin resistance; Lithium sensitivity; Major depression; Myeloid/lymphoid or mixed lineage leukemia; Neurodegenerative diseases; Parkinsonian disorders; Tauopathy GSTM1 Glutathione S-transferase mu 1 1p13.3 GSTM1*0 Acute lymphoblastic leukemia; Acute myeloid leukemia; Acute promyelocytic leukemia; Adverse drug reactions to azathioprine; Aflatoxin-related hepatocarcinogenesis; Aplastic anemia; Autism; Autistic disorder; Azathioprine adverse effects; Benzene toxicity; Basal cell nervous syndrome; Basal cell carcinoma; Bladder cancer; Bleomycin moderate toxicity; Breast cancer; Busulfan pharmacokinetics; Carbamazepine-induced mild hepatotoxicity; Carcinogen toxicity; Colorectal cancer; Colorectal neoplasms; Coronary artery disease; Cytochrome P450 1A1 gene transcription; Diabetes mellitus (type 2); DNA damage after exposure to hydroquinone; Diabetic nephropathy; GSTM1 methylation; Head and neck neoplasms; Hepatocellular carcinoma; Leukemia; Hydroquinone-induced DNA damage; High inducibility of cytochrome P450 1A1 gene transcription; Liver cancer; Liver neoplasms; Lung cancer; Lung neoplasms; Lymphoblastic leukemia; Mesothelioma; Methotrexate toxicity; Myelodisplastic syndrome; Myeloid leukemia; Prostate cancer; Prostatic neoplasms; Raynaud’s disease; Responses to environmental and industrial carcinogens; Second primary neoplasms; Skin diseases; Systemic lupus erythematosus; Tacrine-induced hepatotoxicity; Testicular neoplasms; Thimerosal sensitization; Urinary bladder neoplasms; Troglitazone-induced liver failure GSTP1 Glutathione S-transferase pi 1 11q13 GSTT1 Glutathione S-transferase theta 1 22q11.23 GULP1 GULP, engulfment adaptor PTB domain containing 1 2q32.3-q33rs2004888 GWA 10q2613 rs17101921 GWA 11p141 rs1602565 GWA 16p1312 rs71992086 GWA_10q2 6.13 rs17101921 GWA_11p1 4.1 rs1602565 GWA_16p1 3.12 rs7192086 HINT1 Histidine triad nucleotide binding protein 1 5q31.2 HLA-A Major histocompatibility complex, class I, A 6p21.3 HLA-B Major histocompatibility complex, class I, B 6p21.3 HLA-C Major histocompatibility complex, class I, C 6p21.3 Copyright © 2013 SciRes. OPEN ACCESS
 R. Cacabelos et al. / Open Journal of Psychiatry 2 (2013) 46-139 57 Continued HLA-DQA1 Major histocompatibility complex, class II, DQ alpha 1 6p21.3 Asthma; Celiac disease; Diabetes (type 1); Gluten-sensitive enteropathy; HLA-DQA1 differential expression; Lung adenocarcinoma; Metamizol-related agranulocytosis; Oligoarticular juvenile idiopathic arthritis; Rheumatoid arthritis; Susceptibility to onchocerciasis; Toxoplasmic encephalitis; Ximelagratan sensitivity HLA-DQB Major histocompatibility complex, class II, DQ beta 1 6p21.3 HLA-DRB1 Major histocompatibility complex, class II, DR beta 1 6p21.3 HLA-E Major histocompatibility complex, class I, 6p21.3 Bipolar disorder HIST1H2BJ Histone cluster 1, H2bj 6p22.1 rs6913660 HOMER Homer homolog 2 (Drosophila) 15q24.3 rs17158184; rs2306428 HP Haptoglobin 16q22.1 Hp1/2 Anemia; Atherosclerosis; Autoimmune diseases; Breast neoplasms; Cardiovascular disease; Chronic hepatitis B; Chronic hepatitis C; Coronary atherosclerosis; Crohn’s disease; Diabetic vascular disease; Dyslipidemias; Diabetes mellitus (type 1); Diabetes mellitus (type 2); Diabetic angiopathy; Diabetic nephropathy; Female genital neoplasms; Hemochromatosis; Hepatitis B; HIV infections; Hypersensitivity; Hypertension; Leukemia; Malaria; Myocardial infarction; Nasopharingeal carcinoma; Parkinson’s disease; Pasteurellaceae infections; Pregnancy-induced hypertension; Primary sclerosis cholangitis; Retinal detachment; Sickle cell anemia; Tuberculosis; Trachoma HRH1 Histamine receptor H1 3p25 Allergy; Allergic contact dermatitis; Angioedema; Asthma; Atopic dermatitis; Bronchial hyperreactivity; Hypersensitivity; Inflammation; Learning disorders; Long QT syndrome; Memory disorders; Mental disorders; Perennial allergic rhinitis; Pruritus; Pulmonary eosinophilia; Respiratory hypersensitivity; Respiratory tract diseases; Rhinitis; Urticaria; Sinusitis; Skin disorders; Sneezing; Seasonal allergic rhinitis; Seizures HSPA1B Heat shock 70kDa protein 1B 6q21.3 rs539689 HTR1A 5-Hydroxytryptamine (serotonin) receptor 1A 5q11.2-q13 Alzheimer disease; Antidepressant sensitivity; Anxiety disorders; Depression; Macular degeneration; Major depression; Mental disorders; Neuroleptic-related weight gain in schizophrenia; Panic disorder; Personality disorders; Psychotic disorders; Schizophrenia-related weight gain; Sudden infant death HTR2A 5-Hydroxytryptamine (serotonin) receptor 2A 13q14-q21 rs6313; rs6314; rs6311 Alcohol dependence; Alzheimer disease; Anorexia nervosa; Antidepressant-related response; Antipsychotic-related response; Asperger’s syndrome; Attention-deficit hyperactivity disorder; Autistic disorder; Bipolar disorder; Clozapine-induced weight gain; Depression; Depressive disorder; Major depression; Major depressive disorder; Memory performance; Mental disorders; Mental retardation; Migraine; Mood disorders; Neuroleptic-induced weight gain; Neuroleptic-related response; Neurotoxicity syndromes; Obsessive-compulsive disorder; Personality disorders; Pervasive child development disorders; Psychotic disorders; Substance abuse; Response to citalopram therapy in major depressive disorder; Tardive dyskinesia HTR3A 5-Hydroxytryptamine (serotonin) receptor 3A 11q23.1 HTR4 5-Hydroxytryptamine (serotonin) receptor 4 5q31-q33 IDE Insulin-degrading enzyme 10q23-q25 Copyright © 2013 SciRes. OPEN ACCESS
 R. Cacabelos et al. / Open Journal of Psychiatry 2 (2013) 46-139 58 Continued IFNG Interferon, gamma 12q14 rs62559044 AIDS; Allergic contact dermatitis; Aortic aneurysm; Aplastic anemia; Appendicitis; Asthma; Atherosclerosis; Autistic disorder; Crohn’s disease; Drug-induced liver injury; Entamebiasis; Hepatitis; Hepatitis C; Hepatocellular carcinoma; Hepatomegaly; Inflammation; Job’s syndrome; Kidney angiomyolipoma; Kidney failure; Myocardial infarction; Liver neoplasms; Lupus nephritis; Multiple sclerosis; Liver cirrhosis; Liver diseases; Oral submucous fibrosis; Renal cell carcinoma; Tuberculosis; Tuberous sclerosis; Susceptibility to human immunodeficiency virus type 1 IGHA1 Immunoglobulin heavy constant alpha 1 14q32.33 IL1A Interleukin 1, alpha 2q14 IL1B Interleukin 1, beta 2q14 rs1143634 rs16944 Alzheimer disease; Ankylosing spondylitis; Arsenic poisoning; Body fat mass; Breast neoplasms; Bronchopulmonary dysplasia; Colon cancer; Colonic neoplasms; Diabetic nephropathy; Distal interphalangeal joint osteoarthritis; Entamebiasis; Experimental liver cirrhosis; Fever; Gastric cancer; Gastric cancer and Helicobacter pylori; Glioblastoma; IgA nephropathy; Inflammation; Inflammatory bowel disease; Lung diseases; Major depression; Major depressive disorder; Osteoporosis; Microvascular complications of diabetes; Multiple sclerosis; Nervous system diseases; Non-small cell lung cancer; Parkinson’s disease; Postmenopausal osteoporosis; Primary open-angle glaucoma; Pulmonary fibrosis; Skin diseases; Stomach neoplasms; Ulcerative colitis IL1RN Interleukin 1 receptor antagonist 2q14.2 Arsenic poisoning; Autistic disorder; Colonic neoplams; Chagas’ disease; Diabetes; Gastric cancer susceptibility after H. pylori infection; Generalized aggressive periodontitis; Inflammatory bowel disease; Interleukin 1 receptor antagonist deficiency; Memory disorders; Metabolic syndrome; Multiple sclerosis; Osteoarthritis; Osteoporosis; Prostatic neoplasms; Skin diseases; Stroke; Susceptibility to microvascular complications of diabetes 4; Tourette’s syndrome; Ulcer IL3 Interleukin 3 (colony-stimulating factor, multiple) 5q31.1 IL3RA Interleukin 3 receptor, alpha (low affinity) Xp22.3 or Yp11.3 rs6603272 IL4 Interleukin 4 5q31.1 Allergic contact dermatitis; Arthritis; Asthma; Atopic dermatitis; Autistic disorder; Autoimmune hypothyroidism; Bladder cancer; Chronic periodontitis; Common variable immunodeficiency; Graves’ disease; Glioblastoma; Hypersensitivity; Psoriasis; Subacute sclerosing panencephalitis; Systemic lupus erythematosus; Thromboembolic stroke IL10 Interleukin 10 1q31-q32 rs1800896; rs1800872 Acquired immunodeficiency syndrome; Alcoholic liver disease; Alzheimer disease; Appendicitis; Autistic disorder; Colitis; Coronary heart disease; Crohn’s disease; Diabetes mellitus (type 1); Enterocolitis; Entamebiasis; Experimental mammary neoplasms; Graft vs host disease (acute); Hepatitis B; Hepatocellular carcinoma; HIV infections; Inflammatory bowel disease; Irritable bowel syndrome; Kawasaki disease; Leprosy; Lung neoplasms; Malaria; Prostate cancer; Prostatic neoplasms; Psoriasis; Rheumatoid arthritis; Severe malaria anemia; Skin carcinomas; Skin neoplasms; Squamous cell carcinoma; Sudden infant death syndrome; Systemic lupus erythematosus; Trachoma; Ulcerative colitis IL12B Interleukin 12B (natural killer cell stimulatory factor 2, cytotoxic lymphocyte maturation factor 2, p40) 5q31.1-q33.1 Asthma; Atopic dermatitis; Bacillus Calmette-Guérin and Salmonella infection; Colorectal cancer; Crohn’s disease; Diabetes mellitus (type 1); Familial atypical mycobacteriosis; Gastric cancer; Hypothermia; Leprosy; Malaria; Psoriasis; Psoriasis vulgaris; Psoriatic arthritis; Salmonella infection; Tuberculosis; Ulcerative colitis IL18 Interleukin 18 (interferon-gamma-inducing factor) 11q22.2-q22.3 Copyright © 2013 SciRes. OPEN ACCESS
 R. Cacabelos et al. / Open Journal of Psychiatry 2 (2013) 46-139 59 Continued IL18R1 Interleukin 18 receptor 1 2q12 IL18RAP Interleukin 18 receptor accessory protein 2q12 IPO5 Importin 5 13q32.2 ITIH3/4 Inter-alpha (globulin) inhibitor, H3/4 polypeptides 3p21.1 JARID2 Jumonji, AT rich interactive domain 2 6p24-p23 rs9654600; rs2235258 KCNH2 Potassium voltage-gated channel, subfamily H (eag-related), member 2 7q36.1 Arrhythmia; Bradycardia-induced long QT syndrome; Drug-associated torsades de pointes; Long QT syndrome; Long QT syndrome 1/2; Long QT syndrome 2; Long QT syndrome 2/3; Long QT syndrome 2/5; Long QT syndrome 2/9; Nonfamiliar atrial fibrillation (AF); Schizophrenia; Short QT syndrome 1; Torsades de pointes LEPR Leptin receptor 1p31 Acute lymphoblastic leukemia; Diabetes; Diabetes mellitus (type 2); Glucocorticoid-induced hypertension; Hypogonadism; Impaired glucose tolerance; Low bone mineral content; Morbid obesity; Obesity; Osteoporosis; Risk of metabolic syndrome, diabetes or vascular disease LGR4 Leucine-rich repeat containing G protein-coupled receptor 4 11p14-p13 LTA Lymphotoxin alpha (TNF superfamily, member 1) 6p21.3 Susceptibility to leprosy 4; Susceptibility to myocardial infarction; Susceptibility to psoriatic arthritis MAGI1 Membrane associated guanylate kinase, WW and PDZ domain containing 1 3p14.1 MAGI2 Membrane associated guanylate kinase, WW and PDZ domain containing 2 7q21 MAGI3 Membrane associated guanylate kinase, WW and PDZ domain containing 3 1p12-p11.2 MBP Myelin basic protein 18q23 MC5R Melanocortin 5 receptor 18p11.2 MCHR1 Melanin-concentrating hormone receptor 1 22q13.2 MCHR2 Melanin-concentrating hormone receptor 2 6q16 MCTP2 Multiple C2 domains, transmembrane 2 15q26.2 MDGA1 MAM domain containing glycosylphosphatidylinositol anchor 1 6p21 rs11759115 ME2 Malic enzyme 2, NAD(+)-dependent, mitochondrial 18q21 MED12 Mediator complex subunit 12 Xq13 FG syndrome 1; Lujan-Fryns syndrome; Opitz-Kaveggia syndrome MEGF10 Multiple EGF-like-domains 10 5q33 MICB MHC class I polypeptide-related sequence B 6p21.3 MLC1 Megalencephalic leukoencephalopathy with subcortical cysts 1 22q13.33 Megalencephalic leukoencephalopathy with subcortical cysts Copyright © 2013 SciRes. OPEN ACCESS
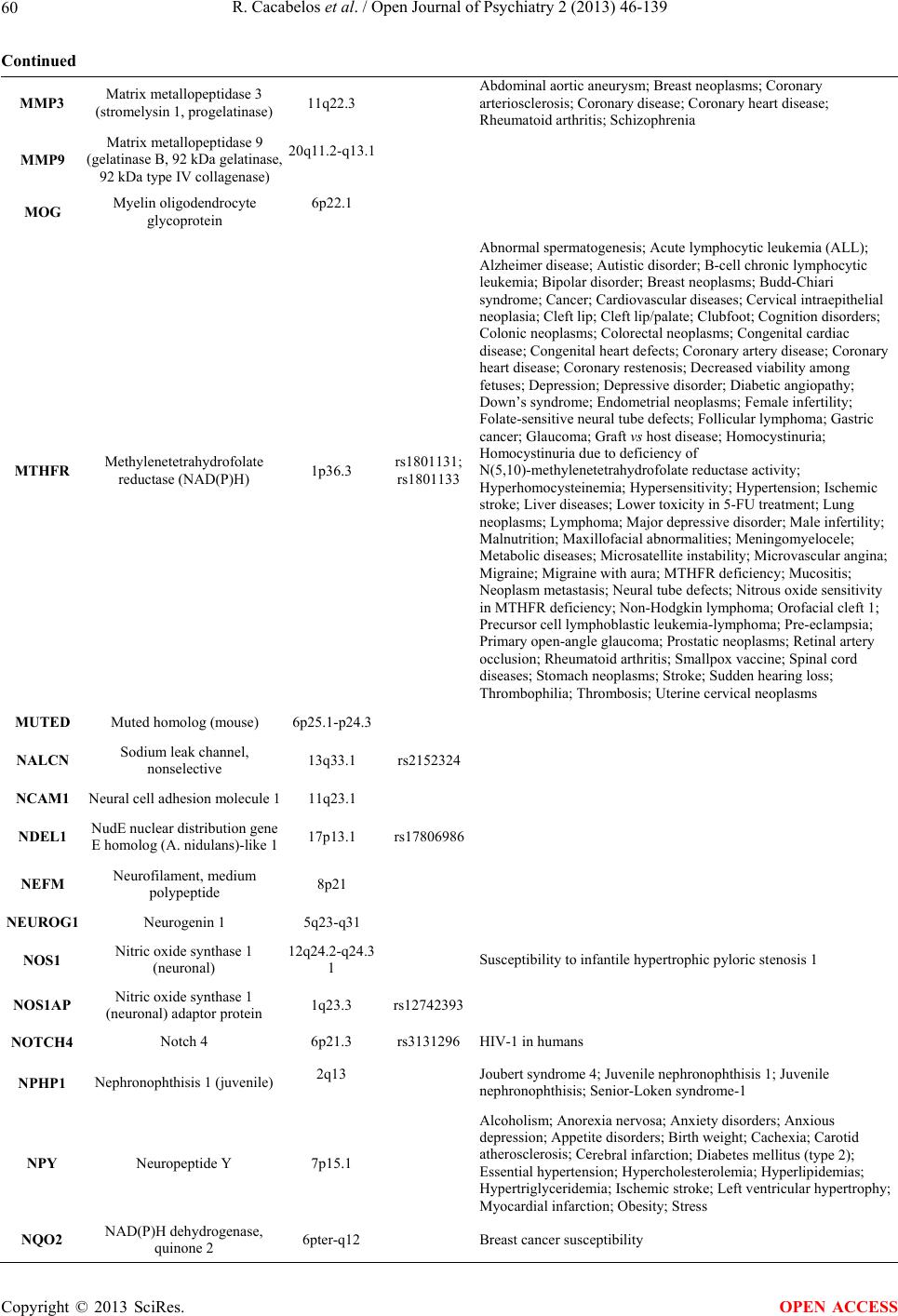 R. Cacabelos et al. / Open Journal of Psychiatry 2 (2013) 46-139 60 Continued MMP3 Matrix metallopeptidase 3 (stromelysin 1, progelatinase) 11q22.3 Abdominal aortic aneurysm; Breast neoplasms; Coronary arteriosclerosis; Coronary disease; Coronary heart disease; Rheumatoid arthritis; Schizophrenia MMP9 Matrix metallopeptidase 9 (gelatinase B, 92 kDa gelatinase, 92 kDa type IV collagenase) 20q11.2-q13.1 MOG Myelin oligodendrocyte glycoprotein 6p22.1 MTHFR Methylenetetrahydrofolate reductase (NAD(P)H) 1p36.3 rs1801131; rs1801133 Abnormal spermatogenesis; Acute lymphocytic leukemia (ALL); Alzheimer disease; Autistic disorder; B-cell chronic lymphocytic leukemia; Bipolar disorder; Breast neoplasms; Budd-Chiari syndrome; Cancer; Cardiovascular diseases; Cervical intraepithelial neoplasia; Cleft lip; Cleft lip/palate; Clubfoot; Cognition disorders; Colonic neoplasms; Colorectal neoplasms; Congenital cardiac disease; Congenital heart defects; Coronary artery disease; Coronary heart disease; Coronary restenosis; Decreased viability among fetuses; Depression; Depressive disorder; Diabetic angiopathy; Down’s syndrome; Endometrial neoplasms; Female infertility; Folate-sensitive neural tube defects; Follicular lymphoma; Gastric cancer; Glaucoma; Graft vs host disease; Homocystinuria; Homocystinuria due to deficiency of N(5,10)-methylenetetrahydrofolate reductase activity; Hyperhomocysteinemia; Hypersensitivity; Hypertension; Ischemic stroke; Liver diseases; Lower toxicity in 5-FU treatment; Lung neoplasms; Lymphoma; Major depressive disorder; Male infertility; Malnutrition; Maxillofacial abnormalities; Meningomyelocele; Metabolic diseases; Microsatellite instability; Microvascular angina; Migraine; Migraine with aura; MTHFR deficiency; Mucositis; Neoplasm metastasis; Neural tube defects; Nitrous oxide sensitivity in MTHFR deficiency; Non-Hodgkin lymphoma; Orofacial cleft 1; Precursor cell lymphoblastic leukemia-lymphoma; Pre-eclampsia; Primary open-angle glaucoma; Prostatic neoplasms; Retinal artery occlusion; Rheumatoid arthritis; Smallpox vaccine; Spinal cord diseases; Stomach neoplasms; Stroke; Sudden hearing loss; Thrombophilia; Thrombosis; Uterine cervical neoplasms MUTED Muted homolog (mouse) 6p25.1-p24.3 NALCN Sodium leak channel, nonselective 13q33.1 rs2152324 NCAM1 Neural cell adhesion molecule 1 11q23.1 NDEL1 NudE nuclear distribution gene E homolog (A. nidulans)-like 1 17p13.1 rs17806986 NEFM Neurofilament, medium polypeptide 8p21 NEUROG1 Neurogenin 1 5q23-q31 NOS1 Nitric oxide synthase 1 (neuronal) 12q24.2-q24.3 1 Susceptibility to infantile hypertrophic pyloric stenosis 1 NOS1AP Nitric oxide synthase 1 (neuronal) adaptor protein 1q23.3 rs12742393 NOTCH4 Notch 4 6p21.3 rs3131296 HIV-1 in humans NPHP1 Nephronophthisis 1 (juvenile) 2q13 Joubert syndrome 4; Juvenile nephronophthisis 1; Juvenile nephronophthisis; Senior-Loken syndrome-1 NPY Neuropeptide Y 7p15.1 Alcoholism; Anorexia nervosa; Anxiety disorders; Anxious depression; Appetite disorders; Birth weight; Cachexia; Carotid atherosclerosis; Cerebral infarction; Diabetes mellitus (type 2); Essential hypertension; Hypercholesterolemia; Hyperlipidemias; Hypertriglyceridemia; Ischemic stroke; Left ventricular hypertrophy; Myocardial infarction; Obesity; Stress NQO2 NAD(P)H dehydrogenase, quinone 2 6pter-q12 Breast cancer susceptibility Copyright © 2013 SciRes. OPEN ACCESS
 R. Cacabelos et al. / Open Journal of Psychiatry 2 (2013) 46-139 61 Continued NR4A2 Nuclear receptor subfamily 4, group A, member 2 2q22-q23 Parkinson’s disease NRG1 Neuregulin 1 8p12 rs2439272; rs35753505; rs473376; rs10503929; rs7825588; rs6994992; rs4623364 NRG2 Neuregulin 2 5q23-q33 NRG3 Neuregulin 3 10q22-q23 rs7825588; rs10883866; rs10748842; rs6584400 NRGN Neurogranin (protein kinase C substrate, RC3) 11q24 rs12807809; rs7113041 NRXN1 Neurexin 1 2p16.3 NTF3 Neurotrophin 3 12p13 NTNG1 Netrin G1 1p13.3 NTRK3 Neurotrophic tyrosine kinase, receptor, type 3 15q25 rs999905 rs4887348 NUMBL Numb homolog (Drosophila)-like 19q13.13-q13. 2 OLIG2 Oligodendrocyte lineage transcription factor 2 21q22.11 rs762237; rs2834072 OPCML Opioid binding protein/cell adhesion molecule-like 11q25 rs3016384Somatic epithelial ovarian cancer; Somatic ovarian cancer OXSR1 Oxidative-stress responsive 1 3p22.2 PALB2 Partner and localizer of BRCA2 16p12.2 PCM1 Pericentriolar material 1 8p22-p21.3 Papillary thyroid carcinoma PCNT Pericentrin 21q22.3 PDE4B Phosphodiesterase 4B, cAMP-specific 1p31 rs910694; rs741271 PDE4D Phosphodiesterase 4D, cAMP-specific 5q12 rs1120303 PDILM5 PDZ and LIM domain 5 4q22 PDE7B Phosphodiesterase 7B 6q23-q24 rs9389370 PGBD1 PiggyBac transposable element derived 1 6p22.1 rs13211507 PICALM Phosphatidylinositol binding clathrin assembly protein 11q14 rs3851179 PICK1 Protein interacting with PRKCA 1 22q13.1 PIK3C3 Phosphoinositide-3-kinase, class 3 18q12.3 PI4K2B Phosphatidylinositol 4-kinase type 2 beta 4p15.2 PLA2G4A Phospholipase A2, group IVA (cytosolic, calcium-dependent) 1q25 rs10798059Deficiency of phospholipase A2, group IV A PLAT Plasminogen activator, tissue 8p12 Familial hyperfibrinolysis, due to increased release of PLAT; Plasminogen activator deficiency Copyright © 2013 SciRes. OPEN ACCESS
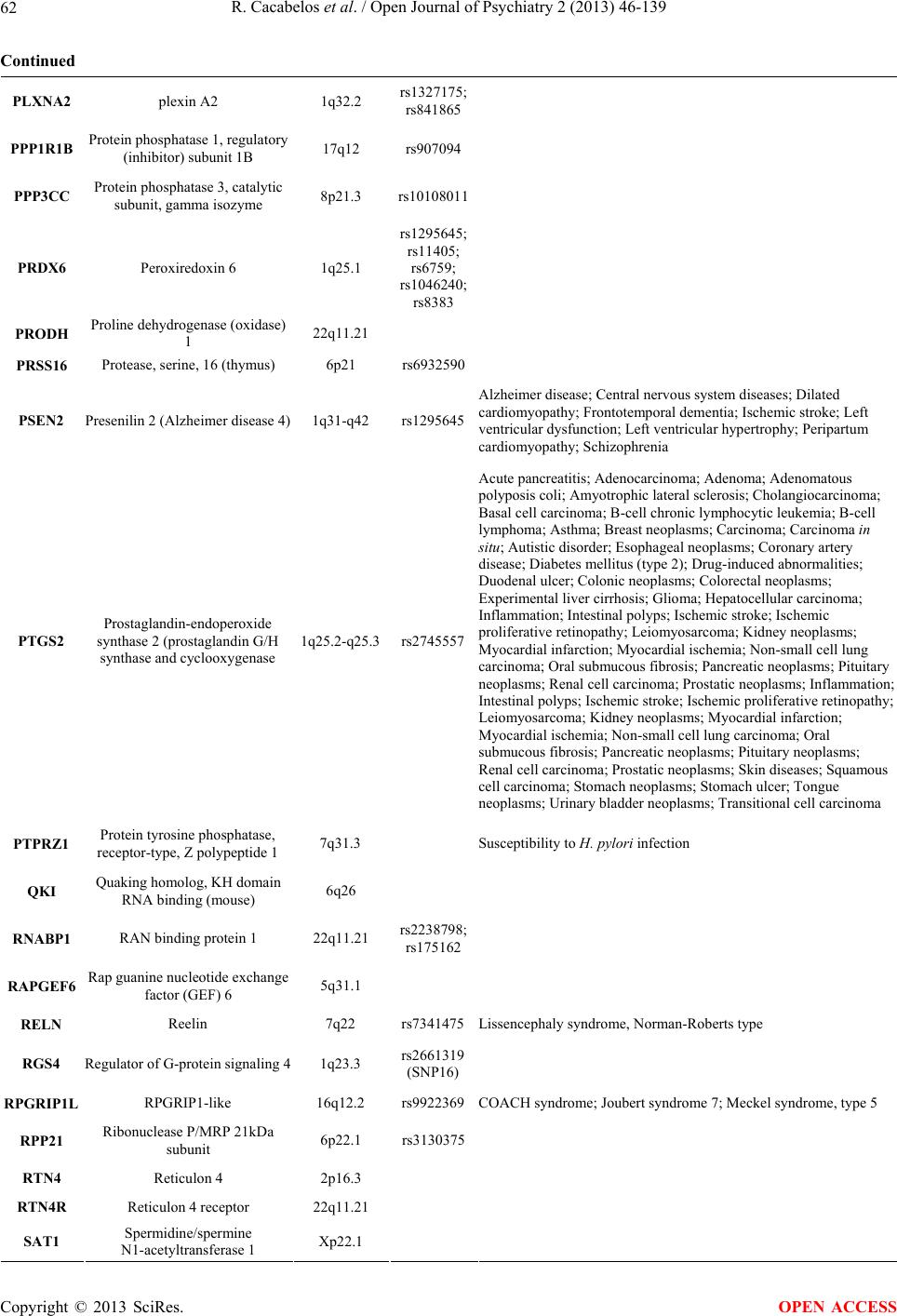 R. Cacabelos et al. / Open Journal of Psychiatry 2 (2013) 46-139 62 Continued PLXNA2 plexin A2 1q32.2 rs1327175; rs841865 PPP1R1B Protein phosphatase 1, regulatory (inhibitor) subunit 1B 17q12 rs907094 PPP3CC Protein phosphatase 3, catalytic subunit, gamma isozyme 8p21.3 rs10108011 PRDX6 Peroxiredoxin 6 1q25.1 rs1295645; rs11405; rs6759; rs1046240; rs8383 PRODH Proline dehydrogenase (oxidase) 1 22q11.21 PRSS16 Protease, serine, 16 (thymus) 6p21 rs6932590 PSEN2 Presenilin 2 (Alzheimer disease 4) 1q31-q42 rs1295645 Alzheimer disease; Central nervous system diseases; Dilated cardiomyopathy; Frontotemporal dementia; Ischemic stroke; Left ventricular dysfunction; Left ventricular hypertrophy; Peripartum cardiomyopathy; Schizophrenia PTGS2 Prostaglandin-endoperoxide synthase 2 (prostaglandin G/H synthase and cyclooxygenase 1q25.2-q25.3 rs2745557 Acute pancreatitis; Adenocarcinoma; Adenoma; Adenomatous polyposis coli; Amyotrophic lateral sclerosis; Cholangiocarcinoma; Basal cell carcinoma; B-cell chronic lymphocytic leukemia; B-cell lymphoma; Asthma; Breast neoplasms; Carcinoma; Carcinoma in situ; Autistic disorder; Esophageal neoplasms; Coronary artery disease; Diabetes mellitus (type 2); Drug-induced abnormalities; Duodenal ulcer; Colonic neoplasms; Colorectal neoplasms; Experimental liver cirrhosis; Glioma; Hepatocellular carcinoma; Inflammation; Intestinal polyps; Ischemic stroke; Ischemic proliferative retinopathy; Leiomyosarcoma; Kidney neoplasms; Myocardial infarction; Myocardial ischemia; Non-small cell lung carcinoma; Oral submucous fibrosis; Pancreatic neoplasms; Pituitary neoplasms; Renal cell carcinoma; Prostatic neoplasms; Inflammation; Intestinal polyps; Ischemic stroke; Ischemic proliferative retinopathy; Leiomyosarcoma; Kidney neoplasms; Myocardial infarction; Myocardial ischemia; Non-small cell lung carcinoma; Oral submucous fibrosis; Pancreatic neoplasms; Pituitary neoplasms; Renal cell carcinoma; Prostatic neoplasms; Skin diseases; Squamous cell carcinoma; Stomach neoplasms; Stomach ulcer; Tongue neoplasms; Urinary bladder neoplasms; Transitional cell carcinoma PTPRZ1 Protein tyrosine phosphatase, receptor-type, Z polypeptide 1 7q31.3 Susceptibility to H. pylori infection QKI Quaking homolog, KH domain RNA binding (mouse) 6q26 RNABP1 RAN binding protein 1 22q11.21 rs2238798; rs175162 RAPGEF6 Rap guanine nucleotide exchange factor (GEF) 6 5q31.1 RELN Reelin 7q22 rs7341475Lissencephaly syndrome, Norman-Roberts type RGS4 Regulator of G-protein signaling 4 1q23.3 rs2661319 (SNP16) RPGRIP1L RPGRIP1-like 16q12.2 rs9922369COACH syndrome; Joubert syndrome 7; Meckel syndrome, type 5 RPP21 Ribonuclease P/MRP 21kDa subunit 6p22.1 rs3130375 RTN4 Reticulon 4 2p16.3 RTN4R Reticulon 4 receptor 22q11.21 SAT1 Spermidine/spermine N1-acetyltransferase 1 Xp22.1 Copyright © 2013 SciRes. OPEN ACCESS
 R. Cacabelos et al. / Open Journal of Psychiatry 2 (2013) 46-139 63 Continued SCNB Synuclein, beta 5q35 Lewy body dementia SCZD1 Schizophrenia disorder 1 5q11.2-q13.3 SCZD2 Schizophrenia disorder 2 11q14-q21 SCZD3 Schizophrenia disorder 3 6p24-p22 SCZD6 Schizophrenia disorder 6 8p21 SCZD7 Schizophrenia disorder 7 13q32 SCZD8 Schizophrenia disorder 8 18p SCZD10 Schizophrenia disorder 10 15q15 SCZD11 Schizophrenia susceptibility locus, chromosome 10q-related 10q22.3 SCZD12 Schizophrenia 12 1p SDCCAG8 CCCAP SLSN7 Serologically defined colon cancer antigen 8 1q43 SELENBP1 Selenium binding protein 1 1q21.3 SFRP1 Secreted frizzled-related protein 1 8p11.21 SH2B1 SH2B adaptor protein 1 16p11.2 SHANK3 SH3 and multiple ankyrin repeat domains 3 22q13.3 Chromosome 22q13.3 deletion syndrome-related autism; Chromosome 22q13.3 deletion syndrome SHMT1 Serine hydroxymethyltransferase 1 (soluble) 17p11.2 SIGMAR1 Sigma non-opioid intracellular receptor 1 9p13.3 SLC1A1 Solute carrier family 1 (neuronal/epithelial high affinity glutamate transporter, system Xag), member 1 9p24 SLC1A2 Solute carrier family 1 (glial high affinity glutamate transporter), member 2 11p13-p12 SLC1A3 Solute carrier family 1 (glial high affinity glutamate transporter), member 3 5p13 SLC1A6 Solute carrier family 1 (high affinity aspartate/glutamate transporter), member 6 19p13.12 SLC6A1 Solute carrier family 6 (neurotransmitter transporter, GABA), member 1 3p25-p24 SLC6A3 Solute carrier family 6 (neurotransmitter transporter, dopamine), member 3 5p15.3 rs6347 Anxiety disorders; Attention-deficit hyperactivity disorder; Bipolar affective disorder; Bipolar disorder; Brain diseases; Cocaine dependence; Cocaine-induced paranoia; Major depressive disorder; Parkinson’s disease; Parkinsonian disorders; Pervasive development disorders; Protection against nicotine dependence; Susceptibility to tobacco addiction; Tic disorders; Tourette’s syndrome Copyright © 2013 SciRes. OPEN ACCESS
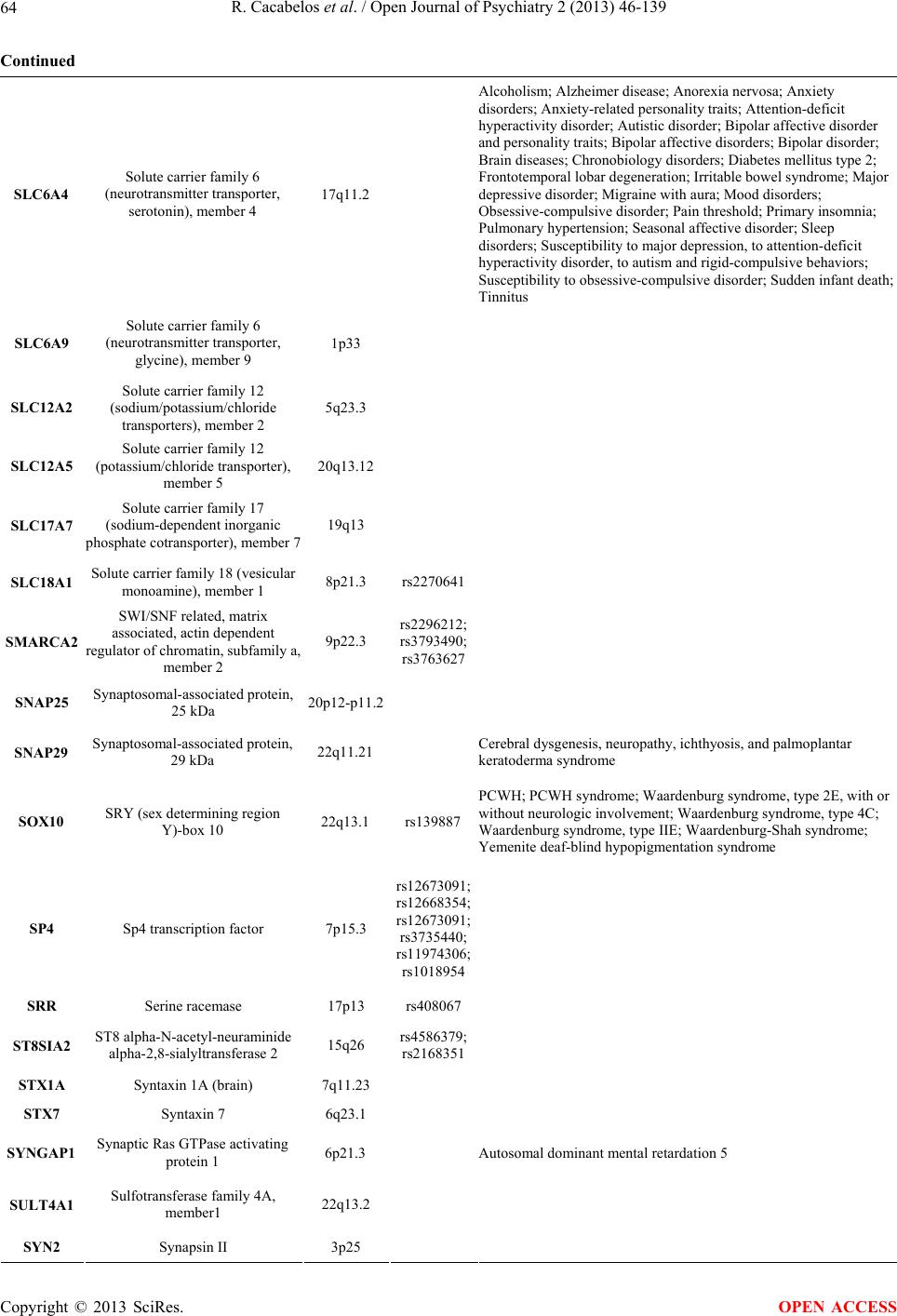 R. Cacabelos et al. / Open Journal of Psychiatry 2 (2013) 46-139 64 Continued SLC6A4 Solute carrier family 6 (neurotransmitter transporter, serotonin), member 4 17q11.2 Alcoholism; Alzheimer disease; Anorexia nervosa; Anxiety disorders; Anxiety-related personality traits; Attention-deficit hyperactivity disorder; Autistic disorder; Bipolar affective disorder and personality traits; Bipolar affective disorders; Bipolar disorder; Brain diseases; Chronobiology disorders; Diabetes mellitus type 2; Frontotemporal lobar degeneration; Irritable bowel syndrome; Major depressive disorder; Migraine with aura; Mood disorders; Obsessive-compulsive disorder; Pain threshold; Primary insomnia; Pulmonary hypertension; Seasonal affective disorder; Sleep disorders; Susceptibility to major depression, to attention-deficit hyperactivity disorder, to autism and rigid-compulsive behaviors; Susceptibility to obsessive-compulsive disorder; Sudden infant death; Tinnitus SLC6A9 Solute carrier family 6 (neurotransmitter transporter, glycine), member 9 1p33 SLC12A2 Solute carrier family 12 (sodium/potassium/chloride transporters), member 2 5q23.3 SLC12A5 Solute carrier family 12 (potassium/chloride transporter), member 5 20q13.12 SLC17A7 Solute carrier family 17 (sodium-dependent inorganic phosphate cotransporter), member 7 19q13 SLC18A1 Solute carrier family 18 (vesicular monoamine), member 1 8p21.3 rs2270641 SMARCA2 SWI/SNF related, matrix associated, actin dependent regulator of chromatin, subfamily a, member 2 9p22.3 rs2296212; rs3793490; rs3763627 SNAP25 Synaptosomal-associated protein, 25 kDa 20p12-p11.2 SNAP29 Synaptosomal-associated protein, 29 kDa 22q11.21 Cerebral dysgenesis, neuropathy, ichthyosis, and palmoplantar keratoderma syndrome SOX10 SRY (sex determining region Y)-box 10 22q13.1 rs139887 PCWH; PCWH syndrome; Waardenburg syndrome, type 2E, with or without neurologic involvement; Waardenburg syndrome, type 4C; Waardenburg syndrome, type IIE; Waardenburg-Shah syndrome; Yemenite deaf-blind hypopigmentation syndrome SP4 Sp4 transcription factor 7p15.3 rs12673091; rs12668354; rs12673091; rs3735440; rs11974306; rs1018954 SRR Serine racemase 17p13 rs408067 ST8SIA2 ST8 alpha-N-acetyl-neuraminide alpha-2,8-sialyltransferase 2 15q26 rs4586379; rs2168351 STX1A Syntaxin 1A (brain) 7q11.23 STX7 Syntaxin 7 6q23.1 SYNGAP1 Synaptic Ras GTPase activating protein 1 6p21.3 Autosomal dominant mental retardation 5 SULT4A1 Sulfotransferase family 4A, member1 22q13.2 SYN2 Synapsin II 3p25 Copyright © 2013 SciRes. OPEN ACCESS
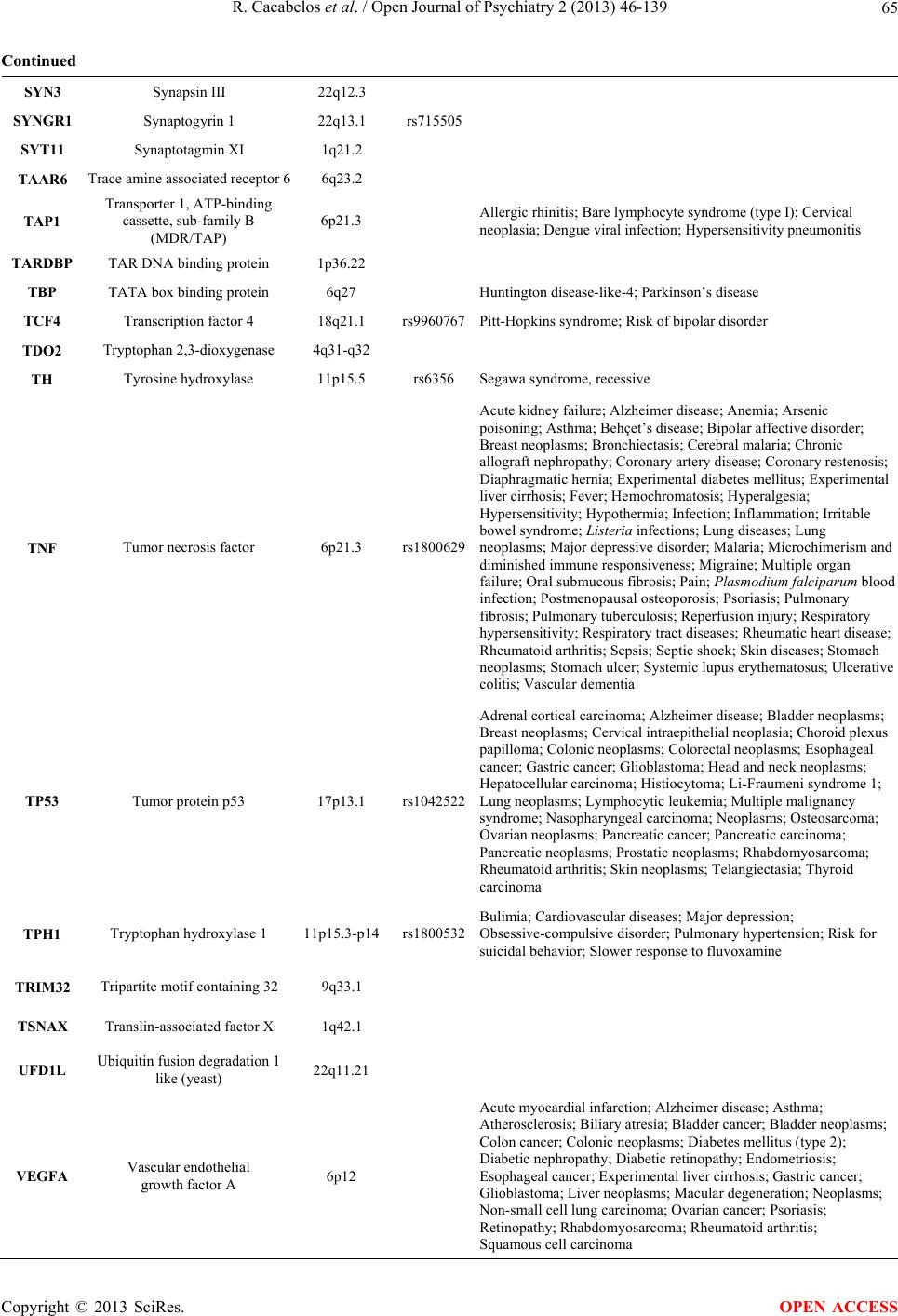 R. Cacabelos et al. / Open Journal of Psychiatry 2 (2013) 46-139 65 Continued SYN3 Synapsin III 22q12.3 SYNGR1 Synaptogyrin 1 22q13.1 rs715505 SYT11 Synaptotagmin XI 1q21.2 TAAR6 Trace amine associated receptor 6 6q23.2 TAP1 Transporter 1, ATP-binding cassette, sub-family B (MDR/TAP) 6p21.3 Allergic rhinitis; Bare lymphocyte syndrome (type I); Cervical neoplasia; Dengue viral infection; Hypersensitivity pneumonitis TARDBP TAR DNA binding protein 1p36.22 TBP TATA box binding protein 6q27 Huntington disease-like-4; Parkinson’s disease TCF4 Transcription factor 4 18q21.1 rs9960767Pitt-Hopkins syndrome; Risk of bipolar disorder TDO2 Tryptophan 2,3-dioxygenase 4q31-q32 TH Tyrosine hydroxylase 11p15.5 rs6356 Segawa syndrome, recessive TNF Tumor necrosis factor 6p21.3 rs1800629 Acute kidney failure; Alzheimer disease; Anemia; Arsenic poisoning; Asthma; Behçet’s disease; Bipolar affective disorder; Breast neoplasms; Bronchiectasis; Cerebral malaria; Chronic allograft nephropathy; Coronary artery disease; Coronary restenosis; Diaphragmatic hernia; Experimental diabetes mellitus; Experimental liver cirrhosis; Fever; Hemochromatosis; Hyperalgesia; Hypersensitivity; Hypothermia; Infection; Inflammation; Irritable bowel syndrome; Listeria infections; Lung diseases; Lung neoplasms; Major depressive disorder; Malaria; Microchimerism and diminished immune responsiveness; Migraine; Multiple organ failure; Oral submucous fibrosis; Pain; Plasmodium falciparum blood infection; Postmenopausal osteoporosis; Psoriasis; Pulmonary fibrosis; Pulmonary tuberculosis; Reperfusion injury; Respiratory hypersensitivity; Respiratory tract diseases; Rheumatic heart disease; Rheumatoid arthritis; Sepsis; Septic shock; Skin diseases; Stomach neoplasms; Stomach ulcer; Systemic lupus erythematosus; Ulcerative colitis; Vascular dementia TP53 Tumor protein p53 17p13.1 rs1042522 Adrenal cortical carcinoma; Alzheimer disease; Bladder neoplasms; Breast neoplasms; Cervical intraepithelial neoplasia; Choroid plexus papilloma; Colonic neoplasms; Colorectal neoplasms; Esophageal cancer; Gastric cancer; Glioblastoma; Head and neck neoplasms; Hepatocellular carcinoma; Histiocytoma; Li-Fraumeni syndrome 1; Lung neoplasms; Lymphocytic leukemia; Multiple malignancy syndrome; Nasopharyngeal carcinoma; Neoplasms; Osteosarcoma; Ovarian neoplasms; Pancreatic cancer; Pancreatic carcinoma; Pancreatic neoplasms; Prostatic neoplasms; Rhabdomyosarcoma; Rheumatoid arthritis; Skin neoplasms; Telangiectasia; Thyroid carcinoma TPH1 Tryptophan hydroxylase 1 11p15.3-p14rs1800532 Bulimia; Cardiovascular diseases; Major depression; Obsessive-compulsive disorder; Pulmonary hypertension; Risk for suicidal behavior; Slower response to fluvoxamine TRIM32 Tripartite motif containing 32 9q33.1 TSNAX Translin-associated factor X 1q42.1 UFD1L Ubiquitin fusion degradation 1 like (yeast) 22q11.21 VEGFA Vascular endothelial growth factor A 6p12 Acute myocardial infarction; Alzheimer disease; Asthma; Atherosclerosis; Biliary atresia; Bladder cancer; Bladder neoplasms; Colon cancer; Colonic neoplasms; Diabetes mellitus (type 2); Diabetic nephropathy; Diabetic retinopathy; Endometriosis; Esophageal cancer; Experimental liver cirrhosis; Gastric cancer; Glioblastoma; Liver neoplasms; Macular degeneration; Neoplasms; Non-small cell lung carcinoma; Ovarian cancer; Psoriasis; Retinopathy; Rhabdomyosarcoma; Rheumatoid arthritis; Squamous cell carcinoma Copyright © 2013 SciRes. OPEN ACCESS
 R. Cacabelos et al. / Open Journal of Psychiatry 2 (2013) 46-139 66 Continued VIPR2 Vasoactive intestinal peptide receptor 2 7q36.3 WNK3 WNK lysine deficient protein kinase 3 Xp11.22 XBP1 X-box binding protein 1 22q12 Susceptibility to bipolar disorder; Susceptibility to major affective disorder-7 XRCC1 X-ray repair complementing defective repair In Chinese hamster cells 4 19q13.2 rs6452536 rs35260 Acute lymphoblastic leukemia; Bladder neoplasms; Breast cancer; Breast neoplasms; Gastric cancer; Mesothelioma; Non-small cell lung cancer; Occupational diseases; Prostatic neoplasms; Squamous cell carcinoma of the head and neck XRCC4 X-ray repair complementing defective repair in Chinese hamster cells 4 5q14.2 YWHAE Tyrosine 3-monooxygenase/tryptophan 5-monooxygenase activation protein, epsilon polypeptide 17p13.3 YWHAH Tyrosine 3-monooxygenase/tryptophan 5-monooxygenase activation protein, eta polypeptide 22q12.3 ZBED4 Zinc finger, BED-type containing 4 22q13.33 ZDHHC8 Zinc finger, DHHC-type containing 8 22q11.21 ZNF804A ZInc finger protein 804A 2q32.1 rs1344706; rs7597593; rs1344706; rs4667000; rs1366842; rs3731834 (Updated from Cacabelos and Martínez-Bouza [17], and Cacabelos et al. [1]). Table 2. Pharmacogenomics of Neuroleptics. Drug Features Aripiprazole Category: Atypical antipsychotic; Arilpiperazine Mechanism: Full agonist: 5-HT1A, 5-HT1B, 5-HT1D, 5-HT6, 5-HT receptors; partial agonist: D2 and 5-HT1A receptors; antagonist: 5-HT2A receptor Genes: ABCB1, ADRA1A, CYP2D6, CYP3A4, DRD2, DRD3, HRH1, HTR1A, HTR1B, HTR1D, HTR2A, HTR2C, HTR7 Substrate: CYP2D6 (major), CYP3A4 (major) Benperidol Category: Antipsychotic, Neuroleptic; Butyrophenone Mechanism: Blocks postsynaptic mesolimbic dopaminergic D1 and D2 receptors Genes: DRD1, DRD2 Bromperidol Category: Antipsychotic, Neuroleptic; Butyrophenone Mechanism: D2 receptor antagonist; moderate serotonin 5-HT2 receptor antagonist Genes: ABCB1, ADRA1A, CYP2D6, DRD2, HTR2A Substrate: CYP2D6 (minor), CYP3A4 (major), UGTs Inhibitor: CYP2D6 (moderate) Chlorpromazine Category: Phenothiazine antipsychotic; Aliphatic phenothiazine Mechanism: Blocks postsynaptic mesolimbic dopaminergic D1 and D2 receptors; has a strong anticholinergic effect; weakly blocks ganglionic, antihistaminic and antiserotonergic receptors; blocks α-adrenergic receptors (strong); inverse agonist: 5-HT6, 5-HT7; antagonist: 5-HT1A, 5-HT2c Genes: ABCB1, ACACA, ADRA1A, ADRA2A, ADRA2B, ADRA2C, BDNF, CYP1A2, CYP2D6, CYP3A4, CYP2E1, DAO, DRD1, DRD2, DRD3, FABP1, FMO1, HRH1, HTR1A, HTR1E, HTR2A, HTR2C, HTR6, HTR7, KCNE2, LEP, NPY, SCN5A, UGT1A3, UGT1A4 Substrate: CYP1A2 (minor), CYP2D6 (major), CYP3A4 (minor), UGT1A3, UGT1A4 Inhibitor: CYP2D6 (strong), CYP2E1 (weak), DAO Copyright © 2013 SciRes. OPEN ACCESS
 R. Cacabelos et al. / Open Journal of Psychiatry 2 (2013) 46-139 67 Continued Clozapine Category: Atypical antipsychotic; Dibenzodiazepine Mechanism: Antagonist of histamine H1, cholinergic and α1-adrenergic receptors; antagonist: 5-HT1A, 5-HT2B; full agonist: 5-HT1A, 5-HT1B, 5-HT1D, 5-HT1F; inverse agonist: 5-HT6, 5-HT7 Genes: ABCB1, ADRA1A, ADRA1B, ADRA1D, ADRB3, APOA5, APOC3, APOD, CNR1, CYP1A2, CYP2A6, CYP2C8/9, CYP2C19, CYP2D6, CYP2E1, CYP3A4, DRD1, DRD2, DRD3, DRD4, DTNBP1, FABP1, GNAS1, GNB3, GSK3B, HLAA, HRH1, HRH2, HRH4, HTR1A, HTR1B, HTR1D, HTR1E, HTR1F, HTR2A, HTR2B, HTR2C, HTR3A, HTR6, HTR7, LPL, RGS2, SLC6A2, SLC6A4, TNF, UGT1A3, UGT1A4 Substrate: ABCB1, CYP1A2 (major), CYP2A6 (minor), CYP2C8/9 (minor), CYP2C19 (minor), CYP2D6 (minor), CYP3A4 (major), FMO3, UGT1A3, UGT1A4 Inhibitor: CYP1A2 (weak), CYP2C8/9 (moderate), CYP2C19 (moderate), CYP2D6 (moderate), CYP2E1 (weak), CYP3A4 (weak) Droperidol Category: Atypical antipsychotic; Butyrophenone Mechanism: Blocks dopaminergic and α-adrenergic receptors Genes: ABCC8, ADRA1A, ADRAB1, ADRA2A, DRD2, CHRM2, CYP2C9, CYP2C19, CYP3D6, CYP3A4, KCNE1, KCNE2, KCNH2, KCNJ11, KCNQ1, SCN5A Substrate: CYP2C9 (major), CYP2C19 (major), CYP2D6 (major), CYP3A4 (major) Fluphenazine Category: Typical antipsychotic; Piperazine phenothiazine Mechanism: Blocks postsynaptic mesolimbic dopaminergic D1 and D2 receptors; inverse agonist: 5-HT7; antagonist: 5-HT2A Genes: ABCB1, CYP1A2, CYP2A6, CYP2B6, CYP2C9, CYP2C19, CYP2D6, CYP2E1, CYP3A4, DRD1, DRD2, HRH1, HTR2A, HTR7 Substrate: CYP2D6 (major) Inhibitor: CYP1A2 (weak), CYP2C9 (weak), CYP2D6 (strong), CYP2E1 (weak) Flupenthixol Category: Typical antipsychotic; Thioxanthene Mechanism: Blocks postsynaptic dopaminergic receptors Genes: DRD1, DRD2 Haloperidol Category: Typical antipsychotic; Butyrophenone Mechanism: Blocks postsynaptic mesolimbic dopaminergic D1 and D2 receptors; antagonist: 5-HT2A, 5-HT2B Genes: ABCB1, ABCC1, ADRA1A, ADRA2A, BDNF, CHRM2, CYP1A2, CYP2C9, CYP2D6, CYP3A4, DRD1, DRD2, DRD4, DTNBP1, FOS, GRIN2B, GSK3B, GSTP1, HRH1, HTR2A, HTR2B, HTT, IL1RN, KCNE1, KCNE2, KCNH2, KCNJ11, KCNQ1, SCN5A, UGTs Substrate: CBR, CYP1A1 (minor), CYP1A2 (minor), CYP2C8/9 (minor), CYP2C19 (minor), CYP2D6 (major), CYP3A4 (major), UGTs Inhibitor: CYP2D6 (moderate), CYP3A4 (moderate) Loxapine Category: Typical antipsychotic; Dibenzoxazepine Mechanism: Blocks postsynaptic mesolimbic dopaminergic D1 and D2 receptors; blocks serotonin 5-HT2 receptors; inverse agonist: 5-HT2c, 5-HT6 Genes: ADRA1A, DRD1, DRD2, HRH1, HTR2A, HTR2C, HTR6, KCNE2, SCN5A, UGT1A4 Substrate: UGT1A4 Mesoridazine Category: Typical antipsychotic; Phenothiazine Mechanism: Putative dopaminergic, cholinergic, and adrenergic inhibition Genes: ADRA1A, DRD2, CHRM2, CYP2J2, KCNE1, KCNE2, KCNH2, KCNJ11, KCNQ1, SCN5A Substrate: CYP2J2 Molindone Category: Typical antipsychotic; Dihydroindolone Mechanism: Blocks postsynaptic mesolimbic dopaminergic D1 and D2 receptors; has a strong anticholinergic effect; weak ganglionic, antihistaminic and antiserotonergic block; strong α-adrenergic block; antagonist: 5-HT2A Genes: ADRA1A, CYPs, DRD2,DRD3, HRH1, HTR1A, HTR1E, HTR2A, HTR2C Olanzapine Category: Atypical antipsychotic; Thienobenzodiazepine Mechanism: Strong antagonist of serotonin 5-HT2A and 5-HT2C, 5-HT7, dopaminergic D1-4, histamine H1 and α1-adrenergic receptors; antagonist: 5-HT2A, 5-HT3 and muscarinic M1-5; full agonist: 5-HT1A, 5-HT1B, 5-HT1D, 5-HT1F; inverse agonist: 5-HT2c, 5-HT6 Genes: ABCB1, ADRA1A, ADRB3, APOA5, APOC3, CHRM1, CHRM2, CHRM3, CHRM4, CHRM5, COMT, CYP1A2, CYP2C8/9, CYP2C19, CYP2D6, CYP3A4, DRD1, DRD2, DRD3, DRD4, FMO1, GNB3, GRM3, HRH1, HRH2, HTR1A, HTR1B, HTR1D, HTR1E, HTR1F, HTR2A, HTR2C, HTR3A, HTR6, HTR7, KCNH2, LEP, LEPR, LPL, RGS2, SLC6A2, TNF, UGT1A4 Substrate: CYP1A2 (major), CYP2D6 (major), UGT1A4 Inhibitor: CYP1A2 (weak), CYP2C8/9 (weak), CYP2C19 (weak), CYP2D6 (weak), CYP3A4 (weak) Copyright © 2013 SciRes. OPEN ACCESS
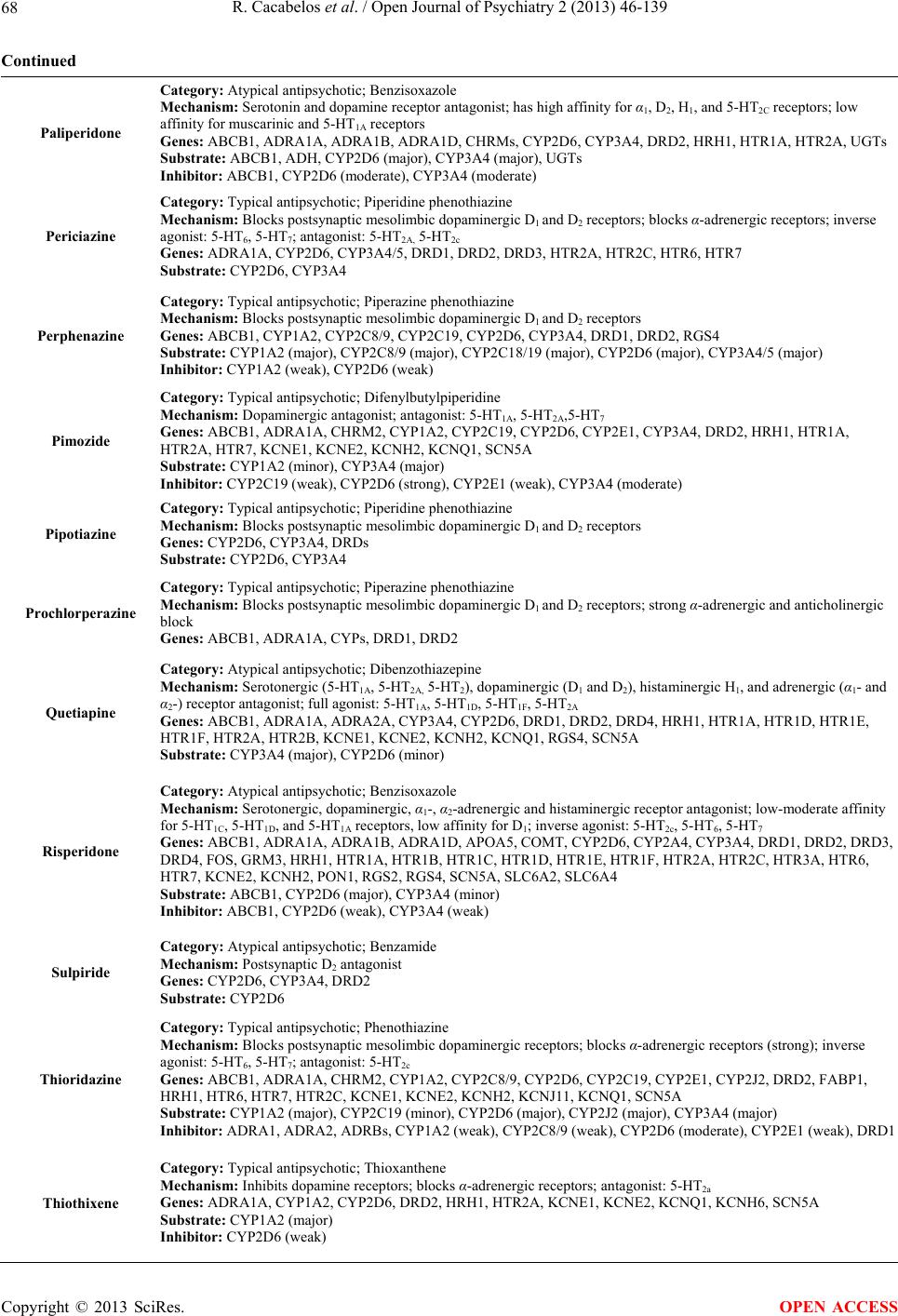 R. Cacabelos et al. / Open Journal of Psychiatry 2 (2013) 46-139 68 Continued Paliperidone Category: Atypical antipsychotic; Benzisoxazole Mechanism: Serotonin and dopamine receptor antagonist; has high affinity for α1, D2, H1, and 5-HT2C receptors; low affinity for muscarinic and 5-HT1A receptors Genes: ABCB1, ADRA1A, ADRA1B, ADRA1D, CHRMs, CYP2D6, CYP3A4, DRD2, HRH1, HTR1A, HTR2A, UGTs Substrate: ABCB1, ADH, CYP2D6 (major), CYP3A4 (major), UGTs Inhibitor: ABCB1, CYP2D6 (moderate), CYP3A4 (moderate) Periciazine Category: Typical antipsychotic; Piperidine phenothiazine Mechanism: Blocks postsynaptic mesolimbic dopaminergic D1 and D2 receptors; blocks α-adrenergic receptors; inverse agonist: 5-HT6, 5-HT7; antagonist: 5-HT2A, 5-HT2c Genes: ADRA1A, CYP2D6, CYP3A4/5, DRD1, DRD2, DRD3, HTR2A, HTR2C, HTR6, HTR7 Substrate: CYP2D6, CYP3A4 Perphenazine Category: Typical antipsychotic; Piperazine phenothiazine Mechanism: Blocks postsynaptic mesolimbic dopaminergic D1 and D2 receptors Genes: ABCB1, CYP1A2, CYP2C8/9, CYP2C19, CYP2D6, CYP3A4, DRD1, DRD2, RGS4 Substrate: CYP1A2 (major), CYP2C8/9 (major), CYP2C18/19 (major), CYP2D6 (major), CYP3A4/5 (major) Inhibitor: CYP1A2 (weak), CYP2D6 (weak) Pimozide Category: Typical antipsychotic; Difenylbutylpiperidine Mechanism: Dopaminergic antagonist; antagonist: 5-HT1A, 5-HT2A,5-HT7 Genes: ABCB1, ADRA1A, CHRM2, CYP1A2, CYP2C19, CYP2D6, CYP2E1, CYP3A4, DRD2, HRH1, HTR1A, HTR2A, HTR7, KCNE1, KCNE2, KCNH2, KCNQ1, SCN5A Substrate: CYP1A2 (minor), CYP3A4 (major) Inhibitor: CYP2C19 (weak), CYP2D6 (strong), CYP2E1 (weak), CYP3A4 (moderate) Pipotiazine Category: Typical antipsychotic; Piperidine phenothiazine Mechanism: Blocks postsynaptic mesolimbic dopaminergic D1 and D2 receptors Genes: CYP2D6, CYP3A4, DRDs Substrate: CYP2D6, CYP3A4 Prochlorperazine Category: Typical antipsychotic; Piperazine phenothiazine Mechanism: Blocks postsynaptic mesolimbic dopaminergic D1 and D2 receptors; strong α-adrenergic and anticholinergic block Genes: ABCB1, ADRA1A, CYPs, DRD1, DRD2 Quetiapine Category: Atypical antipsychotic; Dibenzothiazepine Mechanism: Serotonergic (5-HT1A, 5-HT2A, 5-HT2), dopaminergic (D1 and D2), histaminergic H1, and adrenergic (α1- and α2-) receptor antagonist; full agonist: 5-HT1A, 5-HT1D, 5-HT1F, 5-HT2A Genes: ABCB1, ADRA1A, ADRA2A, CYP3A4, CYP2D6, DRD1, DRD2, DRD4, HRH1, HTR1A, HTR1D, HTR1E, HTR1F, HTR2A, HTR2B, KCNE1, KCNE2, KCNH2, KCNQ1, RGS4, SCN5A Substrate: CYP3A4 (major), CYP2D6 (minor) Risperidone Category: Atypical antipsychotic; Benzisoxazole Mechanism: Serotonergic, dopaminergic, α1-, α2-adrenergic and histaminergic receptor antagonist; low-moderate affinity for 5-HT1C, 5-HT1D, and 5-HT1A receptors, low affinity for D1; inverse agonist: 5-HT2c, 5-HT6, 5-HT7 Genes: ABCB1, ADRA1A, ADRA1B, ADRA1D, APOA5, COMT, CYP2D6, CYP2A4, CYP3A4, DRD1, DRD2, DRD3, DRD4, FOS, GRM3, HRH1, HTR1A, HTR1B, HTR1C, HTR1D, HTR1E, HTR1F, HTR2A, HTR2C, HTR3A, HTR6, HTR7, KCNE2, KCNH2, PON1, RGS2, RGS4, SCN5A, SLC6A2, SLC6A4 Substrate: ABCB1, CYP2D6 (major), CYP3A4 (minor) Inhibitor: ABCB1, CYP2D6 (weak), CYP3A4 (weak) Sulpiride Category: Atypical antipsychotic; Benzamide Mechanism: Postsynaptic D2 antagonist Genes: CYP2D6, CYP3A4, DRD2 Substrate: CYP2D6 Thioridazine Category: Typical antipsychotic; Phenothiazine Mechanism: Blocks postsynaptic mesolimbic dopaminergic receptors; blocks α-adrenergic receptors (strong); inverse agonist: 5-HT6, 5-HT7; antagonist: 5-HT2c Genes: ABCB1, ADRA1A, CHRM2, CYP1A2, CYP2C8/9, CYP2D6, CYP2C19, CYP2E1, CYP2J2, DRD2, FABP1, HRH1, HTR6, HTR7, HTR2C, KCNE1, KCNE2, KCNH2, KCNJ11, KCNQ1, SCN5A Substrate: CYP1A2 (major), CYP2C19 (minor), CYP2D6 (major), CYP2J2 (major), CYP3A4 (major) Inhibitor: ADRA1, ADRA2, ADRBs, CYP1A2 (weak), CYP2C8/9 (weak), CYP2D6 (moderate), CYP2E1 (weak), DRD1 Thiothixene Category: Typical antipsychotic; Thioxanthene Mechanism: Inhibits dopamine receptors; blocks α-adrenergic receptors; antagonist: 5-HT2a Genes: ADRA1A, CYP1A2, CYP2D6, DRD2, HRH1, HTR2A, KCNE1, KCNE2, KCNQ1, KCNH6, SCN5A Substrate: CYP1A2 (major) Inhibitor: CYP2D6 (weak) Copyright © 2013 SciRes. OPEN ACCESS
 R. Cacabelos et al. / Open Journal of Psychiatry 2 (2013) 46-139 Copyright © 2013 SciRes. 69 OPEN ACCESS Continued Trifluoperazine Category: Typical antipsychotic; Phenothiazine Mechanism: Blocks postsynaptic mesolimbic dopaminergic receptors; blocks α-adrenergic receptors Genes: ABCB1, ADRA1A, CYP1A2, DRD2, IL12B, UGT1A4 Substrate: CYP1A2 (major), UGT1A4 Ziprasidone Category: Atypical antipsychotic; Benzylisothiazolylpiperazine Mechanism: High affinity for: D2, D3, 5-HT2A, 5-HT1A, 5-HT2C, 5-HT1D and α1-adrenergic receptors; moderate affinity for histamine H1 receptors; antagonist: D2, 5-HT1A, 5-HT2A, and 5-HT1D; full agonist: 5-HT1B, 5-HT1D; partial agonist: 5-HT1A; inverse agonist: 5-HT2c, 5-HT7 Genes: ADRA1A, AOX1, CYP1A2, CYP2D6, CYP3A4, DRD2, DRD3, DRD4, HRH1, HTR1A, HTR1B, HTR1D, HTR1E, HTR2A, HTR2C, HTR7, KCNE2, KCNH2, RGS4, SCN5A Substrate: AOXs, CYP1A2 (minor), CYP3A4 (major), HTR1A Inhibitor: CYP2D6 (moderate), CYP3A4 (moderate), HTR2A, DRD2 Zuclopenthixol Category: Typical antipsychotic; Thioxanthene Mechanism: Blocks postsynaptic mesolimbic dopaminergic receptors Genes: CYP2D6, DRD1, DRD2 Substrate: CYP2D6 (major) Symbols: ABCB1: ATP-binding cassette, sub-family B (MDR/TAP), member 1, ACACA: Acetyl-Coenzyme A carboxylase alpha, ADRA1A: Adrenergic, al- pha-1A-, receptor, ADRA1B: Adrenergic, alpha-1B-, receptor, ADRB3: Adrenergic, beta-3-, receptor, ADRA1D: Adrenergic, alpha-1D-, receptor, AOX1: Al- dehyde oxidase 1, APOA5: Apolipoprotein A-V, APOC3: Apolipoprotein C-III, APOD: Apolipoprotein D, BDNF: Brain-derived neurotrophic factor, CFTR: Cystic fibrosis transmembrane conductance regulator (ATP-binding cassette sub-family C, member 7), CHRMs: Muscarinic receptors, CHRM1: Cholinergic receptor, muscarinic 1, CHRM2: Cholinergic receptor, muscarinic 2, CHRM3: Cholinergic receptor, muscarinic 3, CHRM4: Cholinergic receptor, muscarinic 4, CHRM5: Cholinergic receptor, muscarinic 5, CNR1: Cannabinoid receptor 1 (brain), COMT: Catechol-O-methyltransferase, CYP1A2: Cytochrome P450, family 1, subfamily A, polypeptide 2, CYP2A6: Cytochrome P450, family 2, subfamily A, polypeptide 6, CYP2C19: Cytochrome P450, family 2, subfamily C, polypeptide 19, CYP2C8: Cytochrome P450, family 2, subfamily C, polypeptide 8, CYP2C9: Cytochrome P450, family 2, subfamily C, polypeptide 9, CYP2D6: Cytochrome P450, family 2, subfamily D, polypeptide 6, CYP2J2: Cytochrome P450, family 2, subfamily J, polypeptide 2, CYP2E1 : Cytochrome P450, family 2, subfamily E, polypeptide 1, CYP3A4: Cytochrome P450, family 3, subfamily A, polypeptide 4, DRDs: Dopamine receptors, DRD1: Dopamine receptor D1, DRD2: Dopamine receptor D2, DRD3: Dopamine receptor D3, DRD4: Dopamine receptor D4, DTNBP1: Dystrobrevin binding protein 1, FABP1: Fatty acid binding protein 1, liver, FMO1: Flavin containing monooxygenase 1, FOS: FBJ murine osteosarcoma viral oncogene homolog, GNAS: GNAS complex locus, GNB3: Guanine nucleotide binding protein (G protein), beta polypeptide 3, GRIN2B: Glutamate receptor, ionotropic, N-methyl D-aspartate 2B, GRM3: Glutamate receptor, metabotropic 3, GSK3B: Glycogen synthase kinase 3 beta, HLA: Major histocompatibility complex, HLA-A: Major histocompatibility complex, class I, A, HRH1: Histamine receptor H1, HRH2: Histamine receptor H2, HRH3: Histamine receptor H3, HRH4: Hista- mine receptor H4, HTR1A: 5-Hydroxytryptamine (serotonin) receptor 1A, HTR1B: 5-Hydroxytryptamine (serotonin) receptor 1B, HTR1D: 5-Hydroxytryptamine (serotonin) receptor 1D, HTR1E: 5-Hydroxytryptamine (serotonin) receptor 1E, HTR1F: 5-Hydroxytryptamine (serotonin) receptor 1F, HTR2A: 5-Hydroxytryptamine (serotonin) receptor 2A, HTR2B: 5-Hydroxytryptamine (serotonin) receptor 2B, HTR2C: 5-Hydroxytryptamine (serotonin) receptor 2C, HTR3A: 5-Hydroxytryptamine (serotonin) receptor 3A, HTR6: 5-Hydroxytryptamine (serotonin) receptor 6, HTR7:5-Hydroxytryptamine (sero- tonin) receptor 7 (adenylate cyclase-coupled), HTT: Huntingtin, IL12B: Interleukin 12B (natural killer cell stimulatory factor 2, cytotoxic lymphocyte matura- tion factor 2, p40), IL1RN: Interleukin 1 receptor antagonist, KCNE1: Potassium voltage-gated channel, Isk-related family, member 1, KCNE2: Potassium voltage-gated channel, Isk-related family, member 2, KCNH: Potassium voltage-gated channel, subfamily H (eag-related), member 1-8, KCNH2: Potassium voltage-gated channel, subfamily H (eag-related), member 2, KCNH6: Potassium voltage-gated channel, subfamily H (eag-related), member 6, KCNJ11: Potassium inwardly-rectifying channel, subfamily J, member 11, KCNQ1: Potassium voltage-gated channel, KQT-like subfamily, member 1, LEP: Leptin, LEPR: Leptin receptor, LPL: Lipoprotein lipase, NPY: Neuropeptide Y, PO N1: Paraoxonase 1, RGS2: Regulator of G-protein signaling 2, 24kDa, RGS4: Regulator of G-protein signaling 4, SCN5A: Sodium channel, voltage-gated, type V, alpha subunit, SLC6A2: Solute carrier family 6 (neurotransmitter trans- porter, noradrenalin), member 2, SLC6A4: Solute carrier family 6 (neurotransmitter transporter, serotonin), member 4, TNF: Tumor necrosis factor (TNF superfamily, member 2), UGT1A3: UDP glucuronosyltransferase 1 family, polypeptide A3, UGT1A4: UDP glucuronosyltransferase 1 family, polypeptide A4. (Generated with data from Cacabelos [25]). of CYP2D6, and 23% of CYP3A4; 24% of antidepres- sants are major substrates of CYP1A2 enzymes, 5% of CYP2B6, 38% of CYP2C19, 85% of CYP2D6, and 38% of CYP3A4; 7% of benzodiazepines are major substrates of CYP2C19 enzymes, 20% of CYP2D6, and 95% of CYP3A4 [14]. Most CYP enzymes exhibit on- togenic-, age-, sex-, circadian-, and ethnic-related dif- ferences [26]. The practical consequence of this genetic variation is that the same drug can be differentially me- tabolized according to the genetic profile/expression during each subject’s lifespan, and that knowing the pharmacogenomic profile of an individual, his/her phar- macodynamic response is potentially predictable to some extent. Among genes of the CYP superfamily with relevance in the metabolism of psychotropic drugs, the CYP2D6, CYP2C19, CYP2C9, and CYP3A4/5 genes deserve spe- cial attention. CYP2D6. CYP2D6 is a 4.38 kb gene with 9 exons mapped on 22q13.2. Four RNA transcripts of 1190 - 1684 bp are expressed in the brain, liver, spleen and re- productive system, where 4 major proteins of 48 - 55 kDa (439 - 494 aa) are identified. This protein is a trans- port enzyme of the cytochrome P450 subfamily IID or multigenic cytochrome P450 superfamily of mixed- function monooxygenases which localizes to the endo- plasmic reticulum and is known to metabolize as many as 25% of commonly-prescribed drugs and over 60% of current psychotropics. The gene is highly polymorphic in the population. There are 141 CYP2D6 allelic variants, of which −100C > T, −1023C > T, −1659G > A, −1707delT, −1846G > A, −2549delA, −2613 − 2615delAGA, −2850C > T, −2988G > A, and −3183G > A represent the 10 most important variants. Different
 R. Cacabelos et al. / Open Journal of Psychiatry 2 (2013) 46-139 70 alleles result in the extensive, intermediate, poor, and ultra-rapid metabolizer phenotypes, characterized by normal, intermediate, decreased, and multiplied ability to metabolize the enzyme’s substrates, respectively. P450 enzymes convert xenobiotics into electrophilic interme- diates which are then conjugated by phase II enzymes to hydrophilic derivatives that can be excreted. According to the database of the World Guide for Drug Use and Pharmacogenomics [25], 982 drugs are CYP2D6-related: 371 drugs are substrates, over 300 drugs are inhibitors, and 18 drugs are CYP2D6 inducers. Among healthy individuals, extensive metabolizers (EMs) account for 55.71% of the population, whereas intermediate metabolizers (IMs) are 34.7%, poor me- tabolizers (PMs) 2.28%, and ultra-rapid metabolizers (UMs) 7.31%. Remarkable interethnic differences exist in the frequency of the PM and UM phenotypes among different societies all over the world [4,27-29]. On aver- age, approximately 6.28% of the world population be- longs to the PM category. Europeans (7.86%), Polyne- sians (7.27%), and Africans (6.73%) exhibit the highest rate of PMs, whereas Orientals (0.94%) show the lowest rate [27]. The frequency of PMs among Middle Eastern populations, Asians, and Americans is in the range of 2% - 3%. CYP2D6 gene duplications are relatively infre- quent among Northern Europeans, but in East Africa the frequency of alleles with duplication of CYP2D6 is as high as 29% [30]. In Europe, there is a North-South gra- dient in the frequency of PMs (6% - 12% of PMs in Southern European countries, and 2% - 3% PMs in Northern latitudes) [25]. In SCZ, EMs, IMs, PMs, and UMs are 58.42%, 27.72%, 3.96%, and 9.9%, respectively, with a 3% increase in the frequency of EMs, and a 7% decrease in the frequency of IMs with respect to controls in the Iberian population. In Alzheimer disease (AD), EMs, IMs, PMs, and UMs are 56.38%, 27.66%, 7.45%, and 8.51%, respectively, and in vascular dementia, 52.81%, 34.83%, 6.74%, and 5.62%, respectively (Fi- gures 2 and 3). There is an accumulation of AD-related genes of risk in PMs and UMs. EMs and IMs are the best responders, and PMs and UMs are the worst responders to pharmacological treatment. Patients with depression show significant differences in the genotypic and pheno- typic profiles as compared to controls and also with re- spect to patients with psychosis, Parkinson’s disease, or brain tumors. Patients with stroke show differences as compared to patients with brain tumors, and both patients with brain tumors or with cranial nerve neuropathies differ in their CYP2D6 phenotype with regard to controls. These geno-phenotypic profiles might be important in Figure 2. CYP2D6 variants in dementia and schizophrenia. C: Controls; AD: Alzheimer disease; VD: Vascular dementia; SCZ: Schizophrenia. Copyright © 2013 SciRes. OPEN ACCESS
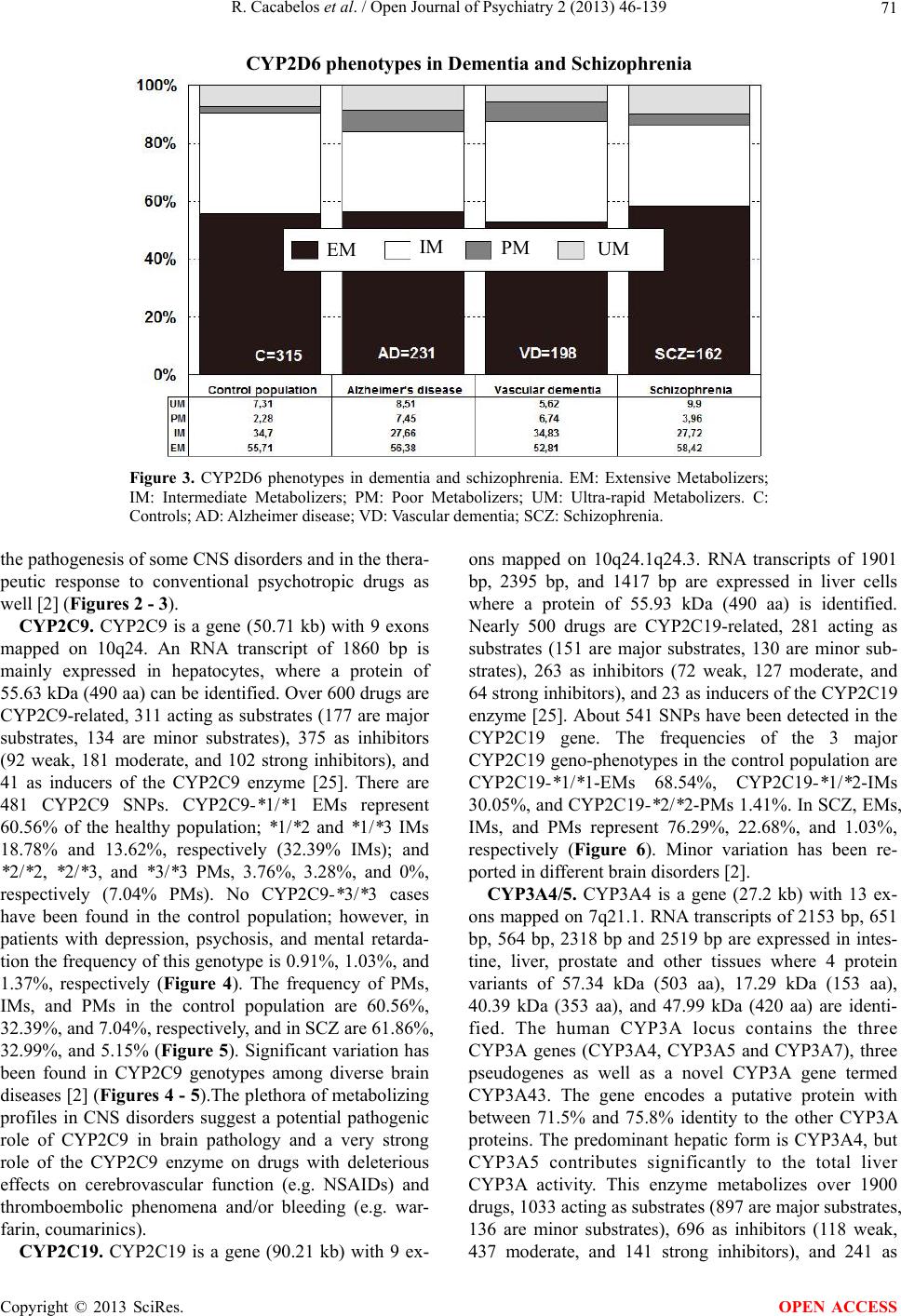 R. Cacabelos et al. / Open Journal of Psychiatry 2 (2013) 46-139 71 CYP2D6 phenotypes in Dementia and Schizophrenia EM IM PM UM Figure 3. CYP2D6 phenotypes in dementia and schizophrenia. EM: Extensive Metabolizers; IM: Intermediate Metabolizers; PM: Poor Metabolizers; UM: Ultra-rapid Metabolizers. C: Controls; AD: Alzheimer disease; VD: Vascular dementia; SCZ: Schizophrenia. the pathogenesis of some CNS disorders and in the thera- peutic response to conventional psychotropic drugs as well [2] (Figures 2 - 3). CYP2C9. CYP2C9 is a gene (50.71 kb) with 9 exons mapped on 10q24. An RNA transcript of 1860 bp is mainly expressed in hepatocytes, where a protein of 55.63 kDa (490 aa) can be identified. Over 600 drugs are CYP2C9-related, 311 acting as substrates (177 are major substrates, 134 are minor substrates), 375 as inhibitors (92 weak, 181 moderate, and 102 strong inhibitors), and 41 as inducers of the CYP2C9 enzyme [25]. There are 481 CYP2C9 SNPs. CYP2C9-*1/*1 EMs represent 60.56% of the healthy population; *1/*2 and *1/*3 IMs 18.78% and 13.62%, respectively (32.39% IMs); and *2/*2, *2/*3, and *3/*3 PMs, 3.76%, 3.28%, and 0%, respectively (7.04% PMs). No CYP2C9-*3/*3 cases have been found in the control population; however, in patients with depression, psychosis, and mental retarda- tion the frequency of this genotype is 0.91%, 1.03%, and 1.37%, respectively (Figure 4). The frequency of PMs, IMs, and PMs in the control population are 60.56%, 32.39%, and 7.04%, respectively, and in SCZ are 61.86%, 32.99%, and 5.15% (Figure 5). Significant variation has been found in CYP2C9 genotypes among diverse brain diseases [2] (Figures 4 - 5).The plethora of metabolizing profiles in CNS disorders suggest a potential pathogenic role of CYP2C9 in brain pathology and a very strong role of the CYP2C9 enzyme on drugs with deleterious effects on cerebrovascular function (e.g. NSAIDs) and thromboembolic phenomena and/or bleeding (e.g. war- farin, coumarinics). CYP2C19. CYP2C19 is a gene (90.21 kb) with 9 ex- ons mapped on 10q24.1q24.3. RNA transcripts of 1901 bp, 2395 bp, and 1417 bp are expressed in liver cells where a protein of 55.93 kDa (490 aa) is identified. Nearly 500 drugs are CYP2C19-related, 281 acting as substrates (151 are major substrates, 130 are minor sub- strates), 263 as inhibitors (72 weak, 127 moderate, and 64 strong inhibitors), and 23 as inducers of the CYP2C19 enzyme [25]. About 541 SNPs have been detected in the CYP2C19 gene. The frequencies of the 3 major CYP2C19 geno-phenotypes in the control population are CYP2C19-*1/*1-EMs 68.54%, CYP2C19-*1/*2-IMs 30.05%, and CYP2C19-*2/*2-PMs 1.41%. In SCZ, EMs, IMs, and PMs represent 76.29%, 22.68%, and 1.03%, respectively (Figure 6). Minor variation has been re- ported in different brain disorders [2]. CYP3A4/5. CYP3A4 is a gene (27.2 kb) with 13 ex- ons mapped on 7q21.1. RNA transcripts of 2153 bp, 651 bp, 564 bp, 2318 bp and 2519 bp are expressed in intes- tine, liver, prostate and other tissues where 4 protein variants of 57.34 kDa (503 aa), 17.29 kDa (153 aa), 40.39 kDa (353 aa), and 47.99 kDa (420 aa) are identi- fied. The human CYP3A locus contains the three CYP3A genes (CYP3A4, CYP3A5 and CYP3A7), three pseudogenes as well as a novel CYP3A gene termed CYP3A43. The gene encodes a putative protein with between 71.5% and 75.8% identity to the other CYP3A proteins. The predominant hepatic form is CYP3A4, but CYP3A5 contributes significantly to the total liver CYP3A activity. This enzyme metabolizes over 1900 drugs, 1033 acting as substrates (897 are major substrates, 136 are minor substrates), 696 as inhibitors (118 weak, 437 moderate, and 141 strong inhibitors), and 241 as Copyright © 2013 SciRes. OPEN ACCESS
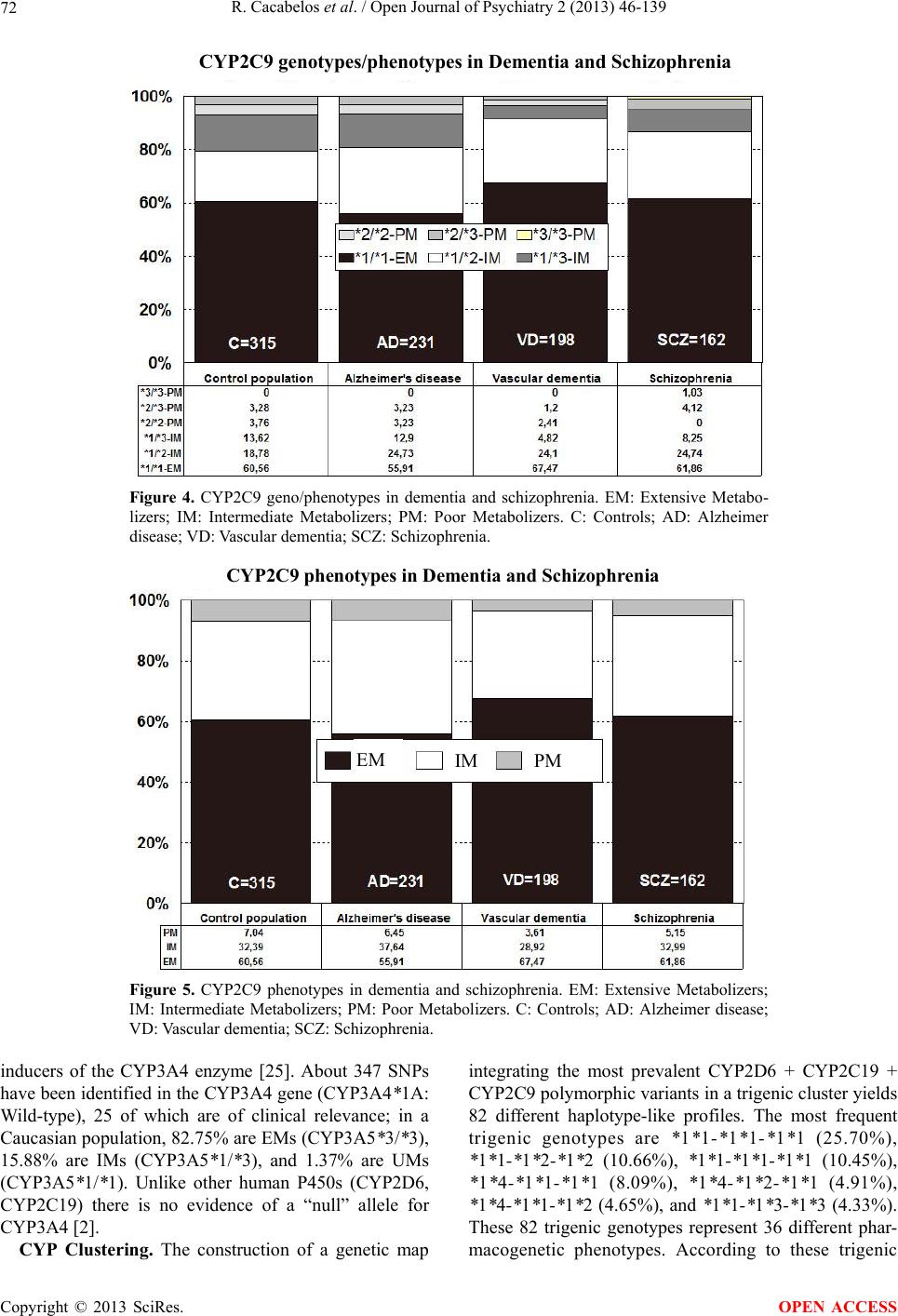 R. Cacabelos et al. / Open Journal of Psychiatry 2 (2013) 46-139 72 CYP2C9 genot ype s/ ph e notypesin Demen ti a and Schizoph reni a Figure 4. CYP2C9 geno/phenotypes in dementia and schizophrenia. EM: Extensive Metabo- lizers; IM: Intermediate Metabolizers; PM: Poor Metabolizers. C: Controls; AD: Alzheimer disease; VD: Vascular dementia; SCZ: Schizophrenia. CYP2C9 phenotypesin Dementia and Schizophrenia EM IM PM Figure 5. CYP2C9 phenotypes in dementia and schizophrenia. EM: Extensive Metabolizers; IM: Intermediate Metabolizers; PM: Poor Metabolizers. C: Controls; AD: Alzheimer disease; VD: Vascular dementia; SCZ: Schizophrenia. inducers of the CYP3A4 enzyme [25]. About 347 SNPs have been identified in the CYP3A4 gene (CYP3A4*1A: Wild-type), 25 of which are of clinical relevance; in a Caucasian population, 82.75% are EMs (CYP3A5*3/*3), 15.88% are IMs (CYP3A5*1/*3), and 1.37% are UMs (CYP3A5*1/*1). Unlike other human P450s (CYP2D6, CYP2C19) there is no evidence of a “null” allele for CYP3A4 [2]. CYP Clustering. The construction of a genetic map integrating the most prevalent CYP2D6 + CYP2C19 + CYP2C9 polymorphic variants in a trigenic cluster yields 82 different haplotype-like profiles. The most frequent trigenic genotypes are *1*1-*1*1-*1*1 (25.70%), *1*1-*1*2-*1*2 (10.66%), *1*1-*1*1-*1*1 (10.45%), *1*4-*1*1-*1*1 (8.09%), *1*4-*1*2-*1*1 (4.91%), *1*4-*1*1-*1*2 (4.65%), and *1*1-*1*3-*1*3 (4.33%). These 82 trigenic genotypes represent 36 different phar- macogenetic phenotypes. According to these trigenic Copyright © 2013 SciRes. OPEN ACCESS
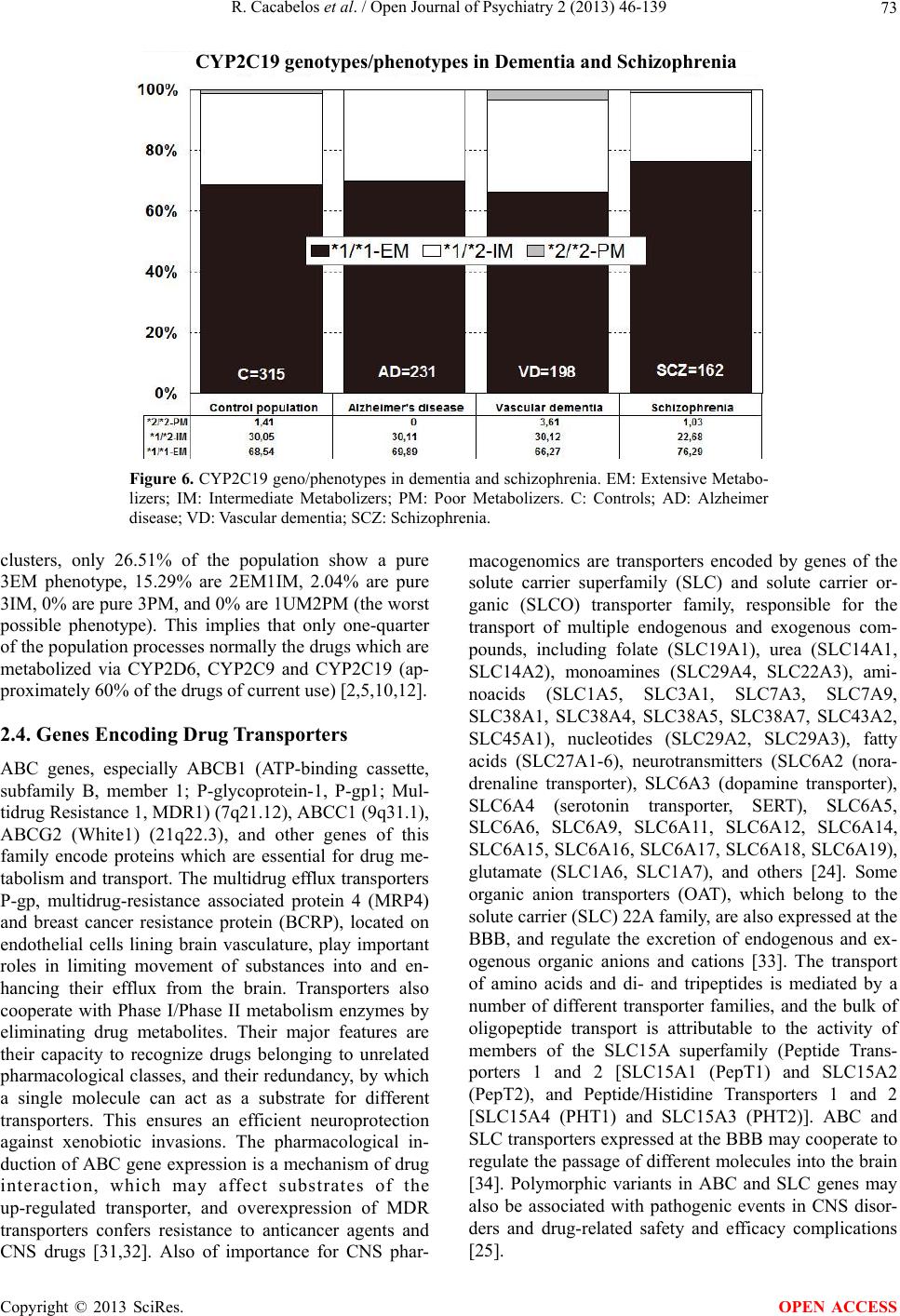 R. Cacabelos et al. / Open Journal of Psychiatry 2 (2013) 46-139 73 CYP2 C19 genotypes/ phenotyp esin Dementia a nd Schizophrenia Figure 6. CYP2C19 geno/phenotypes in dementia and schizophrenia. EM: Extensive Metabo- lizers; IM: Intermediate Metabolizers; PM: Poor Metabolizers. C: Controls; AD: Alzheimer disease; VD: Vascular dementia; SCZ: Schizophrenia. clusters, only 26.51% of the population show a pure 3EM phenotype, 15.29% are 2EM1IM, 2.04% are pure 3IM, 0% are pure 3PM, and 0% are 1UM2PM (the worst possible phenotype). This implies that only one-quarter of the population processes normally the drugs which are metabolized via CYP2D6, CYP2C9 and CYP2C19 (ap- proximately 60% of the drugs of current use) [2,5,10,12]. 2.4. Genes Encoding Drug Transporters ABC genes, especially ABCB1 (ATP-binding cassette, subfamily B, member 1; P-glycoprotein-1, P-gp1; Mul- tidrug Resistance 1, MDR1) (7q21.12), ABCC1 (9q31.1), ABCG2 (White1) (21q22.3), and other genes of this family encode proteins which are essential for drug me- tabolism and transport. The multidrug efflux transporters P-gp, multidrug-resistance associated protein 4 (MRP4) and breast cancer resistance protein (BCRP), located on endothelial cells lining brain vasculature, play important roles in limiting movement of substances into and en- hancing their efflux from the brain. Transporters also cooperate with Phase I/Phase II metabolism enzymes by eliminating drug metabolites. Their major features are their capacity to recognize drugs belonging to unrelated pharmacological classes, and their redundancy, by which a single molecule can act as a substrate for different transporters. This ensures an efficient neuroprotection against xenobiotic invasions. The pharmacological in- duction of ABC gene expression is a mechanism of drug interaction, which may affect substrates of the up-regulated transporter, and overexpression of MDR transporters confers resistance to anticancer agents and CNS drugs [31,32]. Also of importance for CNS phar- macogenomics are transporters encoded by genes of the solute carrier superfamily (SLC) and solute carrier or- ganic (SLCO) transporter family, responsible for the transport of multiple endogenous and exogenous com- pounds, including folate (SLC19A1), urea (SLC14A1, SLC14A2), monoamines (SLC29A4, SLC22A3), ami- noacids (SLC1A5, SLC3A1, SLC7A3, SLC7A9, SLC38A1, SLC38A4, SLC38A5, SLC38A7, SLC43A2, SLC45A1), nucleotides (SLC29A2, SLC29A3), fatty acids (SLC27A1-6), neurotransmitters (SLC6A2 (nora- drenaline transporter), SLC6A3 (dopamine transporter), SLC6A4 (serotonin transporter, SERT), SLC6A5, SLC6A6, SLC6A9, SLC6A11, SLC6A12, SLC6A14, SLC6A15, SLC6A16, SLC6A17, SLC6A18, SLC6A19), glutamate (SLC1A6, SLC1A7), and others [24]. Some organic anion transporters (OAT), which belong to the solute carrier (SLC) 22A family, are also expressed at the BBB, and regulate the excretion of endogenous and ex- ogenous organic anions and cations [33]. The transport of amino acids and di- and tripeptides is mediated by a number of different transporter families, and the bulk of oligopeptide transport is attributable to the activity of members of the SLC15A superfamily (Peptide Trans- porters 1 and 2 [SLC15A1 (PepT1) and SLC15A2 (PepT2), and Peptide/Histidine Transporters 1 and 2 [SLC15A4 (PHT1) and SLC15A3 (PHT2)]. ABC and SLC transporters expressed at the BBB may cooperate to regulate the passage of different molecules into the brain [34]. Polymorphic variants in ABC and SLC genes may also be associated with pathogenic events in CNS disor- ders and drug-related safety and efficacy complications [25]. Copyright © 2013 SciRes. OPEN ACCESS
 R. Cacabelos et al. / Open Journal of Psychiatry 2 (2013) 46-139 74 2.5. Pleiotropic Genes Apolipoprotein E (APOE) is a pathogenic gene in de- mentia and the prototypical paradigm of a pleiotropic gene with multifaceted activities in physiological and pathological conditions, including cardiovascular disease, dyslipidemia, atherosclerosis, stroke, and AD [2,6,14]. ApoE is consistently associated with the amyloid plaque marker for AD. APOE-4 may influence AD pathology interacting with APP metabolism and Aβ accumulation, enhancing hyperphosphorylation of tau protein and NFT formation, reducing choline acetyltransferase activity, increasing oxidative processes, modifying inflammation- related neuroimmunotrophic activity and glial activation, altering lipid metabolism, lipid transport and membrane biosynthesis in sprouting and synaptic remodeling, and inducing neuronal apoptosis [35]. The distribution of APOE genotypes in the Iberian Peninsula is as follows: APOE-2/2 0.32%, APOE-2/3 7.3%, APOE-2/4 1.27%, APOE-3/3 71.11%, APOE-3/4 18.41%, and APOE-4/4 1.59% (Figure 3). These fre- quencies are very similar in Europe and in other Western societies. There is a clear accumulation of APOE-4 car- riers among patients with AD (APOE-3/4 30.30%; APOE-4/4 6.06%) and vascular dementia (APOE-3/4 35.85%, APOE-4/4 6.57%) as compared to controls (Figure 7). The distribution and frequencies of APOE genotypes in AD also differ from those of patients with anxiety, depression, psychosis, migraine, vascular en- cephalopathy, and post-traumatic brain injury syndrome [2,6,14] (Figure 3). Different APOE genotypes confer specific phenotypic profiles to AD patients. Some of these profiles may add risk or benefit when the patients are treated with conventional drugs, and in many in- stances the clinical phenotype demands the administra- tion of additional drugs which increase the complexity of therapeutic protocols. From studies designed to define APOE-related AD phenotypes, several conclusions can be drawn: 1) the age-at-onset is 5 - 10 years earlier in approximately 80% of AD cases harboring the APOE-4/4 genotype; 2) the serum levels of ApoE are lowest in APOE-4/4, intermediate in APOE-3/3 and APOE-3/4, and highest in APOE-2/3 and APOE-2/4; 3) serum cho- lesterol levels are higher in APOE-4/4 than in the other genotypes; 4) HDL-cholesterol levels tend to be lower in APOE-3 homozygotes than in APOE-4 allele carriers; 5) LDL-cholesterol levels are systematically higher in APOE-4/4 than in any other genotype; 6) triglyceride levels are significantly lower in APOE-4/4; 7) nitric ox- ide levels are slightly lower in APOE-4/4; 8) serum and cerebrospinal fluid Aβ levels tend to differ between APOE-4/4 and the other most frequent genotypes (APOE-3/3, APOE-3/4); 9) blood histamine levels are dramatically reduced in APOE-4/4 as compared with the other genotypes; 10) brain atrophy is markedly increased in APOE-4/4 > APOE-3/4 > APOE-3/3; 11) brain map- ping activity shows a significant increase in slow wave activity in APOE-4/4 from early stages of the disease; 12) brain hemodynamics, as reflected by reduced brain blood flow velocity and increased pulsatility and resistance indices, is significantly worse in APOE-4/4 (and in APOE-4 carriers in general, as compared with APOE-3 carriers); brain hypoperfusion and neocortical oxygena- tion is also more deficient in APOE-4 carriers; 13) lym- phocyte apoptosis is markedly enhanced in APOE-4 car- riers; 14) cognitive deterioration is faster in APOE-4/4 Figure 7. APOE genotypes in dementia and schizophrenia. Copyright © 2013 SciRes. OPEN ACCESS
 R. Cacabelos et al. / Open Journal of Psychiatry 2 (2013) 46-139 75 patients than in carriers of any other APOE genotype; 15) in approximately 3% - 8% of the AD cases, the presence of some dementia-related metabolic dysfunctions accu- mulates more in APOE-4 carriers than in APOE-3 carri- ers; 16) some behavioral disturbances, alterations in cir- cadian rhythm patterns, and mood disorders are slightly more frequent in APOE-4 carriers; 17) aortic and sys- temic atherosclerosis is also more frequent in APOE-4 carriers; 18) liver metabolism and transaminase activity also differ in APOE-4/4 with respect to other genotypes; 19) hypertension and other cardiovascular risk factors also accumulate in APOE-4; and 20) APOE-4/4 carriers are the poorest responders to conventional drugs. These 20 major phenotypic features clearly illustrate the bio- logical disadvantage of APOE-4 homozygotes and the potential consequences that these patients may experi- ence when they receive pharmacological treatment for AD and/or concomitant pathologies [2,4-6,9-12,35]. When APOE and CYP2D6 genotypes are integrated in bigenic clusters and the APOE + CYP2D6-related thera- peutic response to a combination therapy is analyzed in AD patients, it becomes clear that the presence of the APOE-4/4 genotype is able to convert pure CYP2D6*1/*1 extensive metabolizers into full poor responders to con- ventional treatments, indicating the existence of a pow- erful influence of the APOE-4 homozygous genotype on the drug-metabolizing capacity of pure CYP2D6 exten- sive metabolizers. In addition, a clear accumulation of APOE-4/4 genotypes is observed among CYP2D6 poor and ultra-rapid metabolizers [4,11]. 3. GENOMICS OF SCHIZOPHRENIA AND PSYCHOTIC DISORDERS 3.1. Structural Genomics Genetic studies in SCZ have revealed the presence of cytogenetic changes, chromosome anomalies and multi- ple candidate genes potentially associated with psychosis and related traits; and neurocognitive impairment was found to be heritable in individuals with SCZ and their relatives [36]. First-degree relatives of probands with SCZ or bipolar disorder (BD) are at increased risk of these disorders. Half-siblings have a significantly in- creased risk, but substantially lower than that of full- siblings. Heritability for SCZ and BD is 64% and 59%, respectively. Shared environmental effects are small but substantial (SCZ: 4.5%, 4.4% - 7.4%; BD: 3.4%, 2.3% - 6.2%) for both disorders. SCZ and BD partly share common genetic determinants [37]. Linkage analysis of SCZ in African-American families revealed that several regions show a decrease in the evidence for linkage as the definition broadens: 8q22.1 (rs911, 99.26 cM), 16q24.3 (rs1006547, 130.48 cM), and 20q13.2 (rs1022689, 81.73 cM). One region shows a substantial increase in evidence for linkage, 11p15.2 (rs722317, 24.27 cM). These linkage results overlap two broad, previously- reported linkage regions: 8p23.3-p12, found in studies sampling largely families of European ancestry; and 11p11.2-q22.3, reported by a study of African-American families [38]. The genome-wide linkage scan analysis of 707 Euro- pean-ancestry families identified suggestive evidence for linkage on chromosomes 8p21, 8q24.1, 9q34 and 12q24.1. Genome-wide significant evidence for linkage was ob- served on chromosome 10p12. Significant heterogeneity was also observed on chromosome 22q11.1. Evidence for linkage across family sets and analyses was most consistent on chromosome 8p21, with a one-LOD sup- port interval that does not include the candidate gene NRG1, suggesting that one or more other susceptibility loci might exist in the region [39]. Genome-wide sig- nificant evidence for linkage for SCZ or schizoaffective disorder was found in a region on chromosome 17q21 in families of Mexican and Central American ancestry. A region on chromosome 15q22-23 showed suggestive evidence of linkage with this same phenotype [40]. The human genome is enriched in interspersed seg- mental duplications that sensitize approximately 10% of our genome to recurrent microdeletions and microdupli- cations as a result of unequal crossing-over. Studies of common complex genetic disease show that a subset of these recurrent events plays an important role in autism, SCZ, and epilepsy [41]. The advent of genome-wide SNP and copy number variant (CNV) microarray tech- nologies heralds identification of additional SCZ loci. Over 200 genes, reported in about 2400 studies, have been associated with SCZ during the past two decades; however, it is likely that over 1000 genes might be in- volved in SCZ pathogenesis through epistatic interaction and epigenetic phenomena. Many of these associations could not be replicated in different populations, as hap- pens with many other multifactorial/complex disorders [42]. Data suggest that these susceptibility genes influ- ence the cortical information processing which charac- terizes the schizophrenic phenotype. Aberrant postnatal brain maturation is an essential mechanism underlying the disease. Several candidate genes have been suggested, with the strongest evidence for genes encoding dystro- brevin binding protein 1 (DTNBP1), neuregulin 1 (NRG1), neuregulin 1 receptor (ERBB4) and disrupted in SCZ1 (DISC1), as well as several neurotrophic factors. These genes are involved in neuronal plasticity and also play a role in adult neurogenesis [43]. Several studies of the dystrobrevin-binding protein 1 gene (DTNBP1), neuregulin 1 (NRG1), D-amino-acid oxidase (DAO), DAO activator (DAOA, G72), and me- tabotropic glutamate receptor 3 (GRM3) genes have suggested an association between variants of these genes Copyright © 2013 SciRes. OPEN ACCESS
 R. Cacabelos et al. / Open Journal of Psychiatry 2 (2013) 46-139 76 and SCZ; however, several studies did not replicate as- sociations of DTNBP1, NRG1, DAO, DAOA, and GRM3 gene polymorphisms and SCZ [44]. Within the last 2 years, a number of genome-wide association stud- ies (GWAS) of SCZ and BD have been published [45-48]. These have produced stronger evidence for association to specific risk loci than had earlier studies, specifically for the zinc finger binding protein 804A (ZNF804A) locus in SCZ and for the calcium channel, voltage-dependent, L type, alpha 1C subunit (CACNA1C) and ankyrin 3, node of Ranvier (ANK3) loci in BD. The ZNF804A and CACNA1C loci appear to influence risk for both disor- ders, a finding that supports the hypothesis that SCZ and BD are not etiologically distinct. In the case of SCZ, a number of rare copy number variants have also been de- tected that have fairly large effect sizes on disease risk, and that additionally influence risk of autism, mental retardation, and other neurodevelopmental disorders. The existing findings point to some likely pathophysiological mechanisms but also challenge current concepts of dis- ease classification [49]. A genome scan meta-analysis (GSMA) was carried out on 32 independent genome- wide linkage scan analyses that included 3255 pedigrees with 7413 genotyped cases affected with SCZ or related disorders. Suggestive evidence for linkage was observed in two single bins, on chromosomes 5q (142 - 168 Mb) and 2q (103 - 134 Mb). Genome-wide evidence for link- age was detected on chromosome 2q (119 - 152 Mb) when bin boundaries were shifted to the middle of the previous bins. The primary analysis met empirical crite- ria for “aggregate” genome-wide significance, indicating that some or all of 10 bins are likely to contain loci linked to SCZ, including regions of chromosomes 1, 2q, 3q, 4q, 5q, 8p and 10q. In a secondary analysis of 22 studies of European-ancestry samples, suggestive evi- dence for linkage was observed on chromosome 8p (16 - 33 Mb) [50]. In another GWAS, evidence was found for association to genes reported in other GWAS data sets (CACNA1C) or to closely-related family members of those genes, including CSF2RB, CACNA1B and DGKI [51]. A summary of genes (or pathological pathways) with potential effect in SCZ pathogenesis is the major goal of the present review in order to understand the complex molecular mechanisms which might be responsible for the clinical manifestations of one of the oldest diseases in the constellation of mental illness. 3.2. Genes Potentially Associated with Schizophrenia and Psychotic Disorders ABCA13 (ATP-binding cassette, sub-family A (ABC1), member 13). The lipid transporter gene ABCA13 is a susceptibility factor for both SCZ and BD. SCZ and BD are leading causes of morbidity across all populations, with heritability estimates of approximately 80%, indi- cating a substantial genetic component. Population ge- netics and GWAS suggest an overlap of genetic risk fac- tors between these illnesses. Knight et al. [52] rese- quenced ABCA13 exons in cases with SCZ and controls. Multiple rare coding variants were identified, including one nonsense and nine missense mutations and com- pound heterozygosity/homozygosity in 6% of cases. Variants were genotyped in additional SCZ, bipolar, de- pression and control cohorts, and the frequency of all rare variants combined was greater than controls in SCZ and BD. The population-attributable risk of these muta- tions was 2.2% for SCZ and 4.0% for BD [52]. Abelson helper integration site 1 (AHI1). The Abel- son helper integration site 1 (AHI1) gene locus on chro- mosome 6q23.3 is among a group of candidate loci for SCZ susceptibility that were initially identified by link- age followed by linkage disequilibrium (LD) mapping, and subsequent replication of the association in an inde- pendent sample. The region contains two genes, AHI1 and C6orf217. Both genes and the neighboring phos- phodiesterase 7B (PDE7B) may be considered candi- dates for involvement in the genetic etiology of SCZ [53]. Of 14 SNPs tested (ATP2B2, HS3ST2, UNC5C, BAG3, PDE7B, PAICS, PTGFRN, NR3C2, ZBTB20, ST6GAL2, PIP5K1B, EPHA6, KCNH5, and AJAP1), only one (rs9389370) in PDE7B (high-affinity cAMP-specific pho- sphodiesterase 7B) showed significant evidence for as- sociation with SCZ in a Japanese sample [54]. The AHI1 gene is required for both cerebellar and cor- tical development in humans. According to Rivero et al. [55], while the accelerated evolution of AHI1 in the hu- man lineage indicates a role in cognitive function, a linkage scan in large pedigrees identified AHI1 as a posi- tional candidate for SCZ. These authors evaluated the effect of AHI1 variation on the vulnerability to psychosis in two samples from Spain and Germany. 29 SNPs lo- cated in a genomic region including the AHI1 gene were genotyped in the Ibero-German sample. rs7750586 and rs911507, both located upstream of the AHI1 coding region, were found to be associated with SCZ in the analysis of genotypic and allelic frequencies. Several other risk and protective haplotypes were also detected. Joint analysis of both ethnic samples supported the asso- ciation of rs7750586 and rs911507 with the risk for SCZ. Adenylosuccinate synthase (ADSS) and ataxia te- langiectasia (ATM) genes. The blood-derived RNA lev- els of the adenylosuccinate synthase (ADSS) and ataxia telangiectasia mutated (ATM) genes were found to be down- and up-regulated, respectively, in schizophrenics compared with controls, and ADSS and ATM were among eight biomarker genes to discriminate schizo- phrenics from normal controls. ADSS catalyzes the first committed step of AMP synthesis, while ATM kinase Copyright © 2013 SciRes. OPEN ACCESS
 R. Cacabelos et al. / Open Journal of Psychiatry 2 (2013) 46-139 77 serves as a key signal transducer in the DNA dou- ble-strand breaks response pathway. Studies with 6 SNPs in the ADSS gene and 3 SNPs in the ATM gene did not show significant difference in the genotype, allele, or haplotype distributions in a Chinese population of SCZ patients. Using the Multifactor Dimensionality Reduc- tion (MDR) method, interactions among rs3102460 in the ADSS gene and rs227061 and rs664143 in the ATM gene revealed a significant association with SCZ with a maximum testing accuracy of 60.4%, suggesting that the combined effects of the polymorphisms in the ADSS and ATM genes may confer susceptibility to the development of SCZ in a Chinese population [56]. Adrenergic alpha-2A, receptor (ADRA2A). Several lines of studies have shown the existence of an important inhibitory mechanism for the control of water intake in- volving adrenergic alpha-2A receptors. A human study using patients with SCZ demonstrated an exacerbation of polydipsia by the administration of clonidine, an ADR- A2A-agonist, and a relief of polydipsia by mianserin, an ADRA2A-antagonist, suggesting the involvement of the central adrenergic system in the drinking behavior of patients with SCZ. Based on these findings Yamaguchi et al. [57] examined a possible association between the C-1291G polymorphism in the promoter region of the ADRA2A gene and polydipsia in SCZ using a Japanese case-control sample. No significant association between the ADRA2A C-1291G polymorphism and polydipsia was found [57]. ALDHs and retinoic acid-related genes. Vitamin A (retinol), the biologically active form of retinoic acid, has been proposed as being involved in the pathogenesis of SCZ. 18 SNPs in the regulatory and coding sequences of 7 genes involved in the synthesis, degradation and transportation of retinoic acid, ALDH1A1, ALDH1A2, ALDH1A3, CYP26A1, CYP26B1, CYP26C1 and trans- thyretin (TTR) have been studied in SCZ. Association analyses using both allelic and genotypic single-locus tests revealed no significant association between the risk for each of these genes and SCZ; however, analyses of multiple-locus haplotypes indicated that the overall fre- quency of rs4646642-rs4646580 of the ALDH1A2 gene showed a significant difference between patients and control subjects in the Chinese population [58]. Alpha- and beta-synuclein (SNCA, SNCB). Al- pha-synuclein is expressed in the CNS. A high concen- tration of alpha-synuclein in presynaptic terminals can mimic the normal function of endogenous alpha-synu- clein in regulating synaptic vesicle mobilization at nerve terminals. Beta-synuclein protein is seen primarily in brain tissue. Beta-synuclein may act as an inhibitor of alpha-synuclein aggregation, which occurs in neurode- generative diseases, such as Parkinson’s disease. Noori- Daloii et al. [59] studied the changes of alpha- and beta-synucleins in SCZ patients in relation to a control group. The relative expression of alpha- and beta-synu- cleins showed downregulation in patients in comparison to the control group. Beta-synuclein mRNA expression in the control group was significantly higher than that in the patient group, but downregulation of alpha-synuclein gene was not significant. Angiotensin I converting enzyme (peptidyl-dipep- tidase A) 1 (ACE). ACE variants are currently associ- ated with cardiovascular and cerebrovascular risk factors [5,10,11,35]. ACE insertion/deletion polymorphism was also associated with SCZ and BD. DD genotype and D allele distributions in bipolar patients and their first-de- gree relatives were significantly higher than those of SCZ patients, their relatives, and controls. In contrast, II genotype and I allele were reduced in both the patient groups and their relatives as compared with controls [60]. AP-3 complex genes. Dysbindin is a component of BLOC-1, which interacts with the adaptor protein (AP)-3 complex. Hashimoto et al. [61] examined a possible as- sociation between 16 SNPs in the AP3 complex genes and SCZ in Japanese patients. Nominal association be- tween rs6688 in the AP3M1 gene and SCZ was initially found, but this association was no longer positive after correction for multiple testing, suggesting that AP3 com- plex genes might not play a major role in the pathogene- sis of SCZ in this population [61]. APOE and cholesterol transport genes. Several studies suggest an accumulation of APOE-4 allele in SCZ [35]. Disturbances in lipid homeostasis and myeli- nation have been proposed in the pathophysiology of SCZ and BD. Several antipsychotic and antidepressant drugs increase lipid biosynthesis through activation of the Sterol Regulatory Element-Binding Protein (SREBP) transcription factors, which control the expression of numerous genes involved in fatty acid and cholesterol biosynthesis. Quantitative PCR and immunoblotting were used to determine the level of lipid transport genes in human glioblastoma (GaMg) exposed to clozapine, olanzapine, haloperidol or imipramine. The effect of some of these drugs was also investigated in human as- trocytoma (CCF-STTG1), neuroblastoma (SH-SY5Y) and hepatocellular carcinoma (HepG2) cells. Significant transcriptional changes of cholesterol transport genes (APOE, ABCA1, NPC1, NPC2, NPC1L1), which are predominantly controlled by the liver X receptor (LXR; NR1H2) transcription factor, have been detected. Stimu- lation of cellular lipid biosynthesis by amphiphilic psy- chotropic drugs is followed by a transcriptional activa- tion of cholesterol transport and efflux pathways. Such effects may be relevant for both therapeutic effects and metabolic adverse effects of psychotropic drugs [62]. Copyright © 2013 SciRes. OPEN ACCESS
 R. Cacabelos et al. / Open Journal of Psychiatry 2 (2013) 46-139 78 Apoptotic engulfment pathway. Apoptosis has been speculated to be involved in SCZ. The apoptotic engulf- ment pathway involving the MEGF10, GULP1, ABCA1 and ABCA7 genes has been investigated in SCZ. Nomi- nally significant associations were found in GULP1 (rs2004888), ABCA1 (rs3858075) and ABCA7 genes. A significant 2-marker (rs2242436*rs3858075) interaction between the ABCA1 and ABCA7 genes and a 3-marker interaction (rs246896*rs4522565*rs3858075) amongst the MEGF10, GULP1 and ABCA1 genes were found in different samples. The GULP1 gene and the apoptotic engulfment pathway may be involved in SCZ in subjects of European ancestry and multiple genes in the pathway may interactively increase the risk of the disease [63]. Arginine vasopressin receptor 1A (AVPR1A). Ar- ginine vasopressin (AVP) and the arginine vasopressin receptor 1A gene contribute to memory function and a range of social behaviors both in lower vertebrates and in humans. Human promoter-region microsatellite repeat regions (RS1 and RS3) in the AVPR1a gene region have been associated with autism spectrum disorders, proso- cial behavior and social cognition. Prepulse inhibition (PPI) of the startle response to auditory stimuli is a largely autonomic response that resonates with social cognition in both animal models and humans. Reduced PPI has been observed in disorders, including SCZ, which are distinguished by deficits in social skills. Asso- ciation studies between PPI and the AVPR1a RS1 and RS repeat regions detected association between AVPR1a promoter-region repeat length (especially RS3) and PPI. Longer RS3 alleles were associated with greater levels of prepulse inhibition. Using a short/long classification scheme for the repeat regions, significant association was also observed between all three PPI intervals (30, 60 and 120 ms) and both RS1 and RS3 polymorphisms. Longer alleles, especially in male subjects, are associated with significantly higher PPI response, consistent with a role for the promoter repeat region in partially molding social behavior in both animals and humans [64]. Molecular genetic studies of AVPR1a and oxytocin (OXTR) recep- tors have strengthened the evidence regarding the role of these two neuropeptides in a range of normal and patho- logical behaviors. Significant association has been shown between both AVPR1a repeat regions and OXTR SNPs with risk for autism. AVPR1a has also been linked to eating behavior in both clinical and non-clinical groups. Evidence also suggests that repeat variations in AVPR1a are associated with two other social domains in Homo sapiens: music and altruism. AVPR1a was associated with dance and musical cognition, probably reflecting the ancient role of this hormone in social interactions executed by vocalization, ritual movement and dyadic (mother-offspring) and group communication. Individual differences have been observed in allocation of funds in the dictator game, a laboratory game of pure altruism, associated with length of the AVPR1a RS3 promoter- region repeat [65]. Although molecular data are very limited, it might be possible that dysfunctions in these primitive neuropeptides involved in higher activities of the CNS may influence autism/SCZ pathogenesis [66]. Arrestin, beta 2 (ARRB2). Tardive dyskinesia (TD) may be associated with mediators or signaling complexes behind DRD2, such as beta-arrestin-2 (ARRB2), an im- portant mediator between DRD2 and serine-threonine protein kinase (AKT) signal cascade. There is a signifi- cant difference in the genotype distribution of ARRB2- rs1045280 (Ser280Ser) between TD and non-TD SCZ groups; and patients with the T allele have increased risk of tardive dyskinesia [67]. BCL2-interacting killer (apoptosis-inducing) (BIK). The Bcl2-interacting killer (BIK) gene interacts with cellular and viral survival-promoting proteins, such as Bcl-2, to enhance apoptosis. The BIK protein promotes cell death in a manner analogous to Bcl-2-related death- promoting proteins, Bax and Bak. Low Bcl-2 levels and increased Bax/Bcl-2 ratio have been found in the tempo- ral cortex of patients with SCZ. The BIK protein is sug- gested to be a likely target for antiapoptotic proteins. Nominal evidence for association of alleles rs926328 and rs2235316 with SCZ was found in Japanese patients; however, these associations were no longer positive after correction for multiple testing [68]. Biogenesis of lysosome-related organelles complex 1 (BLOC-1). Biogenesis of lysosome-related organelles complex 1 (BLOC-1) is a protein complex formed by the products of eight distinct genes. Loss-of-function muta- tions in two of these genes, DTNBP1 and BLOC1S3, cause Hermansky-Pudlak syndrome, a human disorder characterized by defective biogenesis of lysosome-re- lated organelles. Haplotype variants within the same two genes have been postulated to increase the risk of devel- oping SCZ. In a fly model of BLOC-1 deficiency, mutant flies lacking the conserved Blos1 subunit displayed eye pigmentation defects due to abnormal pigment granules, which are lysosome-related organelles, as well as ab- normal glutamatergic transmission and behavior [69]. Brain-derived neurotrophic factor (BDNF). A vari- ety of evidence suggests brain-derived neurotrophic fac- tor (BDNF) as a candidate gene for SCZ. Several genetic studies have shown a significant association between the disease and certain SNPs within BDNF (specifically, Val66Met and C270T). The functional microsatellite marker BDNF-LCPR (BDNF-linked complex polymor- phic region), which affects the expression level of BDNF, is associated with BD. A meta-analysis of the two most extensively studied polymorphisms (Val66Met and C270T) revealed no association in single-marker or mul- timarker analysis and no association of the Val66Met Copyright © 2013 SciRes. OPEN ACCESS
 R. Cacabelos et al. / Open Journal of Psychiatry 2 (2013) 46-139 79 polymorphism with SCZ, whereas C270T showed a trend for association in a fixed model, but not in a random model. These findings suggest that if BDNF is indeed associated with SCZ, the A1 allele in BDNF-LCPR would be the most promising candidate [70]. In a Tai- wanese population no association was found between the BDNF Val66Met polymorphism and SCZ; however, this polymorphism may reduce psychopathology, in particu- lar negative symptoms [71]. Allelic variation in the BDNF gene has been associated with affective disorders, but generally not SCZ. BDNF variants may help clarify the status of schizoaffective disorder. Patients with schizoaffective disorder and other affective disorders are significantly more likely to carry two copies of the most common BDNF haplotype (containing the valine allele of the Val66Met polymorphism) compared with healthy volunteers. When compared with SCZ patients, individu- als with schizoaffective disorder are significantly more likely to carry two copies of the common haplotype [72]. Defective BDNF has been proposed as a candidate pathogenic mechanism in SCZ and dementia. BDNF transcription is regulated during the protracted period of human frontal cortex development. Expression of the four most abundant alternative 5’ exons of the BDNF gene (exons I, II, IV, and VI) has been studied in RNA extracted from the prefrontal cortex. Expression of tran- scripts I-IX and VI-IX was highest during infancy, whereas that of transcript II-IX was lowest just after birth, slowly increasing to reach a peak in toddlers. Transcript IV-IX was significantly upregulated within the first year of life, and was maintained at this level until school age. Quantification of BDNF protein revealed that levels fol- lowed a similar developmental pattern as transcript IV-IX. In situ hybridization of mRNA in cortical sections showed the highest expression in layers V and VI for all four BDNF transcripts, whereas moderate expression was observed in layers II and III. Although low expres- sion of BDNF was observed in cortical layer IV, this BDNF mRNA low-zone decreased in prominence with age and showed an increase in neuronal mRNA localiza- tion. These findings reported by Wong et al. [73] show that dynamic regulation of BDNF expression occurs through differential use of alternative promoters during the development of the human prefrontal cortex, particu- larly in the younger age groups, when the prefrontal cor- tex is more plastic. Alterations in BDNF processing dur- ing brain maturation cannot be neglected as a potential mechanism for prefrontal cortex dysfunction in SCZ. The levels of (pro)BDNF and receptor proteins, TrkB and p75, are altered in the hippocampus in SCZ and mood disor- der and polymorphisms in each gene influence protein expression [74]. Neurodegenerative processes may be involved in the pathogenesis of tardive dyskinesia (TD), and a growing body of evidence suggests that BDNF plays a role in both the antipsychotic effects and the pathogenesis of TD. BDNF and glycogen synthase kinase (GSK)-3beta are important in neuronal survival, and thus abnormal regu- lation of BDNF and GSK-3beta may contribute to TD pathophysiology. Park et al. [75] studied the relationship between two polymorphisms, Val66Met in the BDNF coding region and −50T/C in the GSK-3beta promoter, and susceptibility to TD among a matched sample of patients having SCZ with TD, patients with SCZ without TD, and normal control subjects. PCR analysis revealed no significant difference in the occurrence of the poly- morphisms among the TD, non-TD, and control subjects, but a significant interaction was observed among the groups possessing BDNF Val allele in compound geno- types. The schizophrenic subjects with the C/C GSK-3beta genotype, who carry the Val allele of the BDNF gene, are expected to have a decreased risk of developing neuro- leptic-induced tardive dyskinesia [75]. Bromodomain containing 1 (BRD1). The bromodo- main-containing protein 1 (BRD1) gene located at chro- mosome 22q13.33 has been associated with SCZ and BD susceptibility [76]. Calcium channel, voltage-dependent, L type, alpha 1C subunit (CACNA1C). Strong evidence of associa- tion at the polymorphism rs1006737 (within CACNA1C, the gene encoding the alpha-1C subunit of the L-type voltage-gated calcium channel) with the risk of BD has recently been reported in a meta-analysis of three ge- nome-wide association studies of BD. The risk allele also conferred increased risk for SCZ and recurrent major depression with similar effect sizes to those previously observed in BD. These findings are evidence of some degree of overlap in the biological underpinnings of sus- ceptibility to mental illness across the clinical spectrum of mood and psychotic disorders [77]. A recent meta- analysis of five case-control cohorts for major mood dis- order, including over 13600 individuals genotyped on high-density SNP arrays performed by the Bipolar Dis- order Genome Study (BiGS) Consortium [78] identified SNPs at 3p21.1 associated with major mood disorders (rs2251219), with supportive evidence for association observed in two out of three independent replication co- horts. These results provide another example of a shared genetic susceptibility locus for BD and major depressive disorder [78]. To identify the neural system mechanism that explains the genetic association between the CACNA1C gene and psychiatric illness, Bigos et al. [79] used blood oxygena- tion level-dependent (BOLD) functional magnetic reso- nance imaging (fMRI) to measure brain activation in circuitries related to bipolar disorder and schizophrenia by comparing CACNA1C genotype groups among healthy subjects. The risk-associated SNP rs1006737 in Copyright © 2013 SciRes. OPEN ACCESS
 R. Cacabelos et al. / Open Journal of Psychiatry 2 (2013) 46-139 80 CACNA1C predicted increased hippocampal activity during emotional processing and increased prefrontal activity during executive cognition. The risk-associated SNP also predicted increased expression of CACNA1C mRNA in the human brain. The risk-associated SNP in CACNA1C maps to circuitries implicated in genetic risk for bipolar disorder and schizophrenia. According to Bigos and coworkers [79], its effects in human brain expression implicate a molecular and neural system mechanism for the clinical genetic association. Calreticulin (CALR). Tissue-specific expression of the calreticulin (CALR) gene in the gray matter is de- velopment-dependent and coincides with the expression of psychosis phenotypes. Farokhashtiani et al. [80] re- ported instances of mutations within the core promoter sequence of the gene in schizoaffective disorder. A unique mutation at nucleotide −220 from the transcrip- tion start site, located at a conserved genomic block in the promoter region of the gene, co-occurs with the spec- trum of psychoses. This mutation reverts the human promoter sequence to the ancestral type observed in chimpanzee, mouse, and several other species, implying that the genomic block harboring nucleotide −220 may be involved in the evolution of human-specific higher- order functions of the brain that are ubiquitously im- paired in psychoses. Cannabinoid receptors. Two endocannabinoid re- ceptors, CB1 and CB2 (CNR1, CNR2), are found in the brain. The R63 allele of rs2501432 (R63Q), the C allele of rs12744386 and the haplotype of the R63-C allele of CB2 were significantly increased among patients with SCZ. A significantly lower response to CB2 ligands in cultured CHO cells transfected with the R63 allele com- pared with those with Q63, and significantly lower CB2 receptor mRNA and protein levels found in human brain with the CC and CT genotypes of rs12744386 compared with TT genotype were observed. Endocannabinoid function appears to be involved in SCZ. An increased risk of SCZ might be present in people with low CB2 receptor function [81]. Cathepsin K (CTSK). Recent studies associate cathepsin K with SCZ. Cathepsin K is capable of liber- ating Met-enkephalin from beta-endorphin (beta-EP) in vitro. To verify if this process might possibly contribute to the pathogenesis of SCZ, post-mortem brains were analyzed immunohistochemically for the presence and co-localization of cathepsin K and beta-EP. In support of a functional role of the observed formation of Met-en- kephalin on the expense of beta-EP, increased numbers of cathepsin K immunoreactive cells, but diminished numbers of both beta-EP-positive cells and double-posi- tive (cathepsin K/beta-EP) cells, were found in left and right arcuate nucleus of schizophrenics. A reduced den- sity of beta-EP-immunoreactive neuropil (fibers, nerve terminals) was estimated in the left and right paraven- tricular nucleus (PVN) of individuals with SCZ. Cathep- sin K, which becomes up-regulated in its cerebral ex- pression by neuroleptic treatment, might significantly contribute to altered opioid levels in brains of schizo- phrenics [82]. Cholecystokinin A receptor gene. Cholecystokinin A receptor (CCKAR) has been implicated in the patho- physiology of SCZ through its mediation of dopamine- release in the CNS. Association between the CCKAR gene and SCZ has been observed, especially between the 779T/C polymorphism and auditory hallucinations or positive symptoms of SCZ. In the Japanese population, no significant difference was observed in genotypic dis- tributions or allelic frequencies between SCZ and con- trols, although there was a trend for the association be- tween the C allele of the polymorphism and hallucination or hallucinatory-paranoid state [83]. Cholinergic receptor, nicotinic, alpha 7 (CHRNA7). Multiple genetic linkage studies support the hypothesis that the 15q14 chromosomal region contributes to the etiology of SCZ. Among the putative candidate genes in this area are the alpha7 nicotinic acetylcholine receptor gene (CHRNA7) and its partial duplication, CHRFAM7A. A large chromosomal segment including the CHRFAM7A gene locus, but not the CHRNA7 locus, is deleted in some individuals. The CHRFAM7A gene contains a polymorphism consisting of a 2 base pair (2 bp) deletion at position 497 - 498 bp of exon 6. The 2 bp polymor- phism was associated with SCZ in African-Americans and in Caucasians [84]. The rs3087454 SNP, located at position −1831 bp in the upstream regulatory region of CHRNA7, was significantly associated with SCZ in Af- rican-American and non-Hispanic Caucasian case-con- trol samples [85]. CLOCK. The clock genes have been reported to play some roles in neural transmitter systems, including the dopamine system, as well as to regulate circadian rhythms. Abnormalities in both of these mechanisms are thought to be involved in the pathophysiology of major mental illness such as SCZ and mood disorders including BP and major depressive disorder (MDD). Recent ge- netic studies have reported that CLOCK, one of the clock genes, might be associated with these psychiatric disor- ders; however, association of CLOCK with SCZ might be weak [86]. Clusterin (CLU). Clusterin (CLU) and clathrin as- sembly lymphoid myeloid (CALM; PICALM) protein are implicated in the function of neuronal synapses. Zhou et al. [87] examined whether SNPs rs11136000 within the CLU gene and rs3851179 within the CALM gene, were associated with SCZ in the Chinese population. Patients with SCZ and with family history showed a sig- nificant increase of allele C frequency in rs11136000 in Copyright © 2013 SciRes. OPEN ACCESS
 R. Cacabelos et al. / Open Journal of Psychiatry 2 (2013) 46-139 81 comparison to normal controls. The C allele frequency was also higher in patients with negative symptoms. In contrast, allele and genotype frequencies of rs3851179 did not show significant differences between patients and normal subjects or between patients with different sym- ptoms. CMYA5 (Cardiomyopathy-associated protein 5; myosprym; tripartite motif-containing protein 76, TRIM76). Chen et al. [88] found that in the CMYA5 gene, there were two non-synonymous markers, rs3828611 and rs10043986, showing nominal signifi- cance in both the CATIE (Clinical Antipsychotic Trials of Intervention Effectiveness) and MGS-GAIN (molecu- lar genetics of schizophrenia genome-wide association study supported by the genetic association information network) samples. In a combined analysis of both the CATIE and MGS-GAIN samples, rs4704591 was identi- fied as the most significant marker in the gene. Linkage disequilibrium analyses indicated that these markers were in low LD. CMYA5 was reported to be physically interacting with the DTNBP1 gene, a promising candi- date for schizophrenia, suggesting that CMYA5 may be involved in the same biological pathway and process. In a meta-analysis of all 23 replication samples (family samples, 912 families with 4160 subjects; case-control samples, 11380 cases and 15021 controls), the authors found that the rs10043986 and rs4704591 markers are significantly associated with SCZ. Haplotype condi- tioned analyses indicated that the association signals ob- served at these two markers are independent. CNP. 2’,3’-Cyclic nucleotide 3’-phosphodiesterase (CNP), another candidate gene for SCZ, participates in oligodendrocyte function and in myelination. Five CNP SNPs were investigated in a Chinese Han SCZ case- control sample set with negative results. Factors includ- ing gender, genotype, sub-diagnosis and antipsychotic treatment were found not to contribute to the expression regulation of the CNP gene in SCZ [89]. CNTNAP2, NRXN1, and the neurexin superfamily. Heterozygous copy-number variants and SNPs of CNTNAP2 and NRXN1, two distantly related members of the neurexin superfamily, have been repeatedly asso- ciated with a wide spectrum of neuropsychiatric disor- ders, such as developmental language disorders, autism spectrum disorders, epilepsy, and SCZ. Homozygous and compound-heterozygous deletions and mutations via molecular karyotyping and mutational screening in CNTNAP2 and NRXN1 were identified in four patients with severe mental retardation and variable features, such as autistic behavior, epilepsy, and breathing anoma- lies, phenotypically overlapping with Pitt-Hopkins syn- drome. With a frequency of at least 1%, recessive defects in CNTNAP2 appear to contribute significantly to severe mental retardation. As known for fly Nrx-1, the CASPR2 ortholog Nrx-IV might also localize to synapses. Over- expression of either protein can reorganize synaptic morphology and induce increased density of active zones, the synaptic domains of neurotransmitter release. Both Nrx-I and Nrx-IV determine the level of the presynaptic active-zone protein bruchpilot, indicating a possible common molecular mechanism in Nrx-1 and Nrx-IV mutant conditions [90]. Complexin-2. Because synaptic dysfunction plays a key role in SCZ, the complexin 2 gene (CPLX2) was examined by Begemann et al. [91] in the first phenotype- based genetic association study (PGAS) of GRAS (Göt- tingen Research Association for Schizophrenia). Six SNPs, distributed over the whole CPLX2 gene, were found to be highly associated with current cognition of schizophrenic subjects but only marginally with premor- bid intelligence. Correspondingly, in CPLX2-null mutant mice, prominent cognitive loss of function was obtained only in combination with a minor brain lesion applied during puberty. In the human CPLX2 gene, 1 of the iden- tified 6 cognition-relevant SNPs, rs3822674 in the 3’ untranslated region, was detected to influence mi- croRNA-498 binding and gene expression. The same marker was associated with differential expression of CPLX2 in peripheral blood mononuclear cells. Results extracted from this study suggest that cognitive perfor- mance in schizophrenic patients may be modified by CPLX2 variants modulating post-transcriptional gene expression. COMT. The catechol-O-methyltransferase (COMT) gene, which is located in the 22q11.21 microdeletion, has been considered as a candidate gene for SCZ due to its ability to degrade catecholamines, including dopamine. Human COMT contains three common polymorphisms (A22S, A52T, and V108M), two of which (A22S and V108M) render the protein susceptible to deactivation by temperature or oxidation. The A52T mutation had no significant effect on COMT structure. Residues 22 (al- pha2) and 108 (alpha5) interact with each other and are located in a polymorphic hotspot approximately 20 Ǻ from the active site. Introduction of either the larger Ser (22) or Met (108) tightens this interaction, pulling alpha2 and alpha5 toward each other and away from the protein core. The V108M polymorphism rearranges active-site residues in alpha5, beta3, and alpha6, increasing the S-adenosylmethionine site solvent exposure. The A22S mutation reorients alpha2, moving critical catechol- binding residues away from the substrate-binding pocket. The A22S and V108M polymorphisms evolved inde- pendently in Northern European and Asian populations. While the decreased activities of both A22S and V108M COMT are associated with an increased risk for SCZ, the V108M-induced destabilization is also linked with im- proved cognitive function. Polymorphisms within this Copyright © 2013 SciRes. OPEN ACCESS
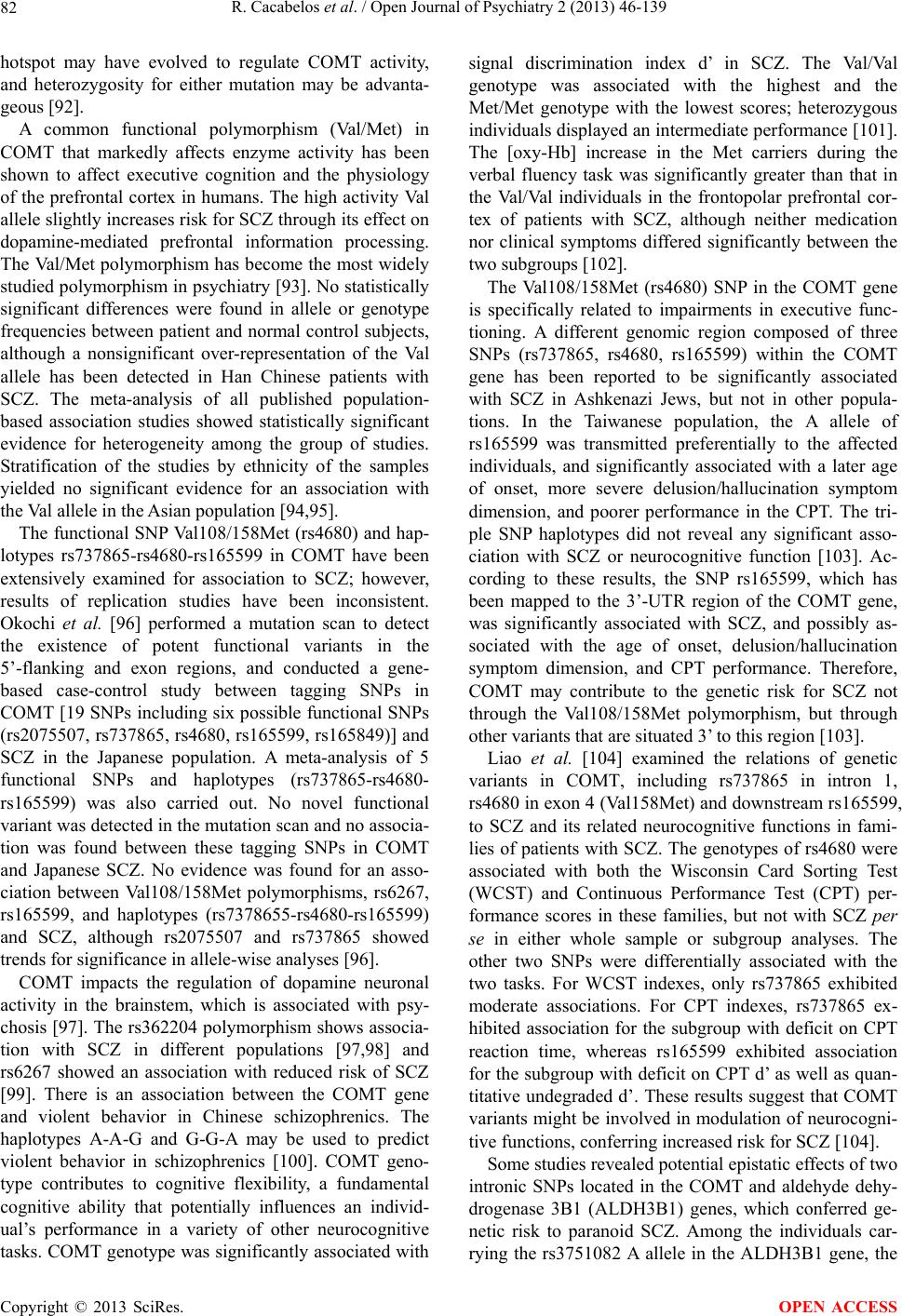 R. Cacabelos et al. / Open Journal of Psychiatry 2 (2013) 46-139 82 hotspot may have evolved to regulate COMT activity, and heterozygosity for either mutation may be advanta- geous [92]. A common functional polymorphism (Val/Met) in COMT that markedly affects enzyme activity has been shown to affect executive cognition and the physiology of the prefrontal cortex in humans. The high activity Val allele slightly increases risk for SCZ through its effect on dopamine-mediated prefrontal information processing. The Val/Met polymorphism has become the most widely studied polymorphism in psychiatry [93]. No statistically significant differences were found in allele or genotype frequencies between patient and normal control subjects, although a nonsignificant over-representation of the Val allele has been detected in Han Chinese patients with SCZ. The meta-analysis of all published population- based association studies showed statistically significant evidence for heterogeneity among the group of studies. Stratification of the studies by ethnicity of the samples yielded no significant evidence for an association with the Val allele in the Asian population [94,95]. The functional SNP Val108/158Met (rs4680) and hap- lotypes rs737865-rs4680-rs165599 in COMT have been extensively examined for association to SCZ; however, results of replication studies have been inconsistent. Okochi et al. [96] performed a mutation scan to detect the existence of potent functional variants in the 5’-flanking and exon regions, and conducted a gene- based case-control study between tagging SNPs in COMT [19 SNPs including six possible functional SNPs (rs2075507, rs737865, rs4680, rs165599, rs165849)] and SCZ in the Japanese population. A meta-analysis of 5 functional SNPs and haplotypes (rs737865-rs4680- rs165599) was also carried out. No novel functional variant was detected in the mutation scan and no associa- tion was found between these tagging SNPs in COMT and Japanese SCZ. No evidence was found for an asso- ciation between Val108/158Met polymorphisms, rs6267, rs165599, and haplotypes (rs7378655-rs4680-rs165599) and SCZ, although rs2075507 and rs737865 showed trends for significance in allele-wise analyses [96]. COMT impacts the regulation of dopamine neuronal activity in the brainstem, which is associated with psy- chosis [97]. The rs362204 polymorphism shows associa- tion with SCZ in different populations [97,98] and rs6267 showed an association with reduced risk of SCZ [99]. There is an association between the COMT gene and violent behavior in Chinese schizophrenics. The haplotypes A-A-G and G-G-A may be used to predict violent behavior in schizophrenics [100]. COMT geno- type contributes to cognitive flexibility, a fundamental cognitive ability that potentially influences an individ- ual’s performance in a variety of other neurocognitive tasks. COMT genotype was significantly associated with signal discrimination index d’ in SCZ. The Val/Val genotype was associated with the highest and the Met/Met genotype with the lowest scores; heterozygous individuals displayed an intermediate performance [101]. The [oxy-Hb] increase in the Met carriers during the verbal fluency task was significantly greater than that in the Val/Val individuals in the frontopolar prefrontal cor- tex of patients with SCZ, although neither medication nor clinical symptoms differed significantly between the two subgroups [102]. The Val108/158Met (rs4680) SNP in the COMT gene is specifically related to impairments in executive func- tioning. A different genomic region composed of three SNPs (rs737865, rs4680, rs165599) within the COMT gene has been reported to be significantly associated with SCZ in Ashkenazi Jews, but not in other popula- tions. In the Taiwanese population, the A allele of rs165599 was transmitted preferentially to the affected individuals, and significantly associated with a later age of onset, more severe delusion/hallucination symptom dimension, and poorer performance in the CPT. The tri- ple SNP haplotypes did not reveal any significant asso- ciation with SCZ or neurocognitive function [103]. Ac- cording to these results, the SNP rs165599, which has been mapped to the 3’-UTR region of the COMT gene, was significantly associated with SCZ, and possibly as- sociated with the age of onset, delusion/hallucination symptom dimension, and CPT performance. Therefore, COMT may contribute to the genetic risk for SCZ not through the Val108/158Met polymorphism, but through other variants that are situated 3’ to this region [103]. Liao et al. [104] examined the relations of genetic variants in COMT, including rs737865 in intron 1, rs4680 in exon 4 (Val158Met) and downstream rs165599, to SCZ and its related neurocognitive functions in fami- lies of patients with SCZ. The genotypes of rs4680 were associated with both the Wisconsin Card Sorting Test (WCST) and Continuous Performance Test (CPT) per- formance scores in these families, but not with SCZ per se in either whole sample or subgroup analyses. The other two SNPs were differentially associated with the two tasks. For WCST indexes, only rs737865 exhibited moderate associations. For CPT indexes, rs737865 ex- hibited association for the subgroup with deficit on CPT reaction time, whereas rs165599 exhibited association for the subgroup with deficit on CPT d’ as well as quan- titative undegraded d’. These results suggest that COMT variants might be involved in modulation of neurocogni- tive functions, conferring increased risk for SCZ [104]. Some studies revealed potential epistatic effects of two intronic SNPs located in the COMT and aldehyde dehy- drogenase 3B1 (ALDH3B1) genes, which conferred ge- netic risk to paranoid SCZ. Among the individuals car- rying the rs3751082 A allele in the ALDH3B1 gene, the Copyright © 2013 SciRes. OPEN ACCESS
 R. Cacabelos et al. / Open Journal of Psychiatry 2 (2013) 46-139 83 rs4633 T allele in the COMT gene was associated with susceptibility to paranoid SCZ, development of halluci- nation, delay of P300 latency, and increased expression of the COMT gene; however, the rs4633 T allele did not show any association in the rs3751082 G/G genotype carriers [105]. CSF2RB (Colony stimulating factor 2 receptor, beta, low-affinity (granulocyte-macrophage)). CSF2RB en- codes the protein which is the common β chain of the high affinity receptor for IL-3, IL-5 and CSF. It locates in the linkage region 22q13.1 of both bipolar disorder and SCZ, and is expressed in most cells. Chen et al. [106] carried out a large-scale case-control study to test the association between CSF2RB and three major mental disorders in the Chinese Han population. Seven SNPs were genotyped in 1140 bipolar affective disorder pa- tients (including 645 type I bipolar affective disorder patients), 1140 schizophrenia patients, 1139 major de- pressive disorder patients and 1140 healthy controls. Three SNPs were found to be associated with both SCZ and major depressive disorder. Haplotype association analysis revealed one protective haplotype for SCZ and for MDD and one risk haplotype for SCZ and for MDD. These results support CSF2RB as a risk factor common to both SCZ and major depression in the Chinese Han population. CTLA4 (Cytotoxic T-lymphocyte-associated pro- tein 4). Some studies have reported that the cytotoxic T lymphocyte antigen-4 (CTLA-4) gene, which is related to immunological functions such as T-cell regulation, is associated with psychiatric disorders. Liu et al. [107] studied the relationship between CTLA-4 and three ma- jor psychiatric disorders, SCZ, major depressive disorder and bipolar disorder in the Chinese Han population. They screened 6 tag SNPs (rs231777, rs231775, rs231779, rs3087243, rs5742909, rs16840252) in the CTLA-4 gene, and found that rs231779 conferred a risk for SCZ, major depressive disorder, and bipolar disorder. rs231777 and rs16840252 had a significant association with SCZ, and rs231777 had significant association with bipolar disor- der; however, after 10000 permutations, only rs231779 remained significant. These results led the Chinese au- thors to conclude that shared common risk factors for SCZ, major depressive disorder and bipolar disorder ex- ist in the CTLA-4 gene in the Chinese Han population. CYP3A4 and CYP3A5. Two-marker haplotypes cov- ering components CYP3A41G and CYP3A53 were ob- served to be significantly associated with SCZ in Chi- nese patients [108]. D-Amino acid oxidase activator (DAOA, G72). The DAOA gene locus on chromosome 13q34 has been im- plicated in the etiology of SCZ. 3 SNPs (rs778294, rs779293 and rs3918342) have been identified in this region, and two of them (rs778293, rs3918342) have shown significant transmission disequilibrium and a highly significant under-transmission between haplotype CAT and SCZ. G72 is one of the most widely tested genes for association with SCZ. As G72 activates the D-amino acid oxidase (DAO), G72 is termed D-amino acid oxidase activator (DAOA). Ohi et al. [109] found nominal evidence for association of alleles M22/ rs778293, M23/rs3918342 and M24/rs1421292, and the genotype of M22/rs778293 with SCZ, although there was no association of allele or genotype in the other five SNPs. They also found nominal haplotypic association, including M15/rs2391191 and M19/rs778294, with SCZ; however, these associations were no longer positive after correction for multiple testing, which suggests that G72 might not play a major role in the risk for SCZ in the Japanese population [109]. Association of the G72/G30 locus with SCZ and BD has been reported in several studies. The G72/G30 locus spans a broad region of chromosome 13q. One meta-analysis of published asso- ciation studies shows highly significant evidence of as- sociation between nucleotide variations in the G72/ G30 region and SCZ, along with compelling evidence of as- sociation with BD [110]. This locus has been associated with panic disorder, SCZ, and BD, especially the 3 SNPs rs2391191, rs3918341, and rs1935062, with controver- sial results [111]. G72 is an activator of D-amino acid oxidase (DAO), supporting the glutamate dysfunction hypothesis of SCZ. G72 mRNA is poorly expressed in a variety of human tissues (e.g. adult brain, amygdala, caudate nucleus, fetal brain, spinal cord and testis) from human cell lines or SCZ/control post-mortem BA10 samples. The lack of demonstrable G72 expression in relevant brain regions does not support a role for G72 in modulation of DAO activity and the pathology of SCZ via a DAO-mediated mechanism. In silico analysis sug- gests that G72 is not robustly expressed and that the transcript is potentially labile [112]. Disrupted-in-schizophrenia-1 (DISC1). The dis- rupted-in-schizophrenia-1 (DISC1) locus is located at the breakpoint of a balanced t (1;11) (q42.1; q14.3) chromo- somal translocation in a large and unique Scottish family. This translocation segregates in a highly statistically sig- nificant manner with a broad diagnosis of psychiatric illness, including SCZ, BD and major depression, as well as with a narrow diagnosis of SCZ alone. Two novel genes were identified at this locus and due to the high prevalence of SCZ in this family, they were named dis- rupted-in-schizophrenia-1 (DISC1) and disrupted-inschi- zophrenia-2 (DISC2). DISC1 encodes a novel multi- functional scaffold protein, whereas DISC2 is a putative noncoding RNA gene antisense to DISC1. A number of independent genetic linkage and association studies in diverse populations support the original linkage findings in the Scottish family and genetic evidence now impli- Copyright © 2013 SciRes. OPEN ACCESS
 R. Cacabelos et al. / Open Journal of Psychiatry 2 (2013) 46-139 84 cates the DISC locus in susceptibility to SCZ, schizoaf- fective disorder, BD and major depression as well as various cognitive traits. DISC1 is a hub protein in a mul- tidimensional risk pathway for major mental illness [113]. Many alternatively spliced transcripts were identified, including groups lacking exon 3 (Delta3), exons 7 and 8 (Delta7Delta8), an exon 3 insertion variant (extra short variant-1, Esv1), and intergenic splicing between TSNAX and DISC1. Isoforms Delta7Delta8, Esv1, and Delta3, which encode truncated DISC1 proteins, were expressed more abundantly during fetal development than during postnatal ages, and their expression was higher in the hippocampus of patients with SCZ. SCZ risk-associated polymorphisms [non-synonymous SNPs rs821616 (Cys704Ser) and rs6675281 (Leu607Phe), and rs821597] were associated with the expression of Delta3 and Delta7Delta8 [114]. An inherited 2.07 Mb microdu- plication in 1q42.2 was identified in two brothers with autism and mild mental retardation in Belgium. The du- plication contains seven genes, including the DISC1 gene which has been associated to SCZ, BD, autism and Asperger syndrome [115]. DISC1 regulates neuronal migration and differentiation during mammalian brain development. Enomoto et al. [116] reported that DISC1 interacts with the actin-binding protein girdin to regulate axonal development. Dentate granule cells (DGCs) in girdin-deficient neonatal mice exhibit deficits in axonal sprouting in the cornu ammonis 3 region of the hippo- campus. Girdin deficiency, RNA interference-mediated knockdown, and inhibition of the DISC1/girdin interac- tion lead to overextended migration and mispositioning of the DGCs resulting in profound cytoarchitectural dis- organization of the DG. These findings identify girdin as an intrinsic factor in postnatal development of the DG and provide insights into the critical role of the DISC1/girdin interaction in postnatal neurogenesis in the DG. DISC1 suppression in newborn neurons of the adult hippocampus leads to overactivated signaling of AKT, another SCZ susceptibility gene. Mechanistically, DISC1 directly interacts with KIAA1212, an AKT-binding part- ner that enhances AKT signaling in the absence of DISC1, and DISC1 binding to KIAA1212 prevents AKT activation in vitro. Multiple genetic manipulations to enhance AKT signaling in adult-born neurons in vivo exhibit similar defects to DISC1 suppression in neuronal development, which can be rescued by pharmacological inhibition of mammalian target of rapamycin (mTOR), an AKT downstream effector. The AKT-mTOR signaling pathway acts as a critical DISC1 target in regulating neuronal development [117]. To elucidate how DISC1 confers susceptibility to psy- chiatric disorders, identification of the molecules, which bind to the domain close to the translocation breakpoint in the DISC1 gene, was performed and fasciculation and elongation protein zeta-1 (Fez1), a novel DISC1-inter- acting protein, termed DISC1-binding zinc-finger protein (DBZ), and Kendrin were identified. The DISC1-Fez1 interaction is up-regulated by nerve growth factor (NGF) and involved in neurite extension. Transient dissociation of the DISC1-DBZ interaction by pituitary adenylate cyclase-activating polypeptide (PACAP) causes neurite extension. SNP association studies have shown the rela- tion of the Fez1, PACAP and PACAP receptor (PAC1) genes to SCZ. In SCZ with DISC1 translocation carrier, the DISC1-Fez1 and DISC1-DBZ interaction is disrupted, and it is likely that neural circuit formation remains im- mature, suggesting that SCZ is a neurodevelopmental disease. The DISC1-Kendrin interaction is suggested to be involved in microtubule network formation and an association between SNPs of the Kendrin gene and bipo- lar disease has also been suggested in a Japanese popula- tion [118]. DISC1 interacts directly with phosphodiesterase 4B (PDE4B), an independently identified risk factor for SCZ. DISC1-PDE4B complexes are therefore likely to be in- volved in molecular mechanisms underlying psychiatric illness. PDE4B hydrolyzes cAMP, and DISC1 may regulate cAMP signaling through modulating PDE4B activity. There is evidence that expression of both genes is altered in some psychiatric patients. DISC1 missense mutations that give rise to phenotypes related to SCZ and depression in mice are located within binding sites for PDE4B. These mutations reduce the association between DISC1 and PDE4B, and one results in reduced brain PDE4B activity. Altered DISC1-PDE4B interaction may thus underlie the symptoms of some cases of SCZ and depression [119]. DISC1 protein binding partners include the nuclear distribution factor E homologs (NDE1 and NDEL1), LIS1, and phosphodiesterases 4B and 4D (PDE4B and PDE4D). NDE1, NDEL1 and LIS1, to- gether with their binding partner dynein, associate with DISC1, PDE4B and PDE4D within the cell, and provide evidence that this complex is present at the centrosome. LIS1, NDEL1, DISC1, NDE1, and PDE4B are localized at synapses in cultured neurons. NDE1 is phosphorylated by cAMP-dependent protein kinase A (PKA), whose activity is, in turn, regulated by the cAMP hydrolysis activity of phosphodiesterases, including PDE4. DISC1 might act as an assembly scaffold for all of these proteins and the NDE1/NDEL1/LIS1/dynein complex might be modulated by cAMP levels via PKA and PDE4 [120]. A DISC1 haplotype, HEP3, and an NDE1 spanning tag haplotype are associated with SCZ in Finnish fami- lies. Tomppo et al. [121] identified three SNPs as being associated with SCZ in PDE4D (rs1120303), PDE4B (rs7412571), and NDEL1 (rs17806986). Greater signifi- cance was observed with allelic haplotypes of PDE4D, PDE4B, and NDEL1 that increased or decreased SCZ Copyright © 2013 SciRes. OPEN ACCESS
 R. Cacabelos et al. / Open Journal of Psychiatry 2 (2013) 46-139 85 susceptibility, highlighting the potential importance of DISC1-related molecular pathways in the etiology of SCZ and other major mental illnesses [121]. DISC1 is expressed in cranial neural crest (CNC) cells. Loss of Disc1 resulted in persistent CNC cell medial migration, dorsal to the developing neural epithelium, and hindered migration away from the region dorsal to the neural rod. The failure of CNC cells to migrate away from the neural rod correlated with the enhanced expres- sion of two transcription factors, foxd3 and sox10. These transcription factors have many functions in CNC cells, including the maintenance of precursor pools, timing of migration onset, and the induction of cell differentiation. DISC1 functions in the transcriptional repression of foxd3 and sox10, thus mediating CNC cell migration and differentiation [122]. DISC1 is widely expressed in cortical and limbic re- gions. Association between the DISC1 Ser704Cys poly- morphism and volumetric measurements for a broad range of fronto-parietal, temporal, and limbic-paralimbic regions was studied in Japanese patients with SCZ using magnetic resonance imaging. The Cys carriers had sig- nificantly larger volumes of the medial superior frontal gyrus and shorter insular cortex than the Ser homozy- gotes, but only in healthy comparison subjects. The Cys carriers tended to have a smaller supramarginal gyrus than the Ser homozygotes in SCZ patients, but not in healthy comparison subjects. The right medial superior frontal gyrus volume was significantly correlated with daily dosage of antipsychotic medication in Ser homo- zygote SCZ patients. These different genotype effects of the DISC1 Ser704Cys polymorphism on the brain mor- phology in SCZ suggest that variation in the DISC1 gene might be involved in the neurobiology of SCZ [123]. Schumacher et al. [124] found evidence for a common SCZ risk interval within DISC1 intron 4 - 6. DNA methyltransferases (DNMTs). Aberrant DNA methylation may be involved in the development of SCZ. DNA methyltransferase 3B (DNMT3B) is the key me- thyltransferase in DNA methylation regulations. Case- control and family-based studies were performed through genotyping two tag SNPs (rs2424908 and rs6119954) covering the whole DNMT3B gene. The frequency of G allele of rs6119954 was significantly higher in SCZ. Genotype distribution of rs6119954 was significantly different between patients and controls. A haplotype-wise analysis revealed a higher frequency of the T-G (rs2424908-rs6119954) haplotype in SCZ. In the trans- mission disequilibrium test analysis, the G allele of rs6119954 was preferentially transmitted in the trios. According to these findings reported by Zhang et al. [125] in Chinese patients, DNMT3B may be a candidate gene for susceptibility to early onset SCZ. In SCZ, a functional downregulation of the prefrontal cortex GABAergic neuronal system is mediated by a promoter hypermethylation, presumably catalyzed by an increase in DNA-methyltransferase-1 (DNMT-1) expression. This promoter hypermethylation may be mediated not only by DNMT-1 but also by an entire family of de novo DNA-methyltransferases, such as DNA-methyltrans- ferase-3a (DNMT-3a) and -3b (DNMT-3b). There is an overexpression of DNMT-3a and DNMT-3b in Brod- mann’s area 10 (BA10) and in the caudate nucleus and putamen of SCZ brains. DNMT-3a and DNMT-1 are expressed and co-localize in distinct GABAergic neuron populations whereas DNMT-3b mRNA is virtually un- detectable. Unlike DNMT-1, which is frequently overex- pressed in telencephalic GABAergic neurons of SCZ, DNMT-3a mRNA is overexpressed only in layer I and II GABAergic interneurons of BA10. DNMT-1 and DNMT- 3a mRNAs are also overexpressed in peripheral blood lymphocytes of SCZ patients. The upregulation of DNMT-1 and to a lesser extent that of DNMT-3a mRNA in lymphocytes of SCZ supports the concept that this readily available peripheral cell type can express an epi- genetic variation of specific biomarkers relevant to SCZ morbidity [126]. Dopamine-Related Genes DARPP-32 (PPP1R1B). Recent findings have highlighted the importance of DARPP-32 (dopa- mine- and cAMP-regulated phosphoprotein, 32 kDa), a key regulatory molecule in the dopaminer- gic signaling pathway for dopamine-related phenol- types such as antisocial behavior, drug addiction and SCZ. Reuter et al. [127] reported the first study investigating the role of the DARPP-32 gene for personality. In a sample of healthy German Cauca- sian subjects they found a significant association between rs907094 and anger. Carriers of the T-allele showed significantly higher anger scores than participants without a T-allele. A negative as- sociation between ANGER scores and the volume of the left amygdala was also detected [127]. Dopamine beta-hydroxylase. The SNP rs1108580 A/G in DBH has been associated with SCZ [98]. Dopamine transporter (DAT; SLC6A3) 3’ UTR VNTR. Dopamine has a crucial role in the modula- tion of neurocognitive function, and synaptic do- pamine activity is normally regulated by the dopa- mine transporter (DAT) and catechol-O-methyl- transferase (COMT). Altered dopamine function is a key pathophysiological feature of SCZ. Prata et al. [128] examined epistasis between the DAT 3’ UTR variable number of tandem repeats (VNTR) and Copyright © 2013 SciRes. OPEN ACCESS
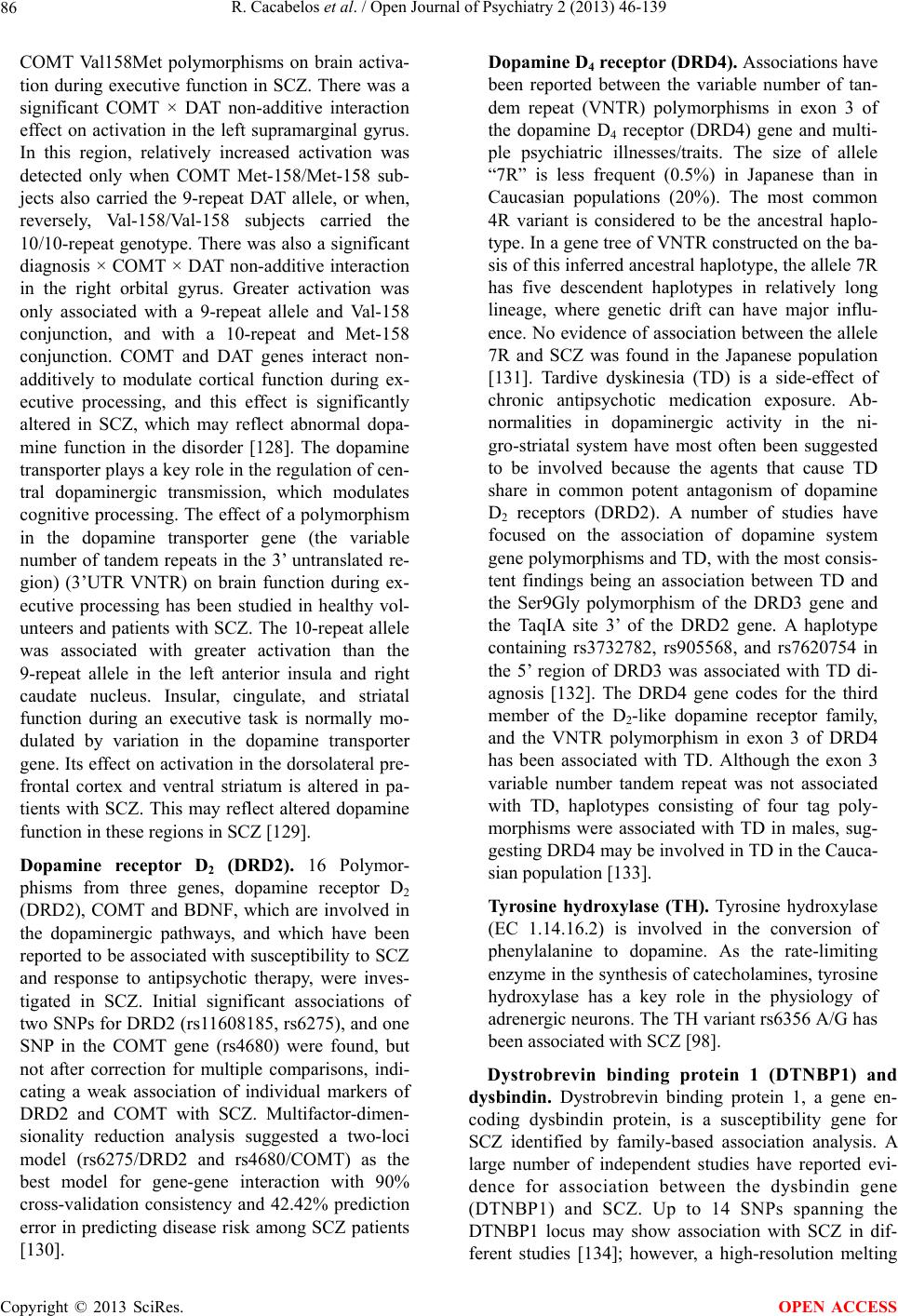 R. Cacabelos et al. / Open Journal of Psychiatry 2 (2013) 46-139 86 COMT Val158Met polymorphisms on brain activa- tion during executive function in SCZ. There was a significant COMT × DAT non-additive interaction effect on activation in the left supramarginal gyrus. In this region, relatively increased activation was detected only when COMT Met-158/Met-158 sub- jects also carried the 9-repeat DAT allele, or when, reversely, Val-158/Val-158 subjects carried the 10/10-repeat genotype. There was also a significant diagnosis × COMT × DAT non-additive interaction in the right orbital gyrus. Greater activation was only associated with a 9-repeat allele and Val-158 conjunction, and with a 10-repeat and Met-158 conjunction. COMT and DAT genes interact non- additively to modulate cortical function during ex- ecutive processing, and this effect is significantly altered in SCZ, which may reflect abnormal dopa- mine function in the disorder [128]. The dopamine transporter plays a key role in the regulation of cen- tral dopaminergic transmission, which modulates cognitive processing. The effect of a polymorphism in the dopamine transporter gene (the variable number of tandem repeats in the 3’ untranslated re- gion) (3’UTR VNTR) on brain function during ex- ecutive processing has been studied in healthy vol- unteers and patients with SCZ. The 10-repeat allele was associated with greater activation than the 9-repeat allele in the left anterior insula and right caudate nucleus. Insular, cingulate, and striatal function during an executive task is normally mo- dulated by variation in the dopamine transporter gene. Its effect on activation in the dorsolateral pre- frontal cortex and ventral striatum is altered in pa- tients with SCZ. This may reflect altered dopamine function in these regions in SCZ [129]. Dopamine receptor D2 (DRD2). 16 Polymor- phisms from three genes, dopamine receptor D2 (DRD2), COMT and BDNF, which are involved in the dopaminergic pathways, and which have been reported to be associated with susceptibility to SCZ and response to antipsychotic therapy, were inves- tigated in SCZ. Initial significant associations of two SNPs for DRD2 (rs11608185, rs6275), and one SNP in the COMT gene (rs4680) were found, but not after correction for multiple comparisons, indi- cating a weak association of individual markers of DRD2 and COMT with SCZ. Multifactor-dimen- sionality reduction analysis suggested a two-loci model (rs6275/DRD2 and rs4680/COMT) as the best model for gene-gene interaction with 90% cross-validation consistency and 42.42% prediction error in predicting disease risk among SCZ patients [130]. Dopamine D4 receptor (DRD4). Associations have been reported between the variable number of tan- dem repeat (VNTR) polymorphisms in exon 3 of the dopamine D4 receptor (DRD4) gene and multi- ple psychiatric illnesses/traits. The size of allele “7R” is less frequent (0.5%) in Japanese than in Caucasian populations (20%). The most common 4R variant is considered to be the ancestral haplo- type. In a gene tree of VNTR constructed on the ba- sis of this inferred ancestral haplotype, the allele 7R has five descendent haplotypes in relatively long lineage, where genetic drift can have major influ- ence. No evidence of association between the allele 7R and SCZ was found in the Japanese population [131]. Tardive dyskinesia (TD) is a side-effect of chronic antipsychotic medication exposure. Ab- normalities in dopaminergic activity in the ni- gro-striatal system have most often been suggested to be involved because the agents that cause TD share in common potent antagonism of dopamine D2 receptors (DRD2). A number of studies have focused on the association of dopamine system gene polymorphisms and TD, with the most consis- tent findings being an association between TD and the Ser9Gly polymorphism of the DRD3 gene and the TaqIA site 3’ of the DRD2 gene. A haplotype containing rs3732782, rs905568, and rs7620754 in the 5’ region of DRD3 was associated with TD di- agnosis [132]. The DRD4 gene codes for the third member of the D2-like dopamine receptor family, and the VNTR polymorphism in exon 3 of DRD4 has been associated with TD. Although the exon 3 variable number tandem repeat was not associated with TD, haplotypes consisting of four tag poly- morphisms were associated with TD in males, sug- gesting DRD4 may be involved in TD in the Cauca- sian population [133]. Tyrosine hydroxylase (TH). Tyrosine hydroxylase (EC 1.14.16.2) is involved in the conversion of phenylalanine to dopamine. As the rate-limiting enzyme in the synthesis of catecholamines, tyrosine hydroxylase has a key role in the physiology of adrenergic neurons. The TH variant rs6356 A/G has been associated with SCZ [98]. Dystrobrevin binding protein 1 (DTNBP1) and dysbindin. Dystrobrevin binding protein 1, a gene en- coding dysbindin protein, is a susceptibility gene for SCZ identified by family-based association analysis. A large number of independent studies have reported evi- dence for association between the dysbindin gene (DTNBP1) and SCZ. Up to 14 SNPs spanning the DTNBP1 locus may show association with SCZ in dif- ferent studies [134]; however, a high-resolution melting Copyright © 2013 SciRes. OPEN ACCESS
 R. Cacabelos et al. / Open Journal of Psychiatry 2 (2013) 46-139 87 analysis (HRMA) to screen the 11 DTNBP1 exons with their corresponding DNA variants in a sample from the UK revealed no significant associations with SCZ [135]. DTNBP1 and MUTED encode proteins that belong to the endosome-localized biogenesis of lysosome-related organelles complex-1 (BLOC-1). BLOC-1 plays a key role in endosomal trafficking and as such has been found to regulate cell-surface abundance of the D2 dopamine receptor, the biogenesis and fusion of synaptic vesicles, and neurite outgrowth. These functions are pertinent to both neurodevelopment and synaptic transmission, proc- esses tightly regulated by selective cell-surface delivery of membrane proteins to and from endosomes. It has been proposed that cellular processes, such as endosomal trafficking, act as convergence points in which multiple small effects from polygenic genetic polymorphisms accumulate to promote the development of SCZ [136]. BLOC-1 physically interacts with the adaptor protein AP-3 complex, which is essential for vesicle or protein sorting. Dysbindin forms a complex with the AP-3 com- plex through the direct binding to its µ subunit. Dys- bindin partially co-localized with the AP-3 complex in CA1 and CA3 of mouse hippocampus, and at presynaptic terminals and axonal growth cones of cultured hippo- campal neurons. Suppression of dysbindin results in the reduction of presynaptic protein expression and gluta- mate release. Thus, dysbindin appears to participate in the exocytosis or sorting of the synaptic vesicle via direct interaction with the AP-3 complex [137]. Dysbindin is involved in the exocytosis and/or formation of synaptic vesicles. Proteins involved in protein localization process, including Munc18-1, were identified as dysbindin-in- teracting proteins [138]. A three-marker C-A-T dysbindin haplotype is associated with increased risk for SCZ, de- creased mRNA expression, reduced gray matter volume in both the right dorsolateral prefrontal and left occipital cortex, poorer cognitive performance, and early sensory processing deficits [139]. 4 SNPs (rs3213207, rs1011313, rs760761, and rs2619522) have been genotyped in a large Korean SCZ sample. Haplotype analyses revealed a significant association with SCZ with the haplotypes A-C-C-C and A-C-T-A having an eminent protective effect toward SCZ. The major contribution to the differ- ence in the haplotype distribution between patients and the controls was the rs760761 (C/T) and rs2619522 (A/C) haplotypes. No association of DTNBP1 with other clini- cal variables was found. This study suggests a possible protective effect of rare DTNBP1 variants in SCZ [140]. Recent studies suggest a degree of overlap in genetic susceptibility across the traditional categories of SCZ and BD. DTNBP1 has also become a focus of investiga- tion in BD. Seven DTNBP1 SNPs: rs2743852 (SNP C), rs760761 (P1320), rs1011313 (P1325), rs3213207 (P1635), rs2619539 (P1655), rs16876571 and rs17470454, were investigated using the SNPlex genotyping system. Significant differences in genotypic and allelic frequen- cies of rs3213207 and rs760761 of DTNBP1 were found between bipolar patients and controls, as well as a global haplotypic association and an association of a particular haplotype with BD [141]. ErbB4 (v-Erb-a erythroblastic leukemia viral on- cogene homolog 4 (avian)). ErbB4 is a growth factor receptor tyrosine kinase essential for neurodevelopment. Genetic variation in ErbB4 is associated with SCZ. Risk-associated polymorphisms predict overexpression of ErbB4 CYT-1 isoforms in the brain of schizophrenic patients. The molecular mechanism of association is un- clear because the polymorphisms flank exon 3 of the gene and reside 700 kb distal to the CYT-1 defining exon. Tan et al. [142] hypothesized that the polymorphisms are indirectly associated with ErbB4 CYT-1 via splicing of exon 3 on the CYT-1 background. They identified novel splice isoforms of ErbB4, whereby exon 3 is skipped (del.3). ErbB4 del.3 transcripts exist as CYT-2 isoforms and are predicted to produce truncated proteins. Jux- tamembrane (JM) splice variants of ErbB4, JM-a and JM-b respectively, are characterized by the replacement of a 75 nucleotide (nt) sequence with a 45-nt insertion, and represent four alternative exons in the gene. Novel splice variants of ErbB4 exist in the developing and adult human brain and, given the failure to identify ErbB4 del3 CYT-1 transcripts, suggest that the association of risk polymorphisms in the ErbB4 gene with CYT-1 transcript levels is not mediated via an exon 3 splicing event, ac- cording to Tan and coworkers [142]. Estrogen signaling. Estrogen signaling may be altered in the brains of people with SCZ. DNA sequence varia- tion in the estrogen receptor (ER) alpha gene (ESR1), lower ERalpha mRNA levels, and/or blunted ERalpha signaling is associated with SCZ. The naturally-occur- ring truncated ERalpha isoform, Delta7, which acts as a dominant negative, can attenuate gene expression in- duced by the wild-type (WT) receptor in an estro-gen- dependent manner in neuronal (SHSY5Y) and non-neu- ronal (CHOK1 and HeLa) cells. ERalpha may also in- teract with NRG1-ErbB4, a leading SCZ susceptibility pathway. Reductions in the transcriptionally active form of ErbB4 comprising the intracytoplasmic domain (ErbB4- ICD) have been found in SCZ, and ERalpha and ErbB4 may converge to control gene expression. ErbB4-ICD can potentiate the transcriptional activity of WT-ERalpha at EREs in two cell lines and this potentiation effect is abolished by the presence of Delta7-ERalpha. Conver- gence between ERalpha and ErbB4-ICD in the transcrip- tional control of ERalpha-target gene expression may represent a convergent pathway that may be disrupted in SCZ [143]. Studies in Japan reported no association be- tween ERBB3 and SCZ in the Japanese population. Li et Copyright © 2013 SciRes. OPEN ACCESS
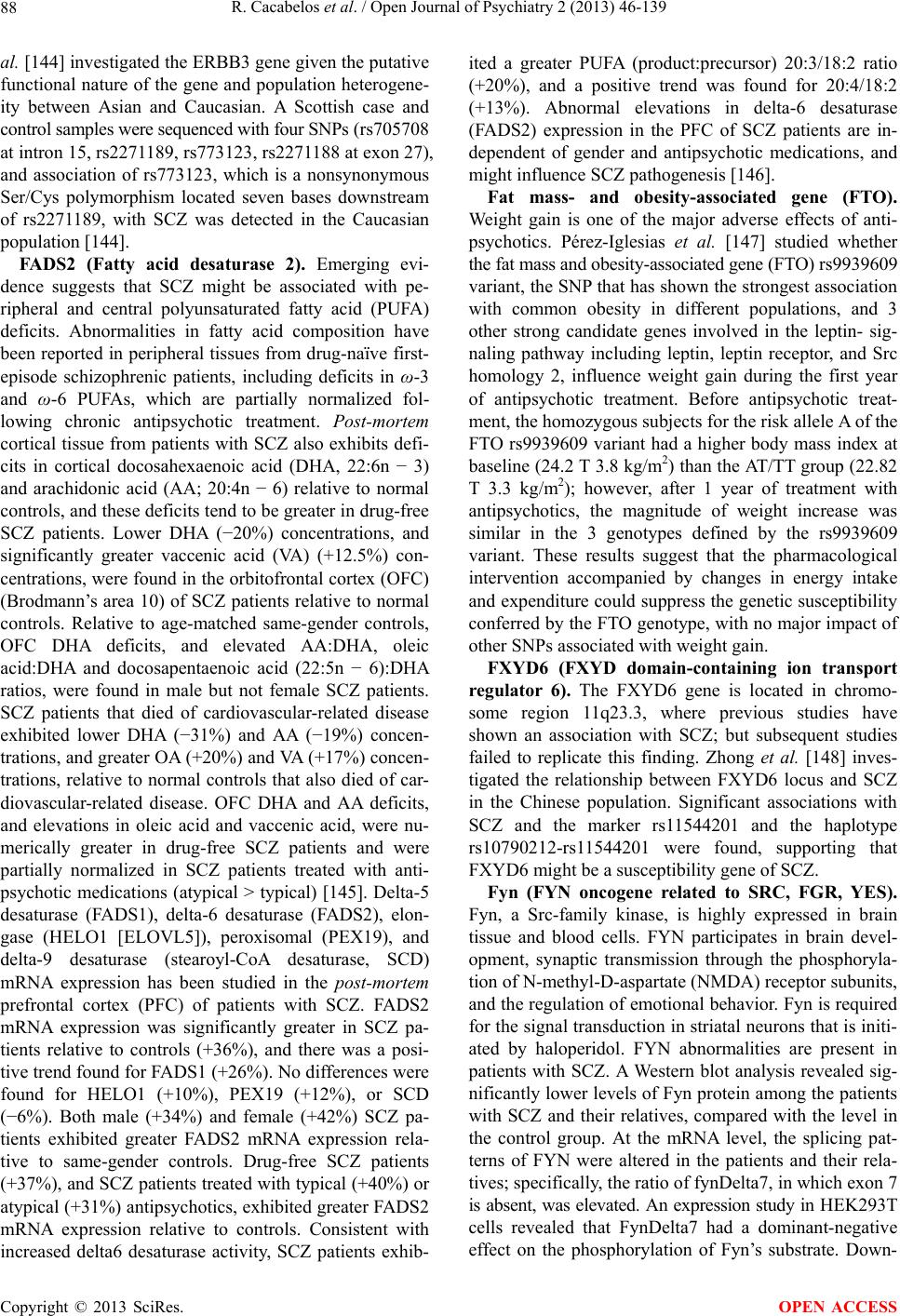 R. Cacabelos et al. / Open Journal of Psychiatry 2 (2013) 46-139 88 al. [144] investigated the ERBB3 gene given the putative functional nature of the gene and population heterogene- ity between Asian and Caucasian. A Scottish case and control samples were sequenced with four SNPs (rs705708 at intron 15, rs2271189, rs773123, rs2271188 at exon 27), and association of rs773123, which is a nonsynonymous Ser/Cys polymorphism located seven bases downstream of rs2271189, with SCZ was detected in the Caucasian population [144]. FADS2 (Fatty acid desaturase 2). Emerging evi- dence suggests that SCZ might be associated with pe- ripheral and central polyunsaturated fatty acid (PUFA) deficits. Abnormalities in fatty acid composition have been reported in peripheral tissues from drug-naïve first- episode schizophrenic patients, including deficits in ω-3 and ω-6 PUFAs, which are partially normalized fol- lowing chronic antipsychotic treatment. Post-mortem cortical tissue from patients with SCZ also exhibits defi- cits in cortical docosahexaenoic acid (DHA, 22:6n − 3) and arachidonic acid (AA; 20:4n − 6) relative to normal controls, and these deficits tend to be greater in drug-free SCZ patients. Lower DHA (−20%) concentrations, and significantly greater vaccenic acid (VA) (+12.5%) con- centrations, were found in the orbitofrontal cortex (OFC) (Brodmann’s area 10) of SCZ patients relative to normal controls. Relative to age-matched same-gender controls, OFC DHA deficits, and elevated AA:DHA, oleic acid:DHA and docosapentaenoic acid (22:5n − 6):DHA ratios, were found in male but not female SCZ patients. SCZ patients that died of cardiovascular-related disease exhibited lower DHA (−31%) and AA (−19%) concen- trations, and greater OA (+20%) and VA (+17%) concen- trations, relative to normal controls that also died of car- diovascular-related disease. OFC DHA and AA deficits, and elevations in oleic acid and vaccenic acid, were nu- merically greater in drug-free SCZ patients and were partially normalized in SCZ patients treated with anti- psychotic medications (atypical > typical) [145]. Delta-5 desaturase (FADS1), delta-6 desaturase (FADS2), elon- gase (HELO1 [ELOVL5]), peroxisomal (PEX19), and delta-9 desaturase (stearoyl-CoA desaturase, SCD) mRNA expression has been studied in the post-mortem prefrontal cortex (PFC) of patients with SCZ. FADS2 mRNA expression was significantly greater in SCZ pa- tients relative to controls (+36%), and there was a posi- tive trend found for FADS1 (+26%). No differences were found for HELO1 (+10%), PEX19 (+12%), or SCD (−6%). Both male (+34%) and female (+42%) SCZ pa- tients exhibited greater FADS2 mRNA expression rela- tive to same-gender controls. Drug-free SCZ patients (+37%), and SCZ patients treated with typical (+40%) or atypical (+31%) antipsychotics, exhibited greater FADS2 mRNA expression relative to controls. Consistent with increased delta6 desaturase activity, SCZ patients exhib- ited a greater PUFA (product:precursor) 20:3/18:2 ratio (+20%), and a positive trend was found for 20:4/18:2 (+13%). Abnormal elevations in delta-6 desaturase (FADS2) expression in the PFC of SCZ patients are in- dependent of gender and antipsychotic medications, and might influence SCZ pathogenesis [146]. Fat mass- and obesity-associated gene (FTO). Weight gain is one of the major adverse effects of anti- psychotics. Pérez-Iglesias et al. [147] studied whether the fat mass and obesity-associated gene (FTO) rs9939609 variant, the SNP that has shown the strongest association with common obesity in different populations, and 3 other strong candidate genes involved in the leptin- sig- naling pathway including leptin, leptin receptor, and Src homology 2, influence weight gain during the first year of antipsychotic treatment. Before antipsychotic treat- ment, the homozygous subjects for the risk allele A of the FTO rs9939609 variant had a higher body mass index at baseline (24.2 T 3.8 kg/m2) than the AT/TT group (22.82 T 3.3 kg/m2); however, after 1 year of treatment with antipsychotics, the magnitude of weight increase was similar in the 3 genotypes defined by the rs9939609 variant. These results suggest that the pharmacological intervention accompanied by changes in energy intake and expenditure could suppress the genetic susceptibility conferred by the FTO genotype, with no major impact of other SNPs associated with weight gain. FXYD6 (FXYD domain-containing ion transport regulator 6). The FXYD6 gene is located in chromo- some region 11q23.3, where previous studies have shown an association with SCZ; but subsequent studies failed to replicate this finding. Zhong et al. [148] inves- tigated the relationship between FXYD6 locus and SCZ in the Chinese population. Significant associations with SCZ and the marker rs11544201 and the haplotype rs10790212-rs11544201 were found, supporting that FXYD6 might be a susceptibility gene of SCZ. Fyn (FYN oncogene related to SRC, FGR, YES). Fyn, a Src-family kinase, is highly expressed in brain tissue and blood cells. FYN participates in brain devel- opment, synaptic transmission through the phosphoryla- tion of N-methyl-D-aspartate (NMDA) receptor subunits, and the regulation of emotional behavior. Fyn is required for the signal transduction in striatal neurons that is initi- ated by haloperidol. FYN abnormalities are present in patients with SCZ. A Western blot analysis revealed sig- nificantly lower levels of Fyn protein among the patients with SCZ and their relatives, compared with the level in the control group. At the mRNA level, the splicing pat- terns of FYN were altered in the patients and their rela- tives; specifically, the ratio of fynDelta7, in which exon 7 is absent, was elevated. An expression study in HEK293T cells revealed that FynDelta7 had a dominant-negative effect on the phosphorylation of Fyn’s substrate. Down- Copyright © 2013 SciRes. OPEN ACCESS
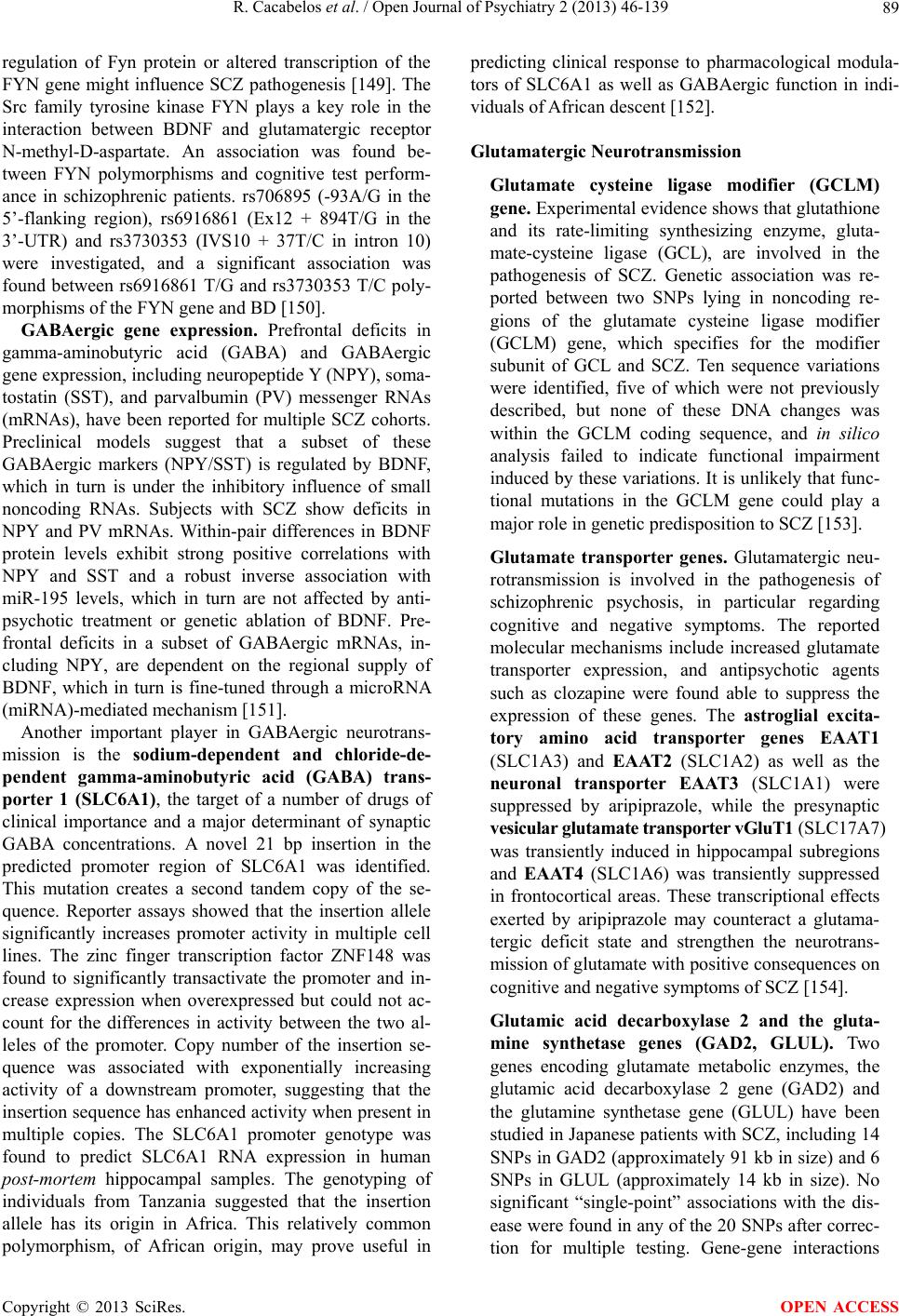 R. Cacabelos et al. / Open Journal of Psychiatry 2 (2013) 46-139 89 regulation of Fyn protein or altered transcription of the FYN gene might influence SCZ pathogenesis [149]. The Src family tyrosine kinase FYN plays a key role in the interaction between BDNF and glutamatergic receptor N-methyl-D-aspartate. An association was found be- tween FYN polymorphisms and cognitive test perform- ance in schizophrenic patients. rs706895 (-93A/G in the 5’-flanking region), rs6916861 (Ex12 + 894T/G in the 3’-UTR) and rs3730353 (IVS10 + 37T/C in intron 10) were investigated, and a significant association was found between rs6916861 T/G and rs3730353 T/C poly- morphisms of the FYN gene and BD [150]. GABAergic gene expression. Prefrontal deficits in gamma-aminobutyric acid (GABA) and GABAergic gene expression, including neuropeptide Y (NPY), soma- tostatin (SST), and parvalbumin (PV) messenger RNAs (mRNAs), have been reported for multiple SCZ cohorts. Preclinical models suggest that a subset of these GABAergic markers (NPY/SST) is regulated by BDNF, which in turn is under the inhibitory influence of small noncoding RNAs. Subjects with SCZ show deficits in NPY and PV mRNAs. Within-pair differences in BDNF protein levels exhibit strong positive correlations with NPY and SST and a robust inverse association with miR-195 levels, which in turn are not affected by anti- psychotic treatment or genetic ablation of BDNF. Pre- frontal deficits in a subset of GABAergic mRNAs, in- cluding NPY, are dependent on the regional supply of BDNF, which in turn is fine-tuned through a microRNA (miRNA)-mediated mechanism [151]. Another important player in GABAergic neurotrans- mission is the sodium-dependent and chloride-de- pendent gamma-aminobutyric acid (GABA) trans- porter 1 (SLC6A1), the target of a number of drugs of clinical importance and a major determinant of synaptic GABA concentrations. A novel 21 bp insertion in the predicted promoter region of SLC6A1 was identified. This mutation creates a second tandem copy of the se- quence. Reporter assays showed that the insertion allele significantly increases promoter activity in multiple cell lines. The zinc finger transcription factor ZNF148 was found to significantly transactivate the promoter and in- crease expression when overexpressed but could not ac- count for the differences in activity between the two al- leles of the promoter. Copy number of the insertion se- quence was associated with exponentially increasing activity of a downstream promoter, suggesting that the insertion sequence has enhanced activity when present in multiple copies. The SLC6A1 promoter genotype was found to predict SLC6A1 RNA expression in human post-mortem hippocampal samples. The genotyping of individuals from Tanzania suggested that the insertion allele has its origin in Africa. This relatively common polymorphism, of African origin, may prove useful in predicting clinical response to pharmacological modula- tors of SLC6A1 as well as GABAergic function in indi- viduals of African descent [152]. Glutamatergic Neurotransmission Glutamate cysteine ligase modifier (GCLM) gene. Experimental evidence shows that glutathione and its rate-limiting synthesizing enzyme, gluta- mate-cysteine ligase (GCL), are involved in the pathogenesis of SCZ. Genetic association was re- ported between two SNPs lying in noncoding re- gions of the glutamate cysteine ligase modifier (GCLM) gene, which specifies for the modifier subunit of GCL and SCZ. Ten sequence variations were identified, five of which were not previously described, but none of these DNA changes was within the GCLM coding sequence, and in silico analysis failed to indicate functional impairment induced by these variations. It is unlikely that func- tional mutations in the GCLM gene could play a major role in genetic predisposition to SCZ [153]. Glutamate transporter genes. Glutamatergic neu- rotransmission is involved in the pathogenesis of schizophrenic psychosis, in particular regarding cognitive and negative symptoms. The reported molecular mechanisms include increased glutamate transporter expression, and antipsychotic agents such as clozapine were found able to suppress the expression of these genes. The astroglial excita- tory amino acid transporter genes EAAT1 (SLC1A3) and EAAT2 (SLC1A2) as well as the neuronal transporter EAAT3 (SLC1A1) were suppressed by aripiprazole, while the presynaptic vesicular glutamate transporter vGluT1 (SLC17A7) was transiently induced in hippocampal subregions and EAAT4 (SLC1A6) was transiently suppressed in frontocortical areas. These transcriptional effects exerted by aripiprazole may counteract a glutama- tergic deficit state and strengthen the neurotrans- mission of glutamate with positive consequences on cognitive and negative symptoms of SCZ [154]. Glutamic acid decarboxylase 2 and the gluta- mine synthetase genes (GAD2, GLUL). Two genes encoding glutamate metabolic enzymes, the glutamic acid decarboxylase 2 gene (GAD2) and the glutamine synthetase gene (GLUL) have been studied in Japanese patients with SCZ, including 14 SNPs in GAD2 (approximately 91 kb in size) and 6 SNPs in GLUL (approximately 14 kb in size). No significant “single-point” associations with the dis- ease were found in any of the 20 SNPs after correc- tion for multiple testing. Gene-gene interactions Copyright © 2013 SciRes. OPEN ACCESS
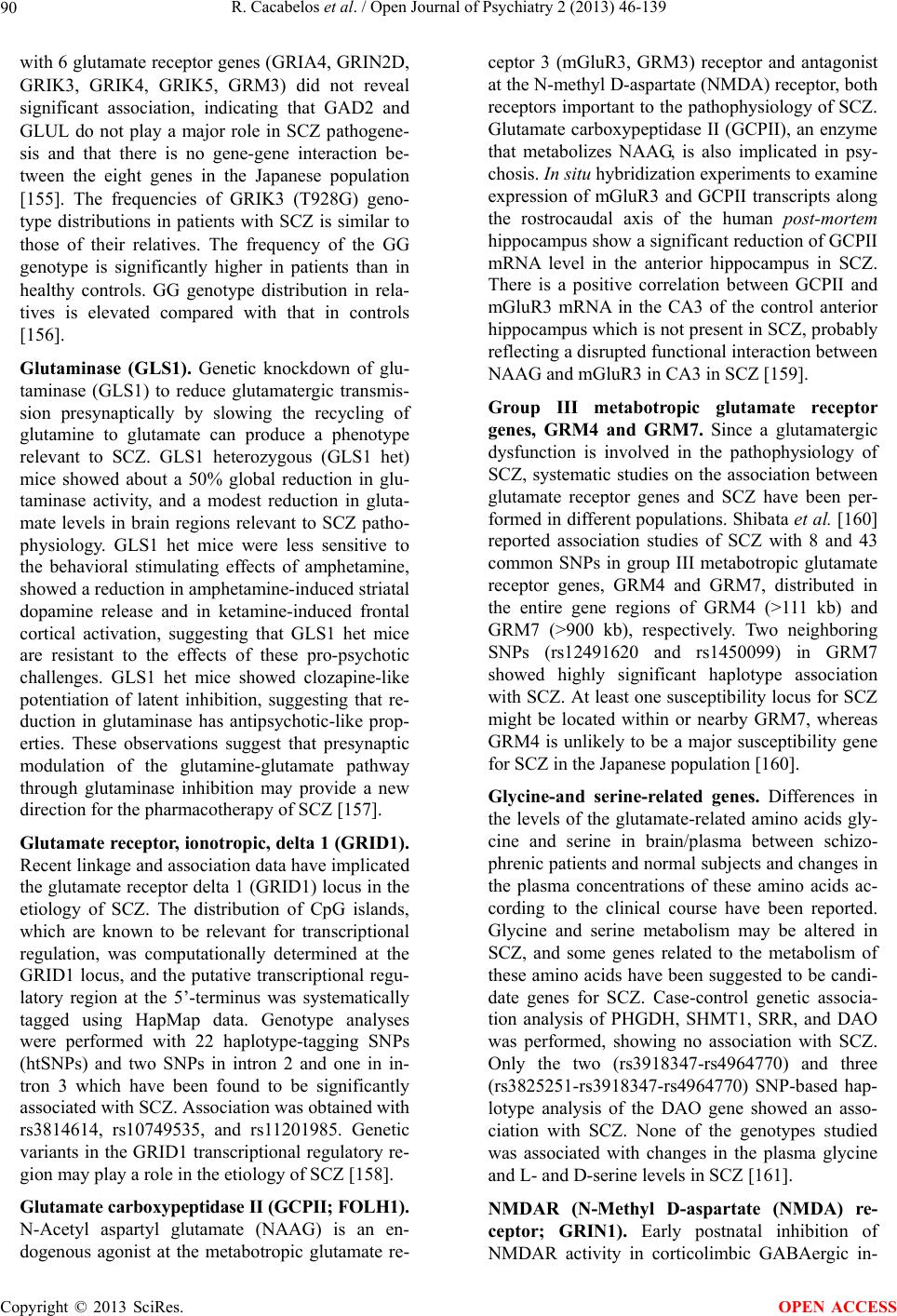 R. Cacabelos et al. / Open Journal of Psychiatry 2 (2013) 46-139 90 with 6 glutamate receptor genes (GRIA4, GRIN2D, GRIK3, GRIK4, GRIK5, GRM3) did not reveal significant association, indicating that GAD2 and GLUL do not play a major role in SCZ pathogene- sis and that there is no gene-gene interaction be- tween the eight genes in the Japanese population [155]. The frequencies of GRIK3 (T928G) geno- type distributions in patients with SCZ is similar to those of their relatives. The frequency of the GG genotype is significantly higher in patients than in healthy controls. GG genotype distribution in rela- tives is elevated compared with that in controls [156]. Glutaminase (GLS1). Genetic knockdown of glu- taminase (GLS1) to reduce glutamatergic transmis- sion presynaptically by slowing the recycling of glutamine to glutamate can produce a phenotype relevant to SCZ. GLS1 heterozygous (GLS1 het) mice showed about a 50% global reduction in glu- taminase activity, and a modest reduction in gluta- mate levels in brain regions relevant to SCZ patho- physiology. GLS1 het mice were less sensitive to the behavioral stimulating effects of amphetamine, showed a reduction in amphetamine-induced striatal dopamine release and in ketamine-induced frontal cortical activation, suggesting that GLS1 het mice are resistant to the effects of these pro-psychotic challenges. GLS1 het mice showed clozapine-like potentiation of latent inhibition, suggesting that re- duction in glutaminase has antipsychotic-like prop- erties. These observations suggest that presynaptic modulation of the glutamine-glutamate pathway through glutaminase inhibition may provide a new direction for the pharmacotherapy of SCZ [157]. Glutamate receptor, ionotropic, delta 1 (GRID1). Recent linkage and association data have implicated the glutamate receptor delta 1 (GRID1) locus in the etiology of SCZ. The distribution of CpG islands, which are known to be relevant for transcriptional regulation, was computationally determined at the GRID1 locus, and the putative transcriptional regu- latory region at the 5’-terminus was systematically tagged using HapMap data. Genotype analyses were performed with 22 haplotype-tagging SNPs (htSNPs) and two SNPs in intron 2 and one in in- tron 3 which have been found to be significantly associated with SCZ. Association was obtained with rs3814614, rs10749535, and rs11201985. Genetic variants in the GRID1 transcriptional regulatory re- gion may play a role in the etiology of SCZ [158]. Glutamate carboxypeptidase II (GCPII; FOLH1). N-Acetyl aspartyl glutamate (NAAG) is an en- dogenous agonist at the metabotropic glutamate re- ceptor 3 (mGluR3, GRM3) receptor and antagonist at the N-methyl D-aspartate (NMDA) receptor, both receptors important to the pathophysiology of SCZ. Glutamate carboxypeptidase II (GCPII), an enzyme that metabolizes NAAG, is also implicated in psy- chosis. In situ hybridization experiments to examine expression of mGluR3 and GCPII transcripts along the rostrocaudal axis of the human post-mortem hippocampus show a significant reduction of GCPII mRNA level in the anterior hippocampus in SCZ. There is a positive correlation between GCPII and mGluR3 mRNA in the CA3 of the control anterior hippocampus which is not present in SCZ, probably reflecting a disrupted functional interaction between NAAG and mGluR3 in CA3 in SCZ [159]. Group III metabotropic glutamate receptor genes, GRM4 and GRM7. Since a glutamatergic dysfunction is involved in the pathophysiology of SCZ, systematic studies on the association between glutamate receptor genes and SCZ have been per- formed in different populations. Shibata et al. [160] reported association studies of SCZ with 8 and 43 common SNPs in group III metabotropic glutamate receptor genes, GRM4 and GRM7, distributed in the entire gene regions of GRM4 (>111 kb) and GRM7 (>900 kb), respectively. Two neighboring SNPs (rs12491620 and rs1450099) in GRM7 showed highly significant haplotype association with SCZ. At least one susceptibility locus for SCZ might be located within or nearby GRM7, whereas GRM4 is unlikely to be a major susceptibility gene for SCZ in the Japanese population [160]. Glycine-and serine-related genes. Differences in the levels of the glutamate-related amino acids gly- cine and serine in brain/plasma between schizo- phrenic patients and normal subjects and changes in the plasma concentrations of these amino acids ac- cording to the clinical course have been reported. Glycine and serine metabolism may be altered in SCZ, and some genes related to the metabolism of these amino acids have been suggested to be candi- date genes for SCZ. Case-control genetic associa- tion analysis of PHGDH, SHMT1, SRR, and DAO was performed, showing no association with SCZ. Only the two (rs3918347-rs4964770) and three (rs3825251-rs3918347-rs4964770) SNP-based hap- lotype analysis of the DAO gene showed an asso- ciation with SCZ. None of the genotypes studied was associated with changes in the plasma glycine and L- and D-serine levels in SCZ [161]. NMDAR (N-Methyl D-aspartate (NMDA) re- ceptor; GRIN1). Early postnatal inhibition of NMDAR activity in corticolimbic GABAergic in- Copyright © 2013 SciRes. OPEN ACCESS
 R. Cacabelos et al. / Open Journal of Psychiatry 2 (2013) 46-139 91 terneurons contributes to the pathophysiology of SCZ-related disorders [162]. Consistent with the NMDAR hypofunction theory of SCZ, in animal models in which the essential NR1 subunit of the NMDA receptor (NMDAR) was selectively elimi- nated in 40% - 50% of cortical and hippocampal interneurons in early postnatal development, dis- tinct SCZ-related symptoms emerged after adoles- cence, including novelty-induced hyperlocomotion, mating and nest-building deficits, as well as anhe- donia-like and anxiety-like behaviors. Social mem- ory, spatial working memory and prepulse inhibi- tion were also impaired. Reduced expression of glutamic acid decarboxylase 67 and parvalbumin was accompanied by disinhibition of cortical exci- tatory neurons and reduced neuronal synchrony [162]. Phosphorylation of the NR1 subunit of NMDA re- ceptors (NMDARs) at serine (S) 897 is markedly reduced in SCZ patients. Knock-in mutations (mice in which the NR1 S897 is replaced with alanine) cause severe impairment in NMDAR synaptic in- corporation and NMDAR-mediated synaptic trans- mission. Phosphomutant animals have reduced AMPA receptor (AMPAR)-mediated synaptic trans- mission, decreased AMPARGluR1 subunit in the synapse, impaired long-term potentiation, and be- havioral deficits in social interaction and sensori- motor gating, suggesting that an impairment in NR1 phosphorylation leads to glutamatergic hypofunc- tion that can contribute to behavioral deficits asso- ciated with psychiatric disorders [163]. Glutathione S-transferase GST-M1, GST-T1, and GST-P1. Data from several studies suggest that oxidative stress may play a role in the pathophysiology of tardive dyskinesia (TD). Glutathione S-transferase (GST) en- zymes exert a protecting effect on cells against oxidative stress. The GST-M1, GST-T1, and GST-P1 loci were analyzed in SCZ patients with TD and without TD. There were no significant differences in the distributions of the GST-M1, GST-T1, and GST-P1 genotypes between the TD and non-TD groups. The Ile/Ile genotype of GST-P1 had higher AIMS score compared to Ile/Val + Val/Val genotype, and MDR analysis did not show a significant interaction between the three GST gene variants and susceptibility to TD. These results suggest that GST gene polymorphisms do not confer increased susceptibility to TD in patients with SCZ but TD severity might be re- lated with GST-P1 variants [164]. Glycogen synthase kinase-3 (GSK3). Adult neuro- genesis augments neuronal plasticity, and deficient neu- rogenesis might contribute to mood disorders and SCZ and impede treatment responses. These diseases might be associated with inadequately controlled glycogen syn- thase kinase-3 (GSK3). There is a drastic 40% impair- ment in neurogenesis in vivo in GSK3 alpha/beta (21A/ 21A/9A/9A) knockin mice compared with wildtype mice. Impaired neurogenesis could be due to effects of GSK3 in neural precursor cells (NPCs) or in surrounding cells that modulate NPCs. In vitro proliferation was equivalent for NPCs from GSK3 knockin and wild-type mice, sug- gesting an in vivo deficiency in GSK3 knockin mice of external support for NPC proliferation. Measurements of two neurotrophins that promote neurogenesis demon- strated less hippocampal vascular endothelial growth factor but not BDNF in GSK3 knockin mice than wild- type mice, reinforcing the possibility that insufficient environmental support in GSK3 knockin mice might contribute to impaired neurogenesis [165]. Accumulating evidence implicates deregulation of GSK3ss as a con- verging pathological event in AD and in neuropsychiatric disorders, including BD and SCZ. These neurological disorders share cognitive dysfunction as a hallmark. In rodents, increased phosphorylation of GSK3ss at serine-9 has been reported following cognitive training in two different hippocampus dependent cognitive tasks, i.e. inhibitory avoidance and novel object recognition task. Transgenic mice expressing the phosphorylation defec- tive mutant GSK3ss [S9A] show impaired memory in these tasks. GSK3ss [S9A] mice displayed impaired hippocampal L-LTP and facilitated LTD. Application of actinomycin, but not anisomycin, mimicked GSK3ss [S9A]-induced defects in L-LTP, suggesting that tran- scriptional activation is affected. This was further sup- ported by decreased expression of the immediate early gene c-Fos, a target gene of CREB. These data suggest a role for GSK3ss in long-term memory formation, by in- hibitory phosphorylation at serine-9 [166]. Golli-MBP. Multiple studies have reported oligoden- drocyte and myelin abnormalities, as well as dysregula- tion of their related genes, in brains of SCZ patients. One of these genes is the myelin-basic-protein (MBP) gene, which encodes two families of proteins: classic-MBPs and golli-MBPs. While the classic-MBPs are predomi- nantly located in the myelin sheaths of the nervous sys- tem, the golli proteins are more widely expressed and are found in both the immune and the nervous systems. As- sociation between six (out of 26 genotyped) SNPs has been found in Jewish Ashkenazi cohorts. Of these, three (rs12458282, rs2008323, rs721286) are from one linkage disequilibrium (LD) block which contains a CTCF bind- ing region. Haplotype analysis revealed significant “risk”/“protective” haplotypes for SCZ, suggesting that golli-MBP is a possible susceptibility gene for SCZ [167]. Growth factor signaling pathways. Evidence has accumulated that the activity of the signaling cascades of neuregulin-1, Wnt, TGF-beta, BDNF-p75 and DISC1 is different between control subjects and patients with SCZ. Copyright © 2013 SciRes. OPEN ACCESS
 R. Cacabelos et al. / Open Journal of Psychiatry 2 (2013) 46-139 92 These pathways are involved in embryonic and adult neurogenesis and neuronal maturation. Clinical data in- dicate that in SCZ the Wnt pathway is most likely hypo- active, whereas the Nrg1-ErbB4, the TGF-beta- and the BDNF-p75-pathways are hyperactive. Haplo-insuffiency of the DISC1 gene is currently the best-established SCZ risk factor. Preclinical experiments indicate that suppres- sion of DISC1 signaling leads to accelerated dendrite development in neuronal stem cells, accelerated migra- tion and aberrant integration into the neuronal network. Increasing NRG1-, BDNF- and TGF-beta signaling and decreasing Wnt signaling also promotes adult neuronal differentiation and migration. Deviations in these path- ways detected in SCZ might contribute to premature neuronal differentiation, accelerated migration and inap- propriate insertion into the neuronal network. Neuronal stem cells isolated from nasal biopsies from SCZ patients display signs of accelerated development, whilst in- creased erosion of telomeres and bone age provide fur- ther support for accelerated cell maturation in SCZ [168]. The role of fibroblast growth factor receptors (FGFR) in normal brain development has been well- documented in transgenic and knock-out mouse models. Changes in FGF and its receptors have also been ob- served in SCZ and related developmental disorders. A transgenic th(tk- )/th(tk-) mouse model with FGF receptor signaling disruption targeted to dopamine (DA) neurons, results in neurodevelopmental, anatomical, and bio- chemical alterations similar to those observed in human SCZ. In th(tk-)/th(tk-) mice hypoplastic development of DA systems induces serotonergic hyperinnervation of midbrain DA nuclei, demonstrating the co-developmen- tal relationship between DA and 5-HT systems. Behav- iorally, th(tk-)/th(tk-) mice displayed impaired sensory gaiting and reduced social interactions correctable by atypical antipsychotics and a specific 5-HT2A antagonist, M100907. The adult onset of neurochemical and behav- ioral deficits was consistent with the postpubertal time course of psychotic symptoms in SCZ and related disor- ders. The spectrum of abnormalities observed in th(tk-)/th(tk-) mice and the ability of atypical neurolep- tics to correct the behavioral deficits consistent with hu- man psychosis suggests that midbrain 5-HT2A-control- ling systems are important loci of therapeutic action [169]. Heat shock proteins (HSPA1B). Pae et al. [170] in- vestigated a group of SNPs of a set of genes coding for heat shock proteins (HSPA1A, HSPA1B and HSPA1L) and found a significant association between one HSPA1B variation and SCZ. An association between a set of variations (rs2227956, rs2075799, rs1043618, rs562047 and rs539689) within the same genes and a larger sample of schizophrenic inpatients was studied. A single varia- tion, rs539689 (HSPA1B), was found to be marginally associated with Positive and Negative Syndrome Scale (PANSS) positive scores at discharge, and haplotype analysis revealed a significant association between im- provement in PANSS scores with both A-C-G-G and A-C-G-G haplotypes. These findings support a role of heat shock proteins in the pathophysiology of SZ [170]. HOMER2. SNPs rs2306428 and rs17158184 of HOMER2 were significantly associated with SCZ in Irish samples. The protective allele at rs2306428 removes a predicted splice-enhancer binding site where HOMER2 is naturally truncated. No allelic effect of rs2306428 on neuropsychological function or on HOMER2 splicing was found [171]. IFNG (Interferon gamma). Dysregulation of the cy- tokine network in schizophrenia has been well docu- mented. Such changes may occur due to disturbances in cytokine levels that are linked to polymorphisms of cy- tokine genes. Paul-Samojedny et al. [172] performed the first study to examine the association between the IFN-γ gene polymorphism and psychopathological symptoms in Polish patients with paranoid SCZ. A SNP in the IFN-γ gene (+874T/A, rs 62559044) was found to be associated with paranoid SCZ in males, but not in females. The presence of allele A at position +874 in the IFN-γ gene correlates with 1.66-fold higher risk of paranoid SCZ development in males. 3’ Ig heavy chain locus enhancer HS1,2*A (IGHA1). Infectious and autoimmune pathogenic hypotheses of SCZ have been proposed, prompting searches for anti- bodies against viruses or brain structures, and for altered levels of immunoglobulins. Allele frequencies of the Ig heavy chain 3’ enhancer HS1,2*A are associated with several autoimmune diseases, suggesting a possible cor- relation between HS1,2 alleles and Ig production. Levels of serum Igs and HS1,2*A genotypes have been studied in SCZ. Serum concentrations of Ig classes and IgG sub- classes are higher in SCZ (80%) as compared to controls (68%). An increased frequency of the HS1,2*2A allele corresponded to increased Ig plasma levels, while an increased frequency of the HS1,2*1A allele corre- sponded to decreased Ig plasma levels. The transcription factor SP1 bound to the polymorphic region of both HS1,2*1A and HS1,2*2A while NF-kB bound only to the HS1,2*2A. Differences in transcription factor bind- ing sites in the two allelic variants of the 3’ IgH enhancer HS1,2 may provide a mechanism by which differences in Ig expression are affected [173]. Insulin-degrading enzyme (IDE). Insulin-degrading enzyme (IDE) is a neutral thiol metalloprotease, which cleaves insulin with high specificity. IDE also hydrolyzes Aβ, glucagon, IGF I and II, and beta-endorphin. The gene encoding IDE is located on chromosome 10q23-q25, a gene locus linked to SCZ. Insulin resistance with brain insulin receptor deficits/receptor dysfunction was re- Copyright © 2013 SciRes. OPEN ACCESS
 R. Cacabelos et al. / Open Journal of Psychiatry 2 (2013) 46-139 93 ported in SCZ. IDE cleaves IGF-I and IGF-II, which are implicated in the pathophysiology of SCZ, although po- lymorphic CA repeat in IGF1 did not show association with SCZ [174]. Brain gamma-endorphin levels, liber- ated from beta-endorphin exclusively by IDE, have been reported to be altered in SCZ. Studies on the expression of IDE protein in post-mortem brains of patients with SCZ and controls revealed a reduced number of IDE-ex- pressing neurons and IDE protein content in the left and right dorsolateral prefrontal cortex in SCZ compared with controls, but not in other brain areas. Haloperidol might exert some effect on IDE, through changes of the expression levels of its substrates IGF-I and II, insulin and beta-endorphin. Reduced cortical IDE expression might be part of the disturbed insulin signaling cascades found in SCZ and it might contribute to the altered me- tabolism of certain neuropeptides (IGF-I and IGF-II, beta-endorphin) in SCZ [175]. Interleukins. SCZ has been associated with abnor- malities in cytokines and cytokine receptors potentially linked to a defective immunological function in psy- chotic disorders. Some reports have shown that IL-3, colony stimulating factor 2 receptor alpha (CSF2RA) and IL-3 receptor alpha (IL3RA) are associated with SCZ. A significant association for IL3RA-rs6603272, but not for rs6645249, and a genotypic association of both polymorphisms with SCZ was found in a Chinese popu- lation. Haplotype TDT was statistically significant, with the rs6603272 (T) - rs6645249 (G) haplotype signifi- cantly associated with SCZ [176]. Interleukin-10 (IL-10), an important immunoregulatory cytokine, is located on chromosome 1q31-32, a region previously reported to be linked to SCZ in genetic studies. Poly- morphisms at positions −1082, −819 and −592 in the IL-10 promoter region were determined in Turkish SCZ patients, and significant differences were observed in both allelic and genotypic frequencies of the -592A/C polymorphism. GTA homozygotes (the high IL-10-pro- ducing haplotype) were more prevalent among schizo- phrenic patients than in controls, suggesting that the IL-10 gene promoter polymorphism may be one of the susceptibility factors to develop SCZ in the Turkish population [177]. ITIH3/4 (Inter-alpha-tryptin inhibitor, heavy chain 4), CACNA1C (Calcium channel, voltage-dependent, L type, alpha-1C subunit), and SDCCAG8 (Serologi- cally defined colon cancer antigen 8). The Schizophre- nia Psychiatric Genome-Wide Association Study Con- sortium (PGC) highlighted 81 single-nucleotide poly- morphisms (SNPs) with moderate evidence for associa- tion to schizophrenia. After follow-up in independent samples, seven loci attained genome-wide significance (GWS), but multi-locus tests suggested some SNPs that did not do so represented true associations. Hamshere et al. [47] found that variants at three loci (ITIH3/4, CACNA1C and SDCCAG8) are associated with SCZ. JARID2 (Jumonji (JMJ), at-rich interactive do- main 2). An association was found of D6S289, a dinu- cleotide repeat polymorphism in the JARID2 gene, with SCZ and was confirmed by individual genotyping after a genome screen with 400 microsatellite markers spaced at approximately 10 cM in two DNA pools consisting of 119 SCZ patients and 119 controls recruited from a ho- mogenous population in the Chang Le area of the Shan- dong peninsula of China. rs2235258 and rs9654600 in JARID2 showed association in allelic, genotypic and haplotypic tests with SCZ patients [178]. KCNH2 (Potassium voltage-gated channel, sub- family h (eag-related), member 2). Organized neuronal firing is crucial for cortical processing and this is dis- rupted in SCZ. A primate-specific isoform (3.1) of the ether-a-go-go-related K+ channel KCNH2 that modulates neuronal firing has been identified. KCNH2-3.1 messen- ger RNA levels are comparable to full-length KCNH2- 1A levels in brain but three orders of magnitude lower in heart. In hippocampus from individuals with SCZ, KCNH2-3.1 expression is 2.5-fold greater than KCNH2- 1A expression. A meta-analysis of five clinical data sets shows association of SNPs in KCNH2 with SCZ. Risk-associated alleles predict lower intelligence quo- tient scores and speed of cognitive processing, altered memory-linked functional magnetic resonance imaging signals and increased KCNH2-3.1 mRNA levels in post-mortem hippocampus. KCNH2-3.1 lacks a domain that is crucial for slow channel deactivation. Overexpres- sion of KCNH2-3.1 in primary cortical neurons induces a rapidly deactivating K+ current and a high-frequency, nonadapting firing pattern [179]. The potassium channels are thought to have a role in modulating electrical excitability in neurons, regulating calcium signaling in oligodendrocytes and regulating action potential duration in presynaptic terminals and GABA release. Shen et al. [180] chose three potassium channel genes, KCNH1, KCNJ10 and KCNN3, to inves- tigate the role of potassium channels in SCZ by geno- typing 23 SNPs (9 in KCNH1, 5 in KCNJ10 and 9 in KCNN3) in a Han Chinese sample consisting of 893 SCZ patients and 611 healthy controls. No significant difference in allelic or genotypic frequency was revealed between SCZ patients and healthy individuals. According to these results, it appears that the 23 SNPs within the three potassium genes examined by Shen et al. do not play a major role in SCZ in the Han Chinese population. Kynurenine pathway. Some studies of mRNA ex- pression, protein expression, and pathway metabolite levels have implicated dysregulation of the kynurenine pathway in the etiology of SCZ and BD. A SNP in each of six genes, TDO2 (tryptophan 2,3-dioxygenase), Copyright © 2013 SciRes. OPEN ACCESS
 R. Cacabelos et al. / Open Journal of Psychiatry 2 (2013) 46-139 94 HM74 (chemokine receptor HM74/ G protein-coupled receptor 109B; GRP109B), HM74A (G protein-cou- pled receptor 109A; GRP109A), MCHR1 (melanin- concentrating hormone receptor 1/G protein-coupled receptor 24; GPR24), MCHR2 (melanin-concentrat- ing hormone receptor 2), and MC5R (melanocortin 5 receptor), was tested for association with SCZ. An A allele in HM74 was significantly associated with SCZ and with SCZ plus BD combined. Augmentation of dis- ease risk was found for the complex genotype HM74[A, any] + MCHR1[T, any] + MCHR2[C, any] which con- ferred an OR maximal for the combined diagnostic cate- gory of SCZ plus BD, carried by 30% of the cases. TDO2[CC] + MC5R[G, any] + MCHR2[GC] conferred an OR maximal for SCZ alone, carried by 8% of SCZ cases. The combined risk posed by these related, com- plex genotypes is greater than any identified single locus and may derive from co-regulation of the kynurenine pathway by interacting genes, a lack of adequate mela- notropin-controlled sequestration of the kynurenine-de- rived pigments, or the production of melanotropin re- ceptor ligands through kynurenine metabolism [181]. MAGI2 (Membrane-associated guanylate kinase, WW and PDZ domains-containing, 2). MAGI2, a large gene (∼1.5 Mbps) that maps to chromosome 7q21, is involved in recruitment of neurotransmitter receptors such as AMPA- and NMDA-type glutamate receptors. Koide et al. [182] examined the relationship of SNP variations in MAGI2 and risk for SCZ in a large Japa- nese sample and explored the potential relationships be- tween variations in MAGI2 and aspects of human cogni- tive function related to glutamate activity. They found suggestive evidence for genetic association of common SNPs within MAGI2 locus and SCZ. Major histocompatibility complex (MHC). The In- ternational Schizophrenia Consortium [183] and the European SGENE-plus [184] found significant associa- tion with several markers spanning the major histocom- patibility complex (MHC) region on chromosome 6p21.3- 22.1. In the MHC region, the five genome-wide signifi- cant markers (MHC/HIST1H2BJ: rs6913660-C; MHC/ PRSS16: rs13219354-T; MHC/PRSS16: rs6932590-T; MHC/PGBD1: rs13211507-T; MHC/NOTCH4: rs3131296- G) have risk alleles with average control frequencies between 78% and 92%. The five chromosome 6p mark- ers, spanning about 5 Mb, cover 1.4 cM and exhibit sub- stantial linkage disequilibrium. rs3131296 shows corre- lation with classical HLA alleles (HLA-A*0101, HLA- B*0801, HLA-C*0701, HLA-DRB*0301, HLA-DQA* 0501, HLA-DQB*0201) [183,184]. This finding might give support to the infective-neuroimmune hypothesis of SCZ. Bergen et al. [48] reported a genome-wide signify- cant association in the MHC region (rs886424). Single- ton deletions were more frequent in both case groups compared with controls, whereas the largest CNVs (>500 kb) were significantly enriched only in SCZ cases. Two CNVs (16p11.2 duplications and 22q11 deletions) asso- ciated with SCZ were also overrepresented in the SCZ sample. Malic enzyme 2. Some studies have identified a puta- tive gene locus for both SCZ and BD in the chromosome 18q21 region. Microsatellite analyses showed evidence of association at two contiguous markers, both located at the same genetic distance and spanning approximately 11 known genes. In a corollary gene expression study, one of these genes, malic enzyme 2 (ME2), showed levels of gene expression 5.6-fold lower in anterior cingulate tis- sue from post-mortem bipolar brains. Subsequent analy- sis of individual SNPs in strong linkage disequilibrium with the ME2 gene revealed one SNP and one haplotype associated with the phenotype of psychosis. ME2 inter- acts directly with the malate shuttle system, which has been shown to be altered in SCZ and BD, and has roles in neuronal synthesis of glutamate and gamma-amino butyric acid. Genetic variation in or near the ME2 gene might be associated with both psychotic and manic dis- orders, including SCZ and BD [185]. Matrix metaloproteinases. Recent studies have de- monstrated that matrix metalloproteinase 3 (MMP3) is a key event in associative memory formation, learning and synaptic plasticity, which are important in psychiatric disorders. Genetic variations in the MMP3 -1171 5A/6A have been studied in patients with SCZ. The frequencies of the 6A6A genotype and 6A allele distributions of MMP3 in patients with SCZ were significantly decreased when compared with controls. In contrast, in patients with SCZ, the distribution of the 5A5A genotype and 5A allele were significantly increased as compared with healthy controls. When the frequencies of genotypes or alleles in schizophrenic patients and bipolar patients were compared, 6A6A genotype and 6A allele in patients with BD-I were significantly higher than in patients with SCZ. A potential link between MMP3-1171 5A/6A vari- ants and SCZ might be possible [186]. Matrix metallopeptidase 9 (gelatinase B, 92 kDa gelatinase, 92 kDa type IV collagenase) (MMP9). This gene plays a role in many pathological conditions such as cancer and heart disease and brain functions. It has been hypothesized that the MMP-9 gene may be associated with bipolar mood disorder. A functional −1562C/T polymorphism of the MMP-9 gene was genotyped. Pa- tients with bipolar mood disorder had significant pre- ponderance of T allele vs C allele of 1562C/T poly- morphism of the MMP-9 gene, compared to healthy con- trol subjects. The higher frequency of T allele com- pared to healthy subjects was especially evident in a subgroup of patients with BD, type II. The results may provide the first evidence for an involvement of the Copyright © 2013 SciRes. OPEN ACCESS
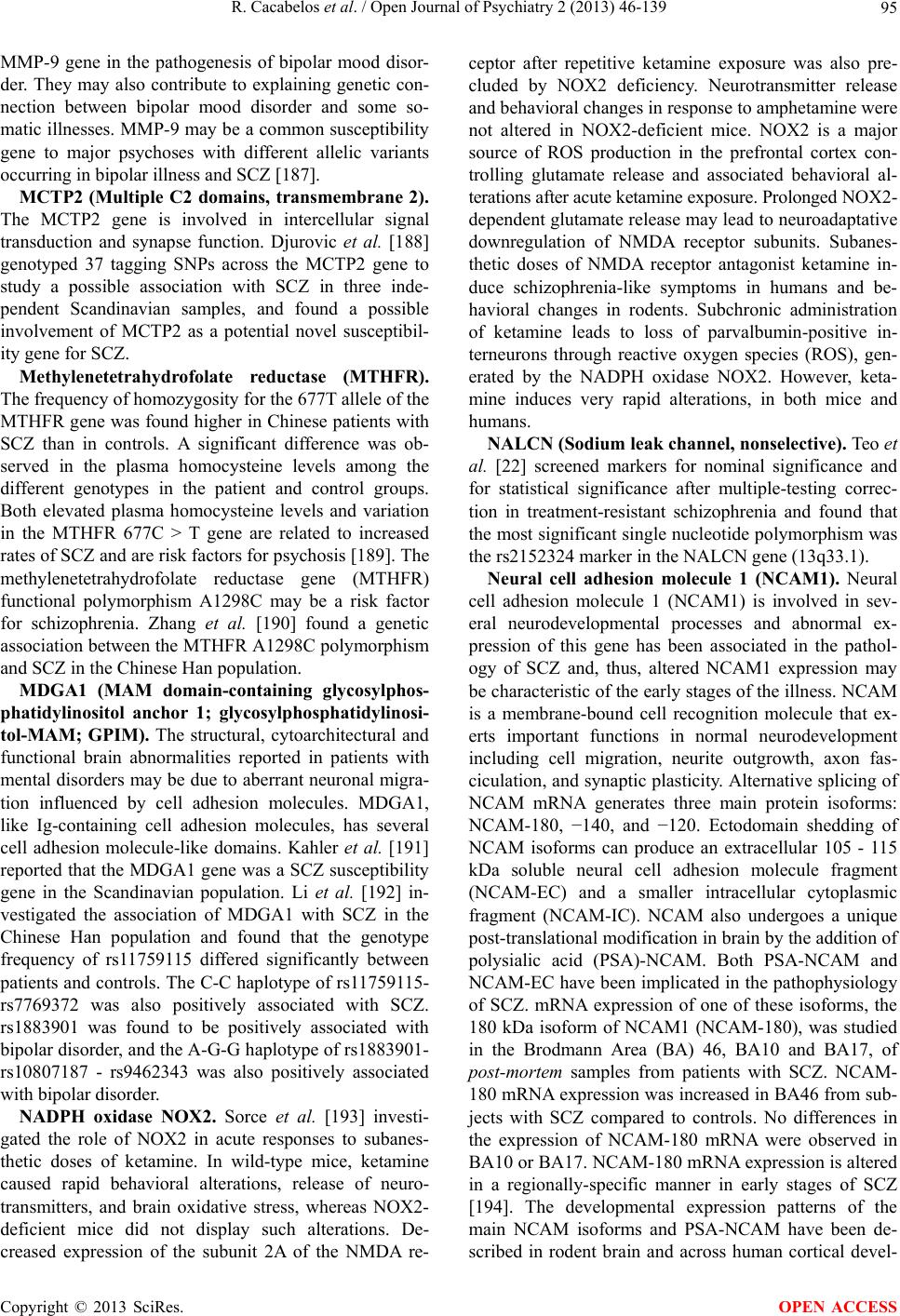 R. Cacabelos et al. / Open Journal of Psychiatry 2 (2013) 46-139 95 MMP-9 gene in the pathogenesis of bipolar mood disor- der. They may also contribute to explaining genetic con- nection between bipolar mood disorder and some so- matic illnesses. MMP-9 may be a common susceptibility gene to major psychoses with different allelic variants occurring in bipolar illness and SCZ [187]. MCTP2 (Multiple C2 domains, transmembrane 2). The MCTP2 gene is involved in intercellular signal transduction and synapse function. Djurovic et al. [188] genotyped 37 tagging SNPs across the MCTP2 gene to study a possible association with SCZ in three inde- pendent Scandinavian samples, and found a possible involvement of MCTP2 as a potential novel susceptibil- ity gene for SCZ. Methylenetetrahydrofolate reductase (MTHFR). The frequency of homozygosity for the 677T allele of the MTHFR gene was found higher in Chinese patients with SCZ than in controls. A significant difference was ob- served in the plasma homocysteine levels among the different genotypes in the patient and control groups. Both elevated plasma homocysteine levels and variation in the MTHFR 677C > T gene are related to increased rates of SCZ and are risk factors for psychosis [189]. The methylenetetrahydrofolate reductase gene (MTHFR) functional polymorphism A1298C may be a risk factor for schizophrenia. Zhang et al. [190] found a genetic association between the MTHFR A1298C polymorphism and SCZ in the Chinese Han population. MDGA1 (MAM domain-containing glycosylphos- phatidylinositol anchor 1; glycosylphosphatidylinosi- tol-MAM; GPIM). The structural, cytoarchitectural and functional brain abnormalities reported in patients with mental disorders may be due to aberrant neuronal migra- tion influenced by cell adhesion molecules. MDGA1, like Ig-containing cell adhesion molecules, has several cell adhesion molecule-like domains. Kahler et al. [191] reported that the MDGA1 gene was a SCZ susceptibility gene in the Scandinavian population. Li et al. [192] in- vestigated the association of MDGA1 with SCZ in the Chinese Han population and found that the genotype frequency of rs11759115 differed significantly between patients and controls. The C-C haplotype of rs11759115- rs7769372 was also positively associated with SCZ. rs1883901 was found to be positively associated with bipolar disorder, and the A-G-G haplotype of rs1883901- rs10807187 - rs9462343 was also positively associated with bipolar disorder. NADPH oxidase NOX2. Sorce et al. [193] investi- gated the role of NOX2 in acute responses to subanes- thetic doses of ketamine. In wild-type mice, ketamine caused rapid behavioral alterations, release of neuro- transmitters, and brain oxidative stress, whereas NOX2- deficient mice did not display such alterations. De- creased expression of the subunit 2A of the NMDA re- ceptor after repetitive ketamine exposure was also pre- cluded by NOX2 deficiency. Neurotransmitter release and behavioral changes in response to amphetamine were not altered in NOX2-deficient mice. NOX2 is a major source of ROS production in the prefrontal cortex con- trolling glutamate release and associated behavioral al- terations after acute ketamine exposure. Prolonged NOX2- dependent glutamate release may lead to neuroadaptative downregulation of NMDA receptor subunits. Subanes- thetic doses of NMDA receptor antagonist ketamine in- duce schizophrenia-like symptoms in humans and be- havioral changes in rodents. Subchronic administration of ketamine leads to loss of parvalbumin-positive in- terneurons through reactive oxygen species (ROS), gen- erated by the NADPH oxidase NOX2. However, keta- mine induces very rapid alterations, in both mice and humans. NALCN (Sodium leak channel, nonselective). Teo et al. [22] screened markers for nominal significance and for statistical significance after multiple-testing correc- tion in treatment-resistant schizophrenia and found that the most significant single nucleotide polymorphism was the rs2152324 marker in the NALCN gene (13q33.1). Neural cell adhesion molecule 1 (NCAM1). Neural cell adhesion molecule 1 (NCAM1) is involved in sev- eral neurodevelopmental processes and abnormal ex- pression of this gene has been associated in the pathol- ogy of SCZ and, thus, altered NCAM1 expression may be characteristic of the early stages of the illness. NCAM is a membrane-bound cell recognition molecule that ex- erts important functions in normal neurodevelopment including cell migration, neurite outgrowth, axon fas- ciculation, and synaptic plasticity. Alternative splicing of NCAM mRNA generates three main protein isoforms: NCAM-180, −140, and −120. Ectodomain shedding of NCAM isoforms can produce an extracellular 105 - 115 kDa soluble neural cell adhesion molecule fragment (NCAM-EC) and a smaller intracellular cytoplasmic fragment (NCAM-IC). NCAM also undergoes a unique post-translational modification in brain by the addition of polysialic acid (PSA)-NCAM. Both PSA-NCAM and NCAM-EC have been implicated in the pathophysiology of SCZ. mRNA expression of one of these isoforms, the 180 kDa isoform of NCAM1 (NCAM-180), was studied in the Brodmann Area (BA) 46, BA10 and BA17, of post-mortem samples from patients with SCZ. NCAM- 180 mRNA expression was increased in BA46 from sub- jects with SCZ compared to controls. No differences in the expression of NCAM-180 mRNA were observed in BA10 or BA17. NCAM-180 mRNA expression is altered in a regionally-specific manner in early stages of SCZ [194]. The developmental expression patterns of the main NCAM isoforms and PSA-NCAM have been de- scribed in rodent brain and across human cortical devel- Copyright © 2013 SciRes. OPEN ACCESS
 R. Cacabelos et al. / Open Journal of Psychiatry 2 (2013) 46-139 96 opment. Each NCAM isoform (NCAM-180, −140, and −120), post-translational modification (PSA-NCAM) and cleavage fragment (NCAM-EC and NCAM-IC) shows developmental regulation in frontal cortex. NCAM-180, −140, and −120, as well as PSA-NCAM, and NCAM-IC all showed strong developmental regulation during fetal and early postnatal ages, consistent with their identified roles in axon growth and plasticity. NCAM-EC demon- strated a more gradual increase from the early postnatal period to reach a plateau by early adolescence, potentially implicating involvement in later developmental processes. Altered NCAM signaling during specific developmental intervals might affect synaptic connectivity and circuit formation, and thereby contribute to neurodevelopmental disorders [195]. Neuregulin (NRG1, NRG3). Chromosome 8p12 has been identified as a locus for SCZ in several genome- wide scans and confirmed by meta-analysis of published linkage data. Systematic fine mapping using extended Icelandic pedigrees identified an associated haplotype in the gene neuregulin 1 (NRG1), also known as heuregulin, glial growth factor, NDF43 and ARIA. A 290 kb core at risk haplotype at the 5’ end of the gene (HAPICE), de- fined by five SNPs and two microsatellite polymor- phisms, was found to be associated with SCZ in the Ice- landic and Scottish populations. Analysis of the HAPICE markers showed the association of a 7-marker and 2-microsatellite haplotype, different from the haplotypes associated in the Icelandic population, and overrepre- sented in northern Swedish control individuals. Signifi- cant association was found with 5 SNPs located in the second intron of NRG1. Furthermore, 2-, 3-, and 4-SNP windows that comprise these SNPs were also associated. One protective haplotype (0% vs 1.8%) and 1 disease risk-causing haplotype (40.4% vs 34.9%) were defined [196]. Li et al. [197] analyzed data from the SNP mark- ers SNP8NRG241930, SNP8NRG243177, SNP8NRG- 221132 and SNP8NRG221533, and the microsatellite markers 478B14-848, 420M9-1395. Across these studies, a strong positive association was found for all six poly- morphisms. The haplotype analysis also showed signifi- cant association in the pooled international populations. In Asian populations, the risk haplotype was focused around the two microsatellite markers, 478B14-848, 420M9-1395 (haplotype block B), and in Caucasian populations with the remaining four SNP markers (hap- lotype block A). This meta-analysis supports the in- volvement of NRG1 in the pathogenesis of SCZ, but with association between two different but adjacent hap- lotype blocks in the Caucasian and Asian populations [197]. The promoter for the NRG1 isoform, SMDF, maps to the 3’ end of the gene complex. Analysis of the SNP data-base revealed several polymorphisms within the ap- proximate borders of the region immunoprecipitated in ChIP-chip experiments, one of which is rs7825588. This SNP was analyzed in patients with SCZ and BD and its effect on promoter function was assessed by electromo- bility gel shift assays and luciferase reporter constructs. A significant increase in homozygosity for the minor allele was found in patients with SCZ, but not in BD. Molecular studies demonstrated modest, but statistically significant, allele-specific differences in protein binding and promoter function. The findings suggest that homo- zygosity for rs725588 could be a risk genotype for SCZ [198]. Prata et al. [199] tested 4 SNPs, SNP8NRG221533 (rs35753505), SNP8NRG241930, SNP8NRG243177 (rs6994992) and SNPNRG222662 (rs4623364) for allelic and haplotypic association with BD and the presence of psychotic or mood-incongruent psychotic features. No- minal allele-wise significant association for SNP8NRG- 221533 was found, with the T allele being overrepresent- ed in SCZ. This is the opposite allelic association to the original association study where the C allele was associ- ated with SCZ. Subphenotypes were significantly associ- ated with the SNP8NRG221533(T)-SNP8NRG241930- (G) haplotype and with the SNP8NRG221533 (T)-SNP8- NRG222662-(C)-SNP8NRG241930(G) haplotype in the case of the broader subphenotype of psychotic bipolar. This study supports the hypothesis that NRG1 may play a role in the development of BD, especially in psychotic subtypes, albeit with different alleles to previous associa- tion reports in SCZ and BD [199]. NRG1 influences the development of white matter connectivity. The cingulum bundle is a white matter structure implicated in SCZ. Abnormalities in the structural integrity of the anterior cingulum in patients with SCZ have been reported. Frac- tional anisotropy is reduced in the anterior cingulum in SCZ. There is interaction between genetic variation in NRG1 and diagnosis of SCZ, and patients with the T allele for SNP8NRG221533: rs35753505 have decreased anterior cingulum fractional anisotropy compared with patients homozygous for the C allele and healthy con- trols who were T-carriers [200]. NRG1 is one of the most researched genes associated with SCZ. Although three meta-analyses in this area have been published, the re- sults have been inconclusive and even conflicting. Gong et al. [201] performed a meta-analysis of 26 published case-control and family-based association studies up to September 2008 covering 8049 cases, 8869 controls and 1515 families. Across these studies, the conclusions are as follows: 1) only SNP8NRG- 221132, 420M9-1395(0) and 478B14-848(0) showed significant association in the relatively small sample size; 2) the association analysis of case-control studies was statistically consistent with that of family studies; and 3) the matrix of coancestry coefficient suggested obvious population stratification. The study reveals that one SNP of the NRG1 gene does not contribute significantly to SCZ and that population Copyright © 2013 SciRes. OPEN ACCESS
 R. Cacabelos et al. / Open Journal of Psychiatry 2 (2013) 46-139 97 stratification is evident [201]. Quantitative real-time PCR was used to check the genotypes of four SNPs- rs221533 (C/T), rs7820838 (C/T), 433E1006 (A/G) and rs3924999 (C/T), located at the 510 terminus of the NRG1 gene, in 258 Chinese Han schizophrenic parent-proband trios. There was significant transmission disequilibrium in allelic transmission of C, A, T from rs221533, 433E1006, rs3924999 loci, respectively. Haplotype was analyzed at a frequency exceeding 1%. In three-marker-haplotype, C/C/G and C/C/A (rs221533, rs7820838, 433E1006) transmitted predominantly. In four-marker-haplotype (rs221533, rs7820838, 433E1006, rs3924999), C/C/G/T, C/C/A/C and C/C/A/T showed transmission disequilib- rium. According to these studies, the NRG1 gene poly- morphism is significantly associated with SCZ in Chi- nese Han, especially in the positive subtype of SCZ [202]. NRG1 is a pleiotropic growth factor involved in di- verse aspects of brain development and function. In SCZ, expression of the NRG1 type I isoform is selectively increased [203]. NRG1 and ERBB4 have emerged as some of the most reproducible SCZ risk genes. The Neuregulin (NRG)/ErbB4 signaling pathway has been implicated in dendritic spine morphogenesis, glutamater- gic synaptic plasticity, and neural network control. ErbB4-expressing interneurons, but not pyramidal neu- rons, are primary targets of NRG signaling in the hippo- campus and implicate ErbB4 as a selective marker for glutamatergic synapses on inhibitory interneurons [204]. Structural brain abnormalities are present at early phases of psychosis and might be the consequence of neurodevelopmental derailment. The SNP8NRG243177 risk T allele was significantly associated, in an allele copy number-dependent fashion, with increased lateral ventricle volume in psychosis. Genotype explained 7% of the variance of lateral ventricle volume. Genetic va- riations of the NRG1 gene can contribute to the enlar- gement of the lateral ventricles described in early phases of SCZ [205]. Linkage studies have implicated 10q22-q23 as a SCZ susceptibility locus in Ashkenazi Jewish (AJ) and Han Chinese from Taiwan populations. Chen et al. [206] per- formed a peakwide association fine mapping study by using 1414 SNPs across approximately 12.5 Mb in 10q22-q23 of Ashkenazi Jews, and found strong evi- dence of association by using the “delusion” factor as the quantitative trait at three SNPs (rs10883866, rs10748842, and rs6584400) located in a 13 kb interval in intron 1 of NRG3. NRG3 is primarily expressed in the CNS and is one of three paralogs of NRG1 [206]. Zhang et al. [207] genotyped 13 SNPs within NRG3 to investigate the as- sociation between NRG3 and schizophrenia in 488 pa- tients and 506 compared controls in Northwest China and no association was detected either in SNPs or in haplotypes. Neurogranin (NRGN). A marker located 3457 bases upstream of the neurogranin gene (NRGN) on 11q24 (rs128078009-T) has been associated with SCZ [208]. This marker has an average risk allele control frequency of 83%. Another NRGN SNP (rs7113041) has been re- ported to be associated with SCZ in Portuguese patients [209]. NRGN is expressed exclusively in brain under control of thyroid hormones, and is reduced in the pre- frontal cortex of patients with SCZ. NRGN encodes a postsynaptic protein kinase substrate that binds calmo- dulin in the absence of calcium. NRGN is abundant in dendritic spines of hippocampal CA1 pyramidal neurons, probably acting as a calmodulin reservoir. Altered NRGN activity might mediate the effects of NMDA hy- pofunction implicated in SCZ pathogenesis [208]. Neuropeptide Y. It has been suggested that hypoac- tivity of neuropeptide Y (NPY) may be involved in the pathophysiology of SCZ. A post-mo rtem study revealed a decreased level of NPY in the brain of patients with SCZ. An increased level of NPY after antipsychotic treatment was also reported in animal brain and cerebrospinal fluid of patients. A positive association between the functional −485C > T polymorphism in the NPY gene and SCZ was reported in the Japanese population; however, more re- cent studies suggest that the NPY −485C > T polymor- phism may not confer susceptibility to SCZ [210]. Neurotrophin receptor (NTRK-3). Based on the important role of neurotrophic factors in brain develop- ment and plasticity as well as their extensive expression in hippocampal areas, it has been hypothesized that a variation in the neurotrophin receptor 3 gene (NTRK-3) is associated with hippocampal function and SCZ. rs999905 was significantly associated with SCZ and the haplotype block that includes markers rs999905 and rs4887348 remained significant after permutation tests. The NTRK-3 gene influences hippocampal function and may modify the risk for SCZ [211]. Nitric oxide synthase (NOS). The neuronal nitric ox- ide synthase gene (NOS1) is located on 12q24.2-q24.31, in a susceptibility region for SCZ, and produces nitric oxide (NO) in the brain. NO plays a role in neurotrans- mitter release and is the second messenger of the N-methyl-D-aspartate (NMDA) receptor. It is also con- nected to the dopaminergic and serotonergic neural transmission systems. Therefore, abnormalities in the NO pathway are thought to be involved in the pathophysiol- ogy of SCZ. NOS1-G/G is associated with clinically sig- nificant variation in cognition. Whether this is a mecha- nism by which SCZ risk is increased is yet to be con- firmed [212]. Several genetic studies showed an associa- tion of NOS1 with SCZ; however, results of replication studies have been inconsistent. In a replication study of NOS1 with SCZ in a Japanese sample, Okumura et al. Copyright © 2013 SciRes. OPEN ACCESS
 R. Cacabelos et al. / Open Journal of Psychiatry 2 (2013) 46-139 98 [213] selected seven SNPs (rs41279104, rs3782221, rs3782219, rs561712, rs3782206, rs2682826, and rs6490121) in NOS1 that were positively associated with SCZ in previous studies. Two SNPs showed an associa- tion with Japanese schizophrenic patients (rs3782219, rs3782206); however, these associations might have re- sulted from type I error on account of multiple testing [213]. In other studies no evidence was found of associa- tion with rs6490121 in NOS1 [214]. Association between markers within a region on chromosome 1q23.3, including the NOS1AP (nitric ox- ide synthese 1 (neuronal) adaptor protein) gene and SCZ has been found in a set of Canadian families of European descent, as well as significantly increased ex- pression in SCZ of NOS1AP in unrelated post-mortem samples from the dorsolateral prefrontal cortex. Using the posterior probability of linkage disequilibrium (PPLD) to measure the probability that a SNP is in link- age disequilibrium with SCZ, Wratten et al. [215] evalu- ated 60 SNPs from NOS1AP in 24 Canadian families demonstrating linkage and association to this region. Two human neural cell lines (SK-N-MC and PFSK-1) were transfected with a vector containing each allelic variant of the NOS1AP promoter and a luciferase gene. Three SNPs produced PPLDs > 40%. One of them, rs12742393, demonstrated significant allelic expression differences in both cell lines tested. The allelic variation at this SNP altered the affinity of nuclear protein binding to this region of DNA, indicating that the A allele of rs12742393 appears to be a risk allele associated with SCZ that acts by enhancing transcription factor binding and increasing gene expression [215]. Findings of an- other study in the South American population are also consistent with a role for NOS1AP in susceptibility to SCZ, especially for the “negative syndrome” of the dis- order [216]. NKCC1 and KCC2 (SLC12A2; SLC12A5). The na- ture of γ-aminobutyric acid neurotransmission depends on the local intracellular chloride concentration. In the CNS, the intracellular chloride level is determined by the activity of 2 cation-chloride transporters, NKCC1 and KCC2. The activities of these transporters are in turn regulated by a network of serine-threonine kinases that includes OXSR1, STK39, and the WNK kinases WNK1, WNK3, and WNK4. Arion and Lewis [217] compared the levels of NKCC1, KCC2, OXSR1, STK39, WNK1, WNK3, and WNK4 transcripts in prefrontal cortex area 9 between subjects with SCZ and healthy subjects. OXSR1 and WNK3 transcripts were substantially overexpressed in subjects with schizophrenia relative to comparison subjects. In contrast, NKCC1, KCC2, STK39, WNK1, and WNK4 transcript levels did not differ between sub- ject groups. OXSR1 and WNK3 transcript expression levels were not changed in antipsychotic-exposed mon- keys and were not affected by potential confounding factors in the subjects with schizophrenia. According to Arion and Lewis, in schizophrenia, increased expression levels, and possibly increased kinase activities, of OXSR1 and WNK3 may shift the balance of chloride transport by NKCC1 and KCC2 and alter the nature of γ-aminobutyric acid neurotransmission in the prefrontal cortex. NOGO-66 receptor 1 (RTN4R). SCZ or schizoaffec- tive disorders are quite common features in patients with DiGeorge/velo-cardio-facial syndrome (DGS/VCFS) as a result of chromosome 22q11.21 haploinsufficiency. In SCZ, genetic predisposition has been linked to chromo- some 22q11, and myelin-specific genes are misexpressed in SCZ. Nogo-66 receptor 1 (NGR or RTN4R) has been considered to be a 22q11 candidate gene for SCZ suscep- tibility because it encodes an axonal protein that medi- ates myelin inhibition of axonal sprouting. The RTN4R gene encodes a subunit of the receptor complex (RTN4R- p75NTR) which results in neuronal growth inhibitory signals in response to Nogo-66, MAG or OMG signaling. RTN4R regulates axonal growth, as well as axon regen- eration after injury. The gene maps to the 22q11.21 SCZ susceptibility locus and is thus a strong functional and positional candidate gene. RTN4R encodes for a func- tional cell surface receptor, a glycosyl-phosphatidy- linositol (GPI)-linked protein, with multiple leucine-rich repeats (LRR), which is implicated in axonal growth inhibition. Three mutant alleles were detected, including two missense changes (c.355C > T; R119W and c.587G > A; R196H), and one synonymous codon variant (c.54G > A; L18L). Schizophrenic patients with the missense changes were strongly resistant to the neuroleptic treat- ment at any dosage. Both missense changes were absent in 300 control subjects. Molecular modeling revealed that both changes lead to putative structural alterations of the native protein [218]. RTN4R deficiency can modu- late the long-term behavioral effects of transient postna- tal N-methyl-D-aspartate (NMDA) receptor hypofunc- tion. Results reported by Meng et al. [219] and Hsu et al. [220] do not support a major role of RTN4R in suscepti- bility to SCZ or the cognitive and behavioral deficits observed in individuals with 22q11 microdeletions; however, they suggest that RTN4R may modulate the genetic risk or clinical expression of SCZ in a subset of patients. Neuronal cultures demonstrate that four different SCZ- derived NgR1 variants fail to transduce myelin signals into axon inhibition, and function as dominant negatives to disrupt endogenous NgR1. Mice lacking NgR1 protein exhibit reduced working memory function, consistent with a potential endophenotype of SCZ. For a restricted subset of individuals diagnosed with SCZ, the expression of dysfunctional NGR variants may contribute to in- Copyright © 2013 SciRes. OPEN ACCESS
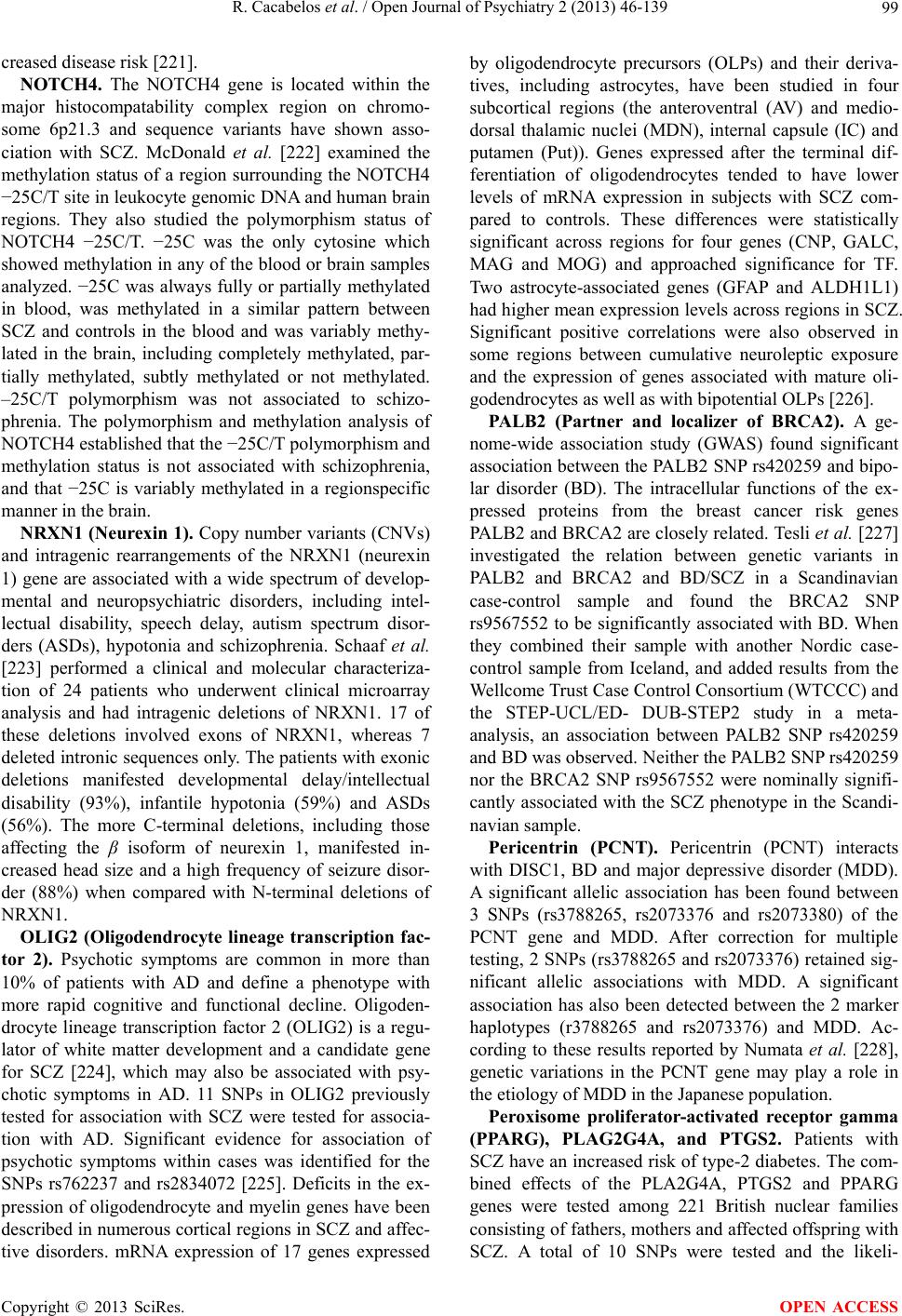 R. Cacabelos et al. / Open Journal of Psychiatry 2 (2013) 46-139 99 creased disease risk [221]. NOTCH4. The NOTCH4 gene is located within the major histocompatability complex region on chromo- some 6p21.3 and sequence variants have shown asso- ciation with SCZ. McDonald et al. [222] examined the methylation status of a region surrounding the NOTCH4 −25C/T site in leukocyte genomic DNA and human brain regions. They also studied the polymorphism status of NOTCH4 −25C/T. −25C was the only cytosine which showed methylation in any of the blood or brain samples analyzed. −25C was always fully or partially methylated in blood, was methylated in a similar pattern between SCZ and controls in the blood and was variably methy- lated in the brain, including completely methylated, par- tially methylated, subtly methylated or not methylated. –25C/T polymorphism was not associated to schizo- phrenia. The polymorphism and methylation analysis of NOTCH4 established that the −25C/T polymorphism and methylation status is not associated with schizophrenia, and that −25C is variably methylated in a regionspecific manner in the brain. NRXN1 (Neurexin 1). Copy number variants (CNVs) and intragenic rearrangements of the NRXN1 (neurexin 1) gene are associated with a wide spectrum of develop- mental and neuropsychiatric disorders, including intel- lectual disability, speech delay, autism spectrum disor- ders (ASDs), hypotonia and schizophrenia. Schaaf et al. [223] performed a clinical and molecular characteriza- tion of 24 patients who underwent clinical microarray analysis and had intragenic deletions of NRXN1. 17 of these deletions involved exons of NRXN1, whereas 7 deleted intronic sequences only. The patients with exonic deletions manifested developmental delay/intellectual disability (93%), infantile hypotonia (59%) and ASDs (56%). The more C-terminal deletions, including those affecting the β isoform of neurexin 1, manifested in- creased head size and a high frequency of seizure disor- der (88%) when compared with N-terminal deletions of NRXN1. OLIG2 (Oligodendrocyte lineage transcription fac- tor 2). Psychotic symptoms are common in more than 10% of patients with AD and define a phenotype with more rapid cognitive and functional decline. Oligoden- drocyte lineage transcription factor 2 (OLIG2) is a regu- lator of white matter development and a candidate gene for SCZ [224], which may also be associated with psy- chotic symptoms in AD. 11 SNPs in OLIG2 previously tested for association with SCZ were tested for associa- tion with AD. Significant evidence for association of psychotic symptoms within cases was identified for the SNPs rs762237 and rs2834072 [225]. Deficits in the ex- pression of oligodendrocyte and myelin genes have been described in numerous cortical regions in SCZ and affec- tive disorders. mRNA expression of 17 genes expressed by oligodendrocyte precursors (OLPs) and their deriva- tives, including astrocytes, have been studied in four subcortical regions (the anteroventral (AV) and medio- dorsal thalamic nuclei (MDN), internal capsule (IC) and putamen (Put)). Genes expressed after the terminal dif- ferentiation of oligodendrocytes tended to have lower levels of mRNA expression in subjects with SCZ com- pared to controls. These differences were statistically significant across regions for four genes (CNP, GALC, MAG and MOG) and approached significance for TF. Two astrocyte-associated genes (GFAP and ALDH1L1) had higher mean expression levels across regions in SCZ. Significant positive correlations were also observed in some regions between cumulative neuroleptic exposure and the expression of genes associated with mature oli- godendrocytes as well as with bipotential OLPs [226]. PALB2 (Partner and localizer of BRCA2). A ge- nome-wide association study (GWAS) found significant association between the PALB2 SNP rs420259 and bipo- lar disorder (BD). The intracellular functions of the ex- pressed proteins from the breast cancer risk genes PALB2 and BRCA2 are closely related. Tesli et al. [227] investigated the relation between genetic variants in PALB2 and BRCA2 and BD/SCZ in a Scandinavian case-control sample and found the BRCA2 SNP rs9567552 to be significantly associated with BD. When they combined their sample with another Nordic case- control sample from Iceland, and added results from the Wellcome Trust Case Control Consortium (WTCCC) and the STEP-UCL/ED- DUB-STEP2 study in a meta- analysis, an association between PALB2 SNP rs420259 and BD was observed. Neither the PALB2 SNP rs420259 nor the BRCA2 SNP rs9567552 were nominally signifi- cantly associated with the SCZ phenotype in the Scandi- navian sample. Pericentrin (PCNT). Pericentrin (PCNT) interacts with DISC1, BD and major depressive disorder (MDD). A significant allelic association has been found between 3 SNPs (rs3788265, rs2073376 and rs2073380) of the PCNT gene and MDD. After correction for multiple testing, 2 SNPs (rs3788265 and rs2073376) retained sig- nificant allelic associations with MDD. A significant association has also been detected between the 2 marker haplotypes (r3788265 and rs2073376) and MDD. Ac- cording to these results reported by Numata et al. [228], genetic variations in the PCNT gene may play a role in the etiology of MDD in the Japanese population. Peroxisome proliferator-activated receptor gamma (PPARG), PLAG2G4A, and PTGS2. Patients with SCZ have an increased risk of type-2 diabetes. The com- bined effects of the PLA2G4A, PTGS2 and PPARG genes were tested among 221 British nuclear families consisting of fathers, mothers and affected offspring with SCZ. A total of 10 SNPs were tested and the likeli- Copyright © 2013 SciRes. OPEN ACCESS
 R. Cacabelos et al. / Open Journal of Psychiatry 2 (2013) 46-139 100 hood-based association analysis for nuclear families was used to analyze the genotyping data. Eight SNPs detected across the PPARG gene did not show allelic association with SCZ; a weak association was detected at rs2745557 in the PTGS2 locus and rs10798059 in the PLA2G4A locus. The gene-gene interaction test did not show any evidence of either cis-phase interactions for the PLA2G4A and PTGS2 combinations or a trans-phase interaction for the PLA2G4A and PPARG combinations. The PPARG gene has been reported to be strongly asso- ciated with type-2 diabetes, but the results reported by Mathur et al. [229] did not support the hypothesis that the PPARG gene may play a role in the development of SCZ. Phosphatidylinositol 4-kinase type-II beta (PI4K2B). Linkage of BD and recurrent major depression with markers on chromosome 4p15.2 has been identified in a large Scottish family and three smaller families. Analysis of haplotypes in the four chromosome 4p-linked families, identified two regions, each shared by three of the four families, which are also supported by a case-control as- sociation study. The candidate gene phosphatidylinositol 4-kinase type-II beta (PI4K2B) lies within one of these regions. PI4K2B is a strong functional candidate as it is a member of the phosphatidylinositol pathway, which is targeted by lithium for therapeutic effect in BD. A case- control association study, using tagging SNPs from the PI4K2B genomic region, in BD, SCZ and controls showed association with a two-marker haplotype in SCZ but not in BD (rs10939038 and rs17408391). Expression studies at the allele-specific mRNA and protein level using lymphoblastoid cell lines from members of the large Scottish family, which showed linkage to 4p15.2 in BD and recurrent major depression, showed no differ- ence in expression differences between affected and non-affected family members. There is no evidence to suggest that PI4K2B is contributing to BD in this family but a role for this gene in SCZ has not been excluded [230]. Presenilin 2 (PSEN2). Mutations in the presenilin 1 (PSEN1) and PSEN2 genes are major causative factors for AD [35]. Presenilins are a group of proteins playing an important role in the Notch, ErbB4 and Wnt signaling pathways which might also be associated with SCZ and/or psychotic symptoms in dementia. The gene cod- ing for presenilin 2 (PSEN2) is located on 1q31-q42 and adjacent to a balanced translocation t (1; 11) (q42; q14.3) that was found to co-segregate within family members of patients with SCZ. Five functional SNPs (rs1295645, rs11405, rs6759, rs1046240 and rs8383) present in the coding regions of the PSEN2 gene were tested in 410 patients with SCZ and 355 controls in a Chinese Han population. Association analysis showed a weak associa- tion for rs1295645, and the rs1295645-T allele was in- volved in increased risk of SCZ. The T-C-T-T-T haplo- type also showed association with increased risk of SCZ. Analysis of gene expression demonstrated that PSEN2 mRNA levels in peripheral leukocytes were significantly lower in SCZ than in controls and that expression levels of the PSEN2 gene were significantly correlated to its genotypes. Analysis of clinical profiles showed an asso- ciation between the PSEN2 gene and some clinical phe- notypes scored using the PANSS. The PSEN2 gene may be a novel candidate involved in the development of cer- tain psychotic symptoms in SCZ [231]. Proline dehydrogenase/proline oxidase (PRODH). Significant associations have been shown for haplotypes comprising three PRODH SNPs: 1945T/C, 1766A/G, and 1852G/A, located in the 3’ region of the gene, suggesting a role of these variants in the pathogenesis of SCZ. Stud- ies on prepulse inhibition (PPI), verbal and working memory, trait anxiety and schizotypy indicate that CGA carriers exhibit attenuated PPI and verbal memory to- gether with higher anxiety and schizotypy compared with noncarriers [232]. Quaking homolog, KH doma in RNA binding (QKI). Chromosome 6q26 includes a susceptibility locus for SCZ in a large pedigree from northern Sweden. Aberg et al. [233] fine-mapped a 10.7 Mb region, included in this locus, using 42 microsatellites or SNP markers, and found a 0.5 Mb haplotype, within the large family that is shared among the majority of the patients (69%). A gam- ete competition test of this haplotype in 176 unrelated nuclear families from the same geographical area as the large family showed association to SCZ. The only gene located in the region, the quaking homolog, KH domain RNA binding (mouse) (QKI), was investigated in human brain autopsies. Relative mRNA expression levels of two QKI splice variants were clearly downregulated in schizophrenic patients. The mouse homolog is involved in neural development and myelination [233]. Disturbed QKI mRNA expression is observed in the prefrontal cor- tex of patients, and some of these changes correlate to treatment with antipsychotic drugs. In human astrocy- toma (U343) and oligodendroglioma (HOG) cell lines treated with five different antipsychotic drugs including haloperidol, aripiprazole, clozapine, olanzapine and risperidone, haloperidol treatment doubled QKI-7 mRNA levels in U343 cells after 6 hours. The effect was dose-dependent, and cells treated with ten times higher concentration responded with a five-fold and three-fold increase in QKI-7, 6 and 24 hours after treatment, re- spectively. QKI-7 mRNA expression in human astrocytes is induced by haloperidol, at concentrations similar to plasma levels relevant to clinical treatment of SCZ [234]. RANBP1 (RAN-binding protein 1). Smooth pursuit eye movement (SPEM) disturbance is proposed as one of the most consistent neurophysiological endophenotypes Copyright © 2013 SciRes. OPEN ACCESS
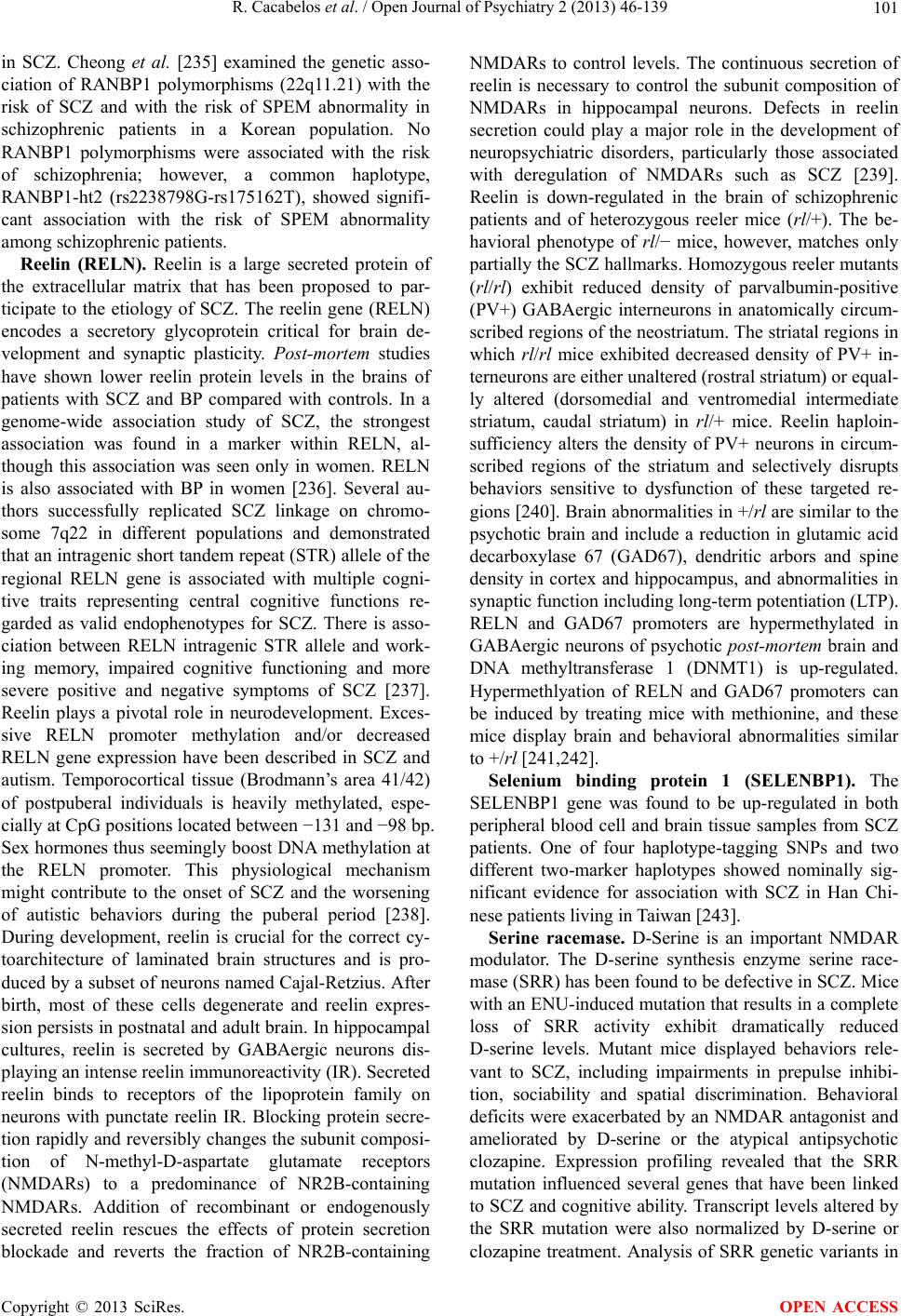 R. Cacabelos et al. / Open Journal of Psychiatry 2 (2013) 46-139 101 in SCZ. Cheong et al. [235] examined the genetic asso- ciation of RANBP1 polymorphisms (22q11.21) with the risk of SCZ and with the risk of SPEM abnormality in schizophrenic patients in a Korean population. No RANBP1 polymorphisms were associated with the risk of schizophrenia; however, a common haplotype, RANBP1-ht2 (rs2238798G-rs175162T), showed signifi- cant association with the risk of SPEM abnormality among schizophrenic patients. Reelin (RELN). Reelin is a large secreted protein of the extracellular matrix that has been proposed to par- ticipate to the etiology of SCZ. The reelin gene (RELN) encodes a secretory glycoprotein critical for brain de- velopment and synaptic plasticity. Post-mortem studies have shown lower reelin protein levels in the brains of patients with SCZ and BP compared with controls. In a genome-wide association study of SCZ, the strongest association was found in a marker within RELN, al- though this association was seen only in women. RELN is also associated with BP in women [236]. Several au- thors successfully replicated SCZ linkage on chromo- some 7q22 in different populations and demonstrated that an intragenic short tandem repeat (STR) allele of the regional RELN gene is associated with multiple cogni- tive traits representing central cognitive functions re- garded as valid endophenotypes for SCZ. There is asso- ciation between RELN intragenic STR allele and work- ing memory, impaired cognitive functioning and more severe positive and negative symptoms of SCZ [237]. Reelin plays a pivotal role in neurodevelopment. Exces- sive RELN promoter methylation and/or decreased RELN gene expression have been described in SCZ and autism. Temporocortical tissue (Brodmann’s area 41/42) of postpuberal individuals is heavily methylated, espe- cially at CpG positions located between −131 and −98 bp. Sex hormones thus seemingly boost DNA methylation at the RELN promoter. This physiological mechanism might contribute to the onset of SCZ and the worsening of autistic behaviors during the puberal period [238]. During development, reelin is crucial for the correct cy- toarchitecture of laminated brain structures and is pro- duced by a subset of neurons named Cajal-Retzius. After birth, most of these cells degenerate and reelin expres- sion persists in postnatal and adult brain. In hippocampal cultures, reelin is secreted by GABAergic neurons dis- playing an intense reelin immunoreactivity (IR). Secreted reelin binds to receptors of the lipoprotein family on neurons with punctate reelin IR. Blocking protein secre- tion rapidly and reversibly changes the subunit composi- tion of N-methyl-D-aspartate glutamate receptors (NMDARs) to a predominance of NR2B-containing NMDARs. Addition of recombinant or endogenously secreted reelin rescues the effects of protein secretion blockade and reverts the fraction of NR2B-containing NMDARs to control levels. The continuous secretion of reelin is necessary to control the subunit composition of NMDARs in hippocampal neurons. Defects in reelin secretion could play a major role in the development of neuropsychiatric disorders, particularly those associated with deregulation of NMDARs such as SCZ [239]. Reelin is down-regulated in the brain of schizophrenic patients and of heterozygous reeler mice (rl/+). The be- havioral phenotype of rl/− mice, however, matches only partially the SCZ hallmarks. Homozygous reeler mutants (rl/rl) exhibit reduced density of parvalbumin-positive (PV+) GABAergic interneurons in anatomically circum- scribed regions of the neostriatum. The striatal regions in which rl/rl mice exhibited decreased density of PV+ in- terneurons are either unaltered (rostral striatum) or equal- ly altered (dorsomedial and ventromedial intermediate striatum, caudal striatum) in rl/+ mice. Reelin haploin- sufficiency alters the density of PV+ neurons in circum- scribed regions of the striatum and selectively disrupts behaviors sensitive to dysfunction of these targeted re- gions [240]. Brain abnormalities in +/rl are similar to the psychotic brain and include a reduction in glutamic acid decarboxylase 67 (GAD67), dendritic arbors and spine density in cortex and hippocampus, and abnormalities in synaptic function including long-term potentiation (LTP). RELN and GAD67 promoters are hypermethylated in GABAergic neurons of psychotic post-mortem brain and DNA methyltransferase 1 (DNMT1) is up-regulated. Hypermethlyation of RELN and GAD67 promoters can be induced by treating mice with methionine, and these mice display brain and behavioral abnormalities similar to +/rl [241,242]. Selenium binding protein 1 (SELENBP1). The SELENBP1 gene was found to be up-regulated in both peripheral blood cell and brain tissue samples from SCZ patients. One of four haplotype-tagging SNPs and two different two-marker haplotypes showed nominally sig- nificant evidence for association with SCZ in Han Chi- nese patients living in Taiwan [243]. Serine racemase. D-Serine is an important NMDAR modulator. The D-serine synthesis enzyme serine race- mase (SRR) has been found to be defective in SCZ. Mice with an ENU-induced mutation that results in a complete loss of SRR activity exhibit dramatically reduced D-serine levels. Mutant mice displayed behaviors rele- vant to SCZ, including impairments in prepulse inhibi- tion, sociability and spatial discrimination. Behavioral deficits were exacerbated by an NMDAR antagonist and ameliorated by D-serine or the atypical antipsychotic clozapine. Expression profiling revealed that the SRR mutation influenced several genes that have been linked to SCZ and cognitive ability. Transcript levels altered by the SRR mutation were also normalized by D-serine or clozapine treatment. Analysis of SRR genetic variants in Copyright © 2013 SciRes. OPEN ACCESS
 R. Cacabelos et al. / Open Journal of Psychiatry 2 (2013) 46-139 102 humans identified a potential association with SCZ. Ab- errant Srr function and diminished D-serine may con- tribute to SCZ pathogenesis [244]. Serotonin 6 (5-HT6) receptors (HTR6). Genetic al- terations in serotonin 6 (5-HT6) receptors might be asso- ciated with the pathophysiology of SCZ. Kishi et al. [245] conducted an association study of the HTR6 gene (rs1805054 (C267T)) in Japanese patients and found no significant associations between the tagging SNPs in HTR6 and SCZ. In a meta-analysis of rs1805054, draw- ing data from five studies, rs1805054 was not associated with SCZ. Serotonin (5-Hydroxytryptamine (5-HT)) trans- porter (SLC6A4). Serotonin (5-hydroxytryptamine (5-HT)) transporter (SLC6A4) is known to influence mood, emotion, cognition and efficacy of antidepressants, particularly that of selective serotonin reuptake inhibitors. Atypical antipsychotics exert their effects partially through serotonergic systems, and hence, variation in 5-HT uptake may affect antipsychotic action mediated through the serotonergic system. The genetic roles of five polymorphisms of SLC6A4, including those of the widely studied 44 base pair variable number of tandem repeat (VNTR) in the promoter region of SLC6A4 (the serotonin transporter gene-linked polymorphic region: 5HTTLPR) and a VNTR polymorphism (STin2) in the second intron, have been studied in SCZ. Significant allelic and genotypic associations with rs2066713, 5HTTLPR and STin2 polymorphisms have been detected. A haplotype linking these three risk alleles, 5HTTLPR/ S-rs2066713/C-STin2/12-repeat, was also significantly associated with SCZ in a South Indian population [246]. A common polymorphism STin2 VNTR in the 5-HTT gene has been extensively investigated in genetic asso- ciation studies. Lin et al. [247] conducted a case-control study of the association between STin2 VNTR and three tagging SNPs in 5-HTT and SCZ in the Han Chinese population and no association was found in the single locus, but haplotype-based analyses revealed significant association between two haplotypes with SCZ [247]. A 44 base pair insertion (“l”)/deletion (“s”) polymorphism (called 5-HTTLPR) in the 5’ promoter region of the hu- man serotonin transporter gene modulates expression and has been associated to anxiety and depressive traits in otherwise healthy individuals. In individuals with SCZ it seems to modulate symptom severity, acting as a disease modifying gene. In dominant models, the 5-HTTLPR genotype accounted for a significant portion of the vari- ance in SCID depression and SANS negative symptoms (about 5%). The l allele was associated with greater psy- chopathology and the s allele was associated with greater anxiety and depression levels. Allelic variation may have different consequences for personality traits or psychiat- ric symptoms depending on epistasis or epigenetic con- text [248]. The serotonin transporter-linked polymorphic region (5-HTTLPR) short allele confers a general sensi- tivity to environmental stimuli, and anger is suspected to have a direct influence on aggressive behavior in SCZ. The 5-HTTLPR gene was associated with aggression and/or anger-related traits in SCZ; however, there was no significant difference in the distribution of the 5- HTTLPR genotype/alleles between the aggressive and nonaggressive patients. Aggressive patients carrying the s allele exhibited more anger-related traits than those with the l/l homozygotes, but this difference was not significant after correction for multiple testing. 5- HTTLPR predisposes aggressive patients to exhibit more anger-related traits, but there is no association between 5-HTTLPR and aggressive behavior in SCZ [249]. SH2B1 (SH2B adaptor protein 1). The short arm of chromosome 16 is rich in segmental duplications, pre- disposing this region of the genome to a number of re- current rearrangements. Genomic imbalances of an ap- proximately 600-kb region in 16p11.2 (29.5 - 30.1 Mb) have been associated with autism, intellectual disability, congenital anomalies, and SCZ. A separate, distal 200-kb region in 16p11.2 (28.7 - 28.9 Mb) that includes the SH2B1 gene has been associated with isolated obesity. Bachmann-Gagescu et al. [250] studied the phenotype of this recurrent SH2B1-containing microdeletion in a co- hort of phenotypically abnormal patients with develop- mental delay. Deletions of the SH2B1-containing region were identified in 31 patients. The deletion is enriched in the patient population when compared with controls, with both inherited and de novo events. Body mass index was ≥95th percentile in four of six patients, supporting the previously described association with obesity. Ac- cordingly, it appears that deletions of the 16p11.2 SH2B1- containing region are pathogenic and are associated with developmental delay in addition to obesity. SHMT1 (Serine hydroxymethyltransferase, cytoso- lic). NMDA receptor function affects PPI integrity and D-serine and glycine are endogenous co-agonists for the receptor. A quantitative trait loci analysis using C57BL/6 (B6) mice with better PPI performance and C3H/He (C3) with lower PPI score has been reported. Genes for both D-serine synthesizing enzyme and enzyme for reversible conversion between glycine and L-serine (SRR and SHMT1, respectively) are located in the same PPI-quan- titative trait loci peak. Maekawa et al. [251] analyzed expression levels and genetic polymorphisms of the two genes. There were promoter polymorphisms in SHMT1, which elicit lower transcriptional activity in B6 com- pared to C3 conforming to the results of brain expression levels, but no functional genetic variants in SRR. SHMT1 levels were higher in schizophrenic brains compared to controls, with no changes in SRR levels. A nominal as- sociation between SHMT1 and SCZ has been suggested. Copyright © 2013 SciRes. OPEN ACCESS
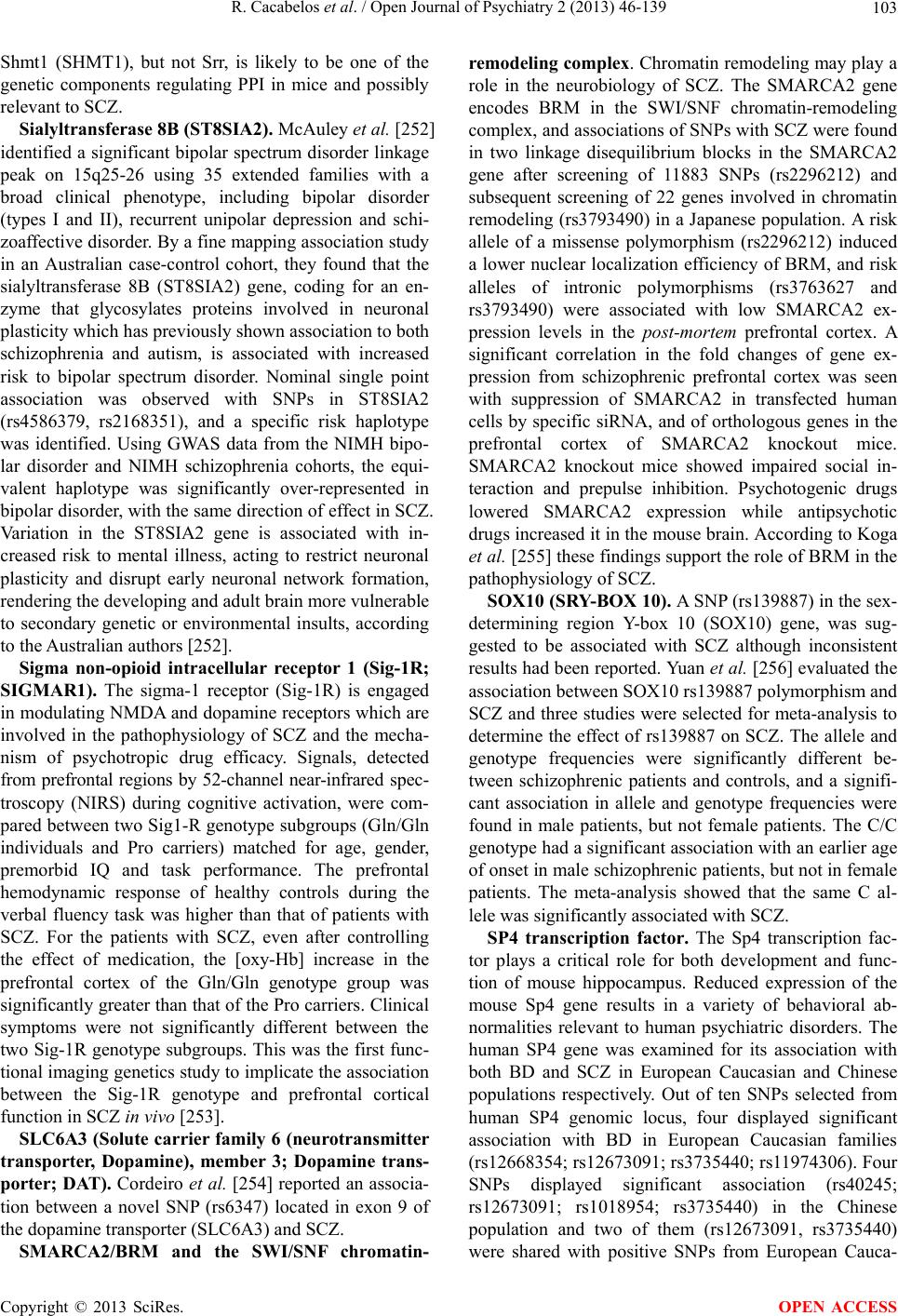 R. Cacabelos et al. / Open Journal of Psychiatry 2 (2013) 46-139 103 Shmt1 (SHMT1), but not Srr, is likely to be one of the genetic components regulating PPI in mice and possibly relevant to SCZ. Sialyltransferase 8B (ST8SIA2). McAuley et al. [252] identified a significant bipolar spectrum disorder linkage peak on 15q25-26 using 35 extended families with a broad clinical phenotype, including bipolar disorder (types I and II), recurrent unipolar depression and schi- zoaffective disorder. By a fine mapping association study in an Australian case-control cohort, they found that the sialyltransferase 8B (ST8SIA2) gene, coding for an en- zyme that glycosylates proteins involved in neuronal plasticity which has previously shown association to both schizophrenia and autism, is associated with increased risk to bipolar spectrum disorder. Nominal single point association was observed with SNPs in ST8SIA2 (rs4586379, rs2168351), and a specific risk haplotype was identified. Using GWAS data from the NIMH bipo- lar disorder and NIMH schizophrenia cohorts, the equi- valent haplotype was significantly over-represented in bipolar disorder, with the same direction of effect in SCZ. Variation in the ST8SIA2 gene is associated with in- creased risk to mental illness, acting to restrict neuronal plasticity and disrupt early neuronal network formation, rendering the developing and adult brain more vulnerable to secondary genetic or environmental insults, according to the Australian authors [252]. Sigma non-opioid intracellular receptor 1 (Sig-1R; SIGMAR1). The sigma-1 receptor (Sig-1R) is engaged in modulating NMDA and dopamine receptors which are involved in the pathophysiology of SCZ and the mecha- nism of psychotropic drug efficacy. Signals, detected from prefrontal regions by 52-channel near-infrared spec- troscopy (NIRS) during cognitive activation, were com- pared between two Sig1-R genotype subgroups (Gln/Gln individuals and Pro carriers) matched for age, gender, premorbid IQ and task performance. The prefrontal hemodynamic response of healthy controls during the verbal fluency task was higher than that of patients with SCZ. For the patients with SCZ, even after controlling the effect of medication, the [oxy-Hb] increase in the prefrontal cortex of the Gln/Gln genotype group was significantly greater than that of the Pro carriers. Clinical symptoms were not significantly different between the two Sig-1R genotype subgroups. This was the first func- tional imaging genetics study to implicate the association between the Sig-1R genotype and prefrontal cortical function in SCZ in vivo [253]. SLC6A3 (Solute carrier family 6 (neurotransmitter transporter, Dopamine), member 3; Dopamine trans- porter; DAT). Cordeiro et al. [254] reported an associa- tion between a novel SNP (rs6347) located in exon 9 of the dopamine transporter (SLC6A3) and SCZ. SMARCA2/BRM and the SWI/SNF chromatin- remodeling complex. Chromatin remodeling may play a role in the neurobiology of SCZ. The SMARCA2 gene encodes BRM in the SWI/SNF chromatin-remodeling complex, and associations of SNPs with SCZ were found in two linkage disequilibrium blocks in the SMARCA2 gene after screening of 11883 SNPs (rs2296212) and subsequent screening of 22 genes involved in chromatin remodeling (rs3793490) in a Japanese population. A risk allele of a missense polymorphism (rs2296212) induced a lower nuclear localization efficiency of BRM, and risk alleles of intronic polymorphisms (rs3763627 and rs3793490) were associated with low SMARCA2 ex- pression levels in the post-mortem prefrontal cortex. A significant correlation in the fold changes of gene ex- pression from schizophrenic prefrontal cortex was seen with suppression of SMARCA2 in transfected human cells by specific siRNA, and of orthologous genes in the prefrontal cortex of SMARCA2 knockout mice. SMARCA2 knockout mice showed impaired social in- teraction and prepulse inhibition. Psychotogenic drugs lowered SMARCA2 expression while antipsychotic drugs increased it in the mouse brain. According to Koga et al. [255] these findings support the role of BRM in the pathophysiology of SCZ. SOX10 (SRY-BOX 10). A SNP (rs139887) in the sex- determining region Y-box 10 (SOX10) gene, was sug- gested to be associated with SCZ although inconsistent results had been reported. Yuan et al. [256] evaluated the association between SOX10 rs139887 polymorphism and SCZ and three studies were selected for meta-analysis to determine the effect of rs139887 on SCZ. The allele and genotype frequencies were significantly different be- tween schizophrenic patients and controls, and a signifi- cant association in allele and genotype frequencies were found in male patients, but not female patients. The C/C genotype had a significant association with an earlier age of onset in male schizophrenic patients, but not in female patients. The meta-analysis showed that the same C al- lele was significantly associated with SCZ. SP4 transcription factor. The Sp4 transcription fac- tor plays a critical role for both development and func- tion of mouse hippocampus. Reduced expression of the mouse Sp4 gene results in a variety of behavioral ab- normalities relevant to human psychiatric disorders. The human SP4 gene was examined for its association with both BD and SCZ in European Caucasian and Chinese populations respectively. Out of ten SNPs selected from human SP4 genomic locus, four displayed significant association with BD in European Caucasian families (rs12668354; rs12673091; rs3735440; rs11974306). Four SNPs displayed significant association (rs40245; rs12673091; rs1018954; rs3735440) in the Chinese population and two of them (rs12673091, rs3735440) were shared with positive SNPs from European Cauca- Copyright © 2013 SciRes. OPEN ACCESS
 R. Cacabelos et al. / Open Journal of Psychiatry 2 (2013) 46-139 104 sian families. Considering the genetic overlap between BD and SCZ, extended studies in Chinese trio families for SCZ revealed that SNP7 (rs12673091) also displayed a significant association. SNP7 (rs12673091) was there- fore significantly associated in all three samples, and shared the same susceptibility allele (A) across all three samples. A gene dosage effect for mouse Sp4 gene in the modulation of sensorimotor gating, a putative endophe- notype for both SCZ and BD, was also found. The defi- cient sensorimotor gating in Sp4 hypomorphic mice was partially reversed by the administration of a dopamine D2 antagonist or mood stabilizers. Both human genetic and mouse pharmacogenetic studies support the Sp4 gene as a susceptibility gene for BD or SCZ [257]. Spermidine/Spermine N1-acetyltransferase (SSAT1; SAT1). Psychotic patients tend to show increased blood and fibroblast total polyamine levels. Spermidine/sper- mine N1-acetyltransferase (SSAT-1) and its coding gene (SAT-1) are the main factors regulating polyamine ca- tabolism. No association between the SAT-1 −1415T/C SNP and SCZ was found in Spanish patients; however, a mild association between allele C and psychopathology was found in the female group [258]. Sulfotransferase 4A1 (SULT4A1). Sulfotransferase 4A1 (SULT4A1) is a novel sulfotransferase expressed almost exclusively in the brain. The gene is located on chromosome 22q13.2, a region implicated in predisposi- tion to SCZ. A variable microsatellite region located up- stream of SULT4A1 was found to be associated with an increase in SCZ risk. If functional dysregulation of SULT4A1 was involved in the etiology of SCZ, then ge- netic variants in the coding sequence of SULT4A1 might be identified in cases compared with controls. A mutation analysis of the coding region (exons 2 - 7) in 71 Austra- lian SCZ cases found no mutations, either synonymous or nonsynonymous. However, intronic variants (IVS5 + 12 C > T and IVS5 + 28 G > C) were identified, the fre- quency of which was not statistically different between cases and controls. The lack of polymorphisms in the coding region of the SULT4A1 gene is highly unusual and, along with its high conservation between species, suggests that SULT4A1 may have an important function in vivo, but recent findings do not support the hypothesis that germline mutations in the coding region of SULT4A1 contribute to susceptibility to SCZ [259]. Synapsin 2-3 (SYN2, SYN3). The synapsin III gene (SYN3), which belongs to the family of synaptic vesi- cle-associated proteins, has been implicated in the modulation of neurotransmitter release and in synapto- genesis, suggesting a potential role in several neuropsy- chiatric diseases. The human SYN3 gene is located on chromosome 22q12-13, a candidate region implicated in previous linkage studies of SCZ. Four SYN3 SNPs (rs133945 (−631C > G), rs133946 (−196G > A), rs9862 and rs1056484) did not show association with SCZ in either Irish or Chinese case-control samples [260]. Stud- ies of SYN3 mRNA expression in human brain regions as well as the methylation specificity in the closest CpG island of this gene indicate that the cytosine methylation in this genomic region is restricted to cytosines in CpG dinucleotides, and is similar in brain regions and blood, and appears conserved in primate evolution. Two cytosi- nes (cytosine 8 and 20) localized as the CpG dinucleotide are partially methylated in all brain regions. The methy- lation of these sites in SCZ and control blood samples is variable. The variation in SYN3 methylation is not re- lated to SCZ or a monozygotic twin pair discordant for SCZ and is not related to the mRNA level of SYN3a in different human brain regions [261]. Synaptogyrin 1 (SYNGR1) . Synaptogyrin 1 (SYNGR1) is a transmembrane protein of neurotransmitter-contain- ing vesicle. Suggestive association between SYNGR1 intragenic polymorphisms and SCZ has been reported in the Indian population. Rare nucleotide changes with a potential pathogenic effect have been found in Indian and Chinese SCZ patients. Evidence of association has been found for rs715505 in the Italian population [262]. Polymorphisms of the synaptogyrin 1 (SYNGR1) and synasin II (SYNII) genes have been shown to be a risk factor for BD or SCZ. A case-control study with these two genes was conducted in 506 BD patients and 507 healthy individuals from the Han Chinese population. No association was found in this study [263]. Synaptosomal-associated protein 25 kDa (SNAP- 25). SNAP-25 (synaptosomal-associated protein of 25 kDa) is a plasma membrane protein that, together with syntaxin and the synaptic vesicle protein VAMP/synap- tobrevin, forms the SNARE (soluble N-ethylmaleimide- sensitive factor attachment protein receptor) docking complex for regulated exocytosis. SNAP-25 also modu- lates different voltage-gated calcium channels, repre- senting therefore a multifunctional protein that plays essential roles in neurotransmitter release at different steps. Recent genetic studies of human populations and of some mouse models implicate alterations in SNAP-25 gene structure, expression, and/or function in contribut- ing directly to these distinct neuropsychiatric and neuro- logical disorders [264]. Both reduced and excessive SNAP-25 activity has been implicated in various disease states that involve cognitive dysfunctions such as ADHD, SCZ and AD. Long-term memory is formed by altera- tions in glutamate-dependent excitatory synaptic trans- mission, which is in turn regulated by SNAP-25, a key component of the SNARE complex essential for exocy- tosis of neurotransmitter-filled synaptic vesicles. Excess SNAP-25 activity, restricted to the adult period, is suffi- cient to mediate significant deficits in the memory for- mation process [265]. Copyright © 2013 SciRes. OPEN ACCESS
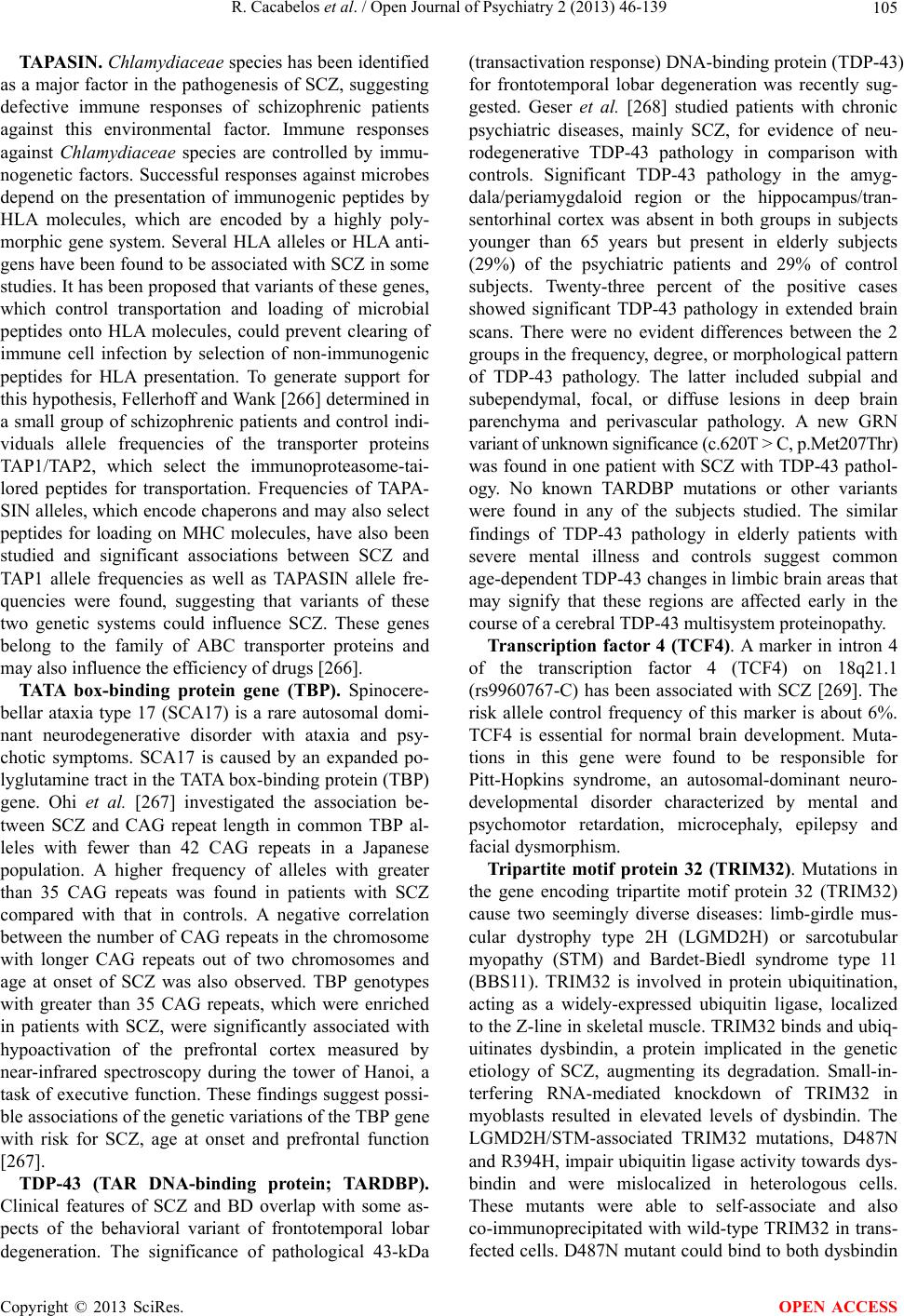 R. Cacabelos et al. / Open Journal of Psychiatry 2 (2013) 46-139 105 TAPASIN. Chlamydiaceae species has been identified as a major factor in the pathogenesis of SCZ, suggesting defective immune responses of schizophrenic patients against this environmental factor. Immune responses against Chlamydiaceae species are controlled by immu- nogenetic factors. Successful responses against microbes depend on the presentation of immunogenic peptides by HLA molecules, which are encoded by a highly poly- morphic gene system. Several HLA alleles or HLA anti- gens have been found to be associated with SCZ in some studies. It has been proposed that variants of these genes, which control transportation and loading of microbial peptides onto HLA molecules, could prevent clearing of immune cell infection by selection of non-immunogenic peptides for HLA presentation. To generate support for this hypothesis, Fellerhoff and Wank [266] determined in a small group of schizophrenic patients and control indi- viduals allele frequencies of the transporter proteins TAP1/TAP2, which select the immunoproteasome-tai- lored peptides for transportation. Frequencies of TAPA- SIN alleles, which encode chaperons and may also select peptides for loading on MHC molecules, have also been studied and significant associations between SCZ and TAP1 allele frequencies as well as TAPASIN allele fre- quencies were found, suggesting that variants of these two genetic systems could influence SCZ. These genes belong to the family of ABC transporter proteins and may also influence the efficiency of drugs [266]. TATA box-binding protein gene (TBP). Spinocere- bellar ataxia type 17 (SCA17) is a rare autosomal domi- nant neurodegenerative disorder with ataxia and psy- chotic symptoms. SCA17 is caused by an expanded po- lyglutamine tract in the TATA box-binding protein (TBP) gene. Ohi et al. [267] investigated the association be- tween SCZ and CAG repeat length in common TBP al- leles with fewer than 42 CAG repeats in a Japanese population. A higher frequency of alleles with greater than 35 CAG repeats was found in patients with SCZ compared with that in controls. A negative correlation between the number of CAG repeats in the chromosome with longer CAG repeats out of two chromosomes and age at onset of SCZ was also observed. TBP genotypes with greater than 35 CAG repeats, which were enriched in patients with SCZ, were significantly associated with hypoactivation of the prefrontal cortex measured by near-infrared spectroscopy during the tower of Hanoi, a task of executive function. These findings suggest possi- ble associations of the genetic variations of the TBP gene with risk for SCZ, age at onset and prefrontal function [267]. TDP-43 (TAR DNA-binding protein; TARDBP). Clinical features of SCZ and BD overlap with some as- pects of the behavioral variant of frontotemporal lobar degeneration. The significance of pathological 43-kDa (transactivation response) DNA-binding protein (TDP-43) for frontotemporal lobar degeneration was recently sug- gested. Geser et al. [268] studied patients with chronic psychiatric diseases, mainly SCZ, for evidence of neu- rodegenerative TDP-43 pathology in comparison with controls. Significant TDP-43 pathology in the amyg- dala/periamygdaloid region or the hippocampus/tran- sentorhinal cortex was absent in both groups in subjects younger than 65 years but present in elderly subjects (29%) of the psychiatric patients and 29% of control subjects. Twenty-three percent of the positive cases showed significant TDP-43 pathology in extended brain scans. There were no evident differences between the 2 groups in the frequency, degree, or morphological pattern of TDP-43 pathology. The latter included subpial and subependymal, focal, or diffuse lesions in deep brain parenchyma and perivascular pathology. A new GRN variant of unknown significance (c.620T > C, p.Met207Thr) was found in one patient with SCZ with TDP-43 pathol- ogy. No known TARDBP mutations or other variants were found in any of the subjects studied. The similar findings of TDP-43 pathology in elderly patients with severe mental illness and controls suggest common age-dependent TDP-43 changes in limbic brain areas that may signify that these regions are affected early in the course of a cerebral TDP-43 multisystem proteinopathy. Transcription factor 4 (TCF4). A marker in intron 4 of the transcription factor 4 (TCF4) on 18q21.1 (rs9960767-C) has been associated with SCZ [269]. The risk allele control frequency of this marker is about 6%. TCF4 is essential for normal brain development. Muta- tions in this gene were found to be responsible for Pitt-Hopkins syndrome, an autosomal-dominant neuro- developmental disorder characterized by mental and psychomotor retardation, microcephaly, epilepsy and facial dysmorphism. Tripartite motif protein 32 (TRIM32). Mutations in the gene encoding tripartite motif protein 32 (TRIM32) cause two seemingly diverse diseases: limb-girdle mus- cular dystrophy type 2H (LGMD2H) or sarcotubular myopathy (STM) and Bardet-Biedl syndrome type 11 (BBS11). TRIM32 is involved in protein ubiquitination, acting as a widely-expressed ubiquitin ligase, localized to the Z-line in skeletal muscle. TRIM32 binds and ubiq- uitinates dysbindin, a protein implicated in the genetic etiology of SCZ, augmenting its degradation. Small-in- terfering RNA-mediated knockdown of TRIM32 in myoblasts resulted in elevated levels of dysbindin. The LGMD2H/STM-associated TRIM32 mutations, D487N and R394H, impair ubiquitin ligase activity towards dys- bindin and were mislocalized in heterologous cells. These mutants were able to self-associate and also co-immunoprecipitated with wild-type TRIM32 in trans- fected cells. D487N mutant could bind to both dysbindin Copyright © 2013 SciRes. OPEN ACCESS
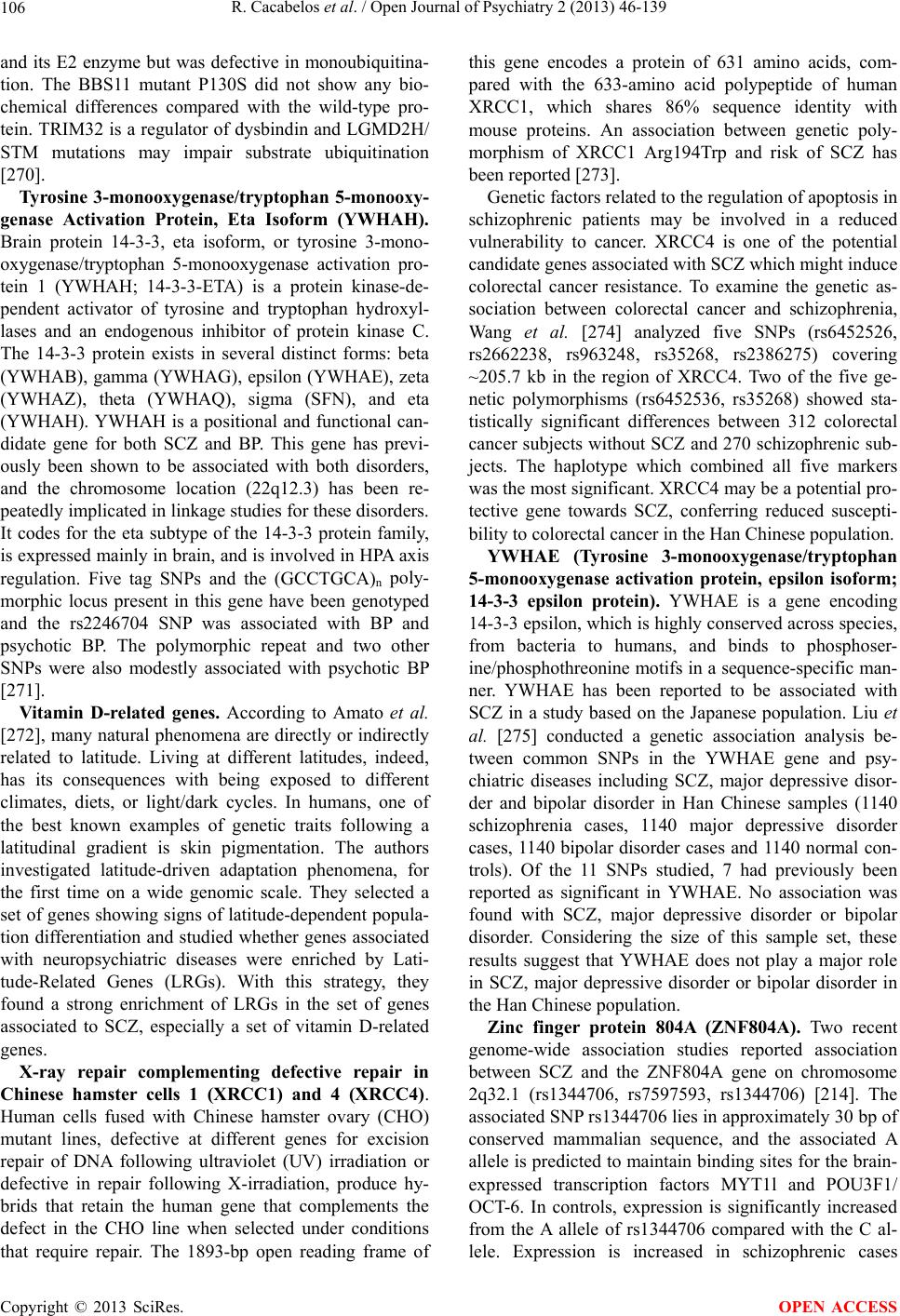 R. Cacabelos et al. / Open Journal of Psychiatry 2 (2013) 46-139 106 and its E2 enzyme but was defective in monoubiquitina- tion. The BBS11 mutant P130S did not show any bio- chemical differences compared with the wild-type pro- tein. TRIM32 is a regulator of dysbindin and LGMD2H/ STM mutations may impair substrate ubiquitination [270]. Tyrosine 3-monooxygenase/tryptophan 5-monooxy- genase Activation Protein, Eta Isoform (YWHAH). Brain protein 14-3-3, eta isoform, or tyrosine 3-mono- oxygenase/tryptophan 5-monooxygenase activation pro- tein 1 (YWHAH; 14-3-3-ETA) is a protein kinase-de- pendent activator of tyrosine and tryptophan hydroxyl- lases and an endogenous inhibitor of protein kinase C. The 14-3-3 protein exists in several distinct forms: beta (YWHAB), gamma (YWHAG), epsilon (YWHAE), zeta (YWHAZ), theta (YWHAQ), sigma (SFN), and eta (YWHAH). YWHAH is a positional and functional can- didate gene for both SCZ and BP. This gene has previ- ously been shown to be associated with both disorders, and the chromosome location (22q12.3) has been re- peatedly implicated in linkage studies for these disorders. It codes for the eta subtype of the 14-3-3 protein family, is expressed mainly in brain, and is involved in HPA axis regulation. Five tag SNPs and the (GCCTGCA)n poly- morphic locus present in this gene have been genotyped and the rs2246704 SNP was associated with BP and psychotic BP. The polymorphic repeat and two other SNPs were also modestly associated with psychotic BP [271]. Vitamin D-related genes. According to Amato et al. [272], many natural phenomena are directly or indirectly related to latitude. Living at different latitudes, indeed, has its consequences with being exposed to different climates, diets, or light/dark cycles. In humans, one of the best known examples of genetic traits following a latitudinal gradient is skin pigmentation. The authors investigated latitude-driven adaptation phenomena, for the first time on a wide genomic scale. They selected a set of genes showing signs of latitude-dependent popula- tion differentiation and studied whether genes associated with neuropsychiatric diseases were enriched by Lati- tude-Related Genes (LRGs). With this strategy, they found a strong enrichment of LRGs in the set of genes associated to SCZ, especially a set of vitamin D-related genes. X-ray repair complementing defective repair in Chinese hamster cells 1 (XRCC1) and 4 (XRCC4). Human cells fused with Chinese hamster ovary (CHO) mutant lines, defective at different genes for excision repair of DNA following ultraviolet (UV) irradiation or defective in repair following X-irradiation, produce hy- brids that retain the human gene that complements the defect in the CHO line when selected under conditions that require repair. The 1893-bp open reading frame of this gene encodes a protein of 631 amino acids, com- pared with the 633-amino acid polypeptide of human XRCC1, which shares 86% sequence identity with mouse proteins. An association between genetic poly- morphism of XRCC1 Arg194Trp and risk of SCZ has been reported [273]. Genetic factors related to the regulation of apoptosis in schizophrenic patients may be involved in a reduced vulnerability to cancer. XRCC4 is one of the potential candidate genes associated with SCZ which might induce colorectal cancer resistance. To examine the genetic as- sociation between colorectal cancer and schizophrenia, Wang et al. [274] analyzed five SNPs (rs6452526, rs2662238, rs963248, rs35268, rs2386275) covering ~205.7 kb in the region of XRCC4. Two of the five ge- netic polymorphisms (rs6452536, rs35268) showed sta- tistically significant differences between 312 colorectal cancer subjects without SCZ and 270 schizophrenic sub- jects. The haplotype which combined all five markers was the most significant. XRCC4 may be a potential pro- tective gene towards SCZ, conferring reduced suscepti- bility to colorectal cancer in the Han Chinese population. YWHAE (Tyrosine 3-monooxygenase/tryptophan 5-monooxygenase activation protein, epsilon isoform; 14-3-3 epsilon protein). YWHAE is a gene encoding 14-3-3 epsilon, which is highly conserved across species, from bacteria to humans, and binds to phosphoser- ine/phosphothreonine motifs in a sequence-specific man- ner. YWHAE has been reported to be associated with SCZ in a study based on the Japanese population. Liu et al. [275] conducted a genetic association analysis be- tween common SNPs in the YWHAE gene and psy- chiatric diseases including SCZ, major depressive disor- der and bipolar disorder in Han Chinese samples (1140 schizophrenia cases, 1140 major depressive disorder cases, 1140 bipolar disorder cases and 1140 normal con- trols). Of the 11 SNPs studied, 7 had previously been reported as significant in YWHAE. No association was found with SCZ, major depressive disorder or bipolar disorder. Considering the size of this sample set, these results suggest that YWHAE does not play a major role in SCZ, major depressive disorder or bipolar disorder in the Han Chinese population. Zinc finger protein 804A (ZNF804A). Two recent genome-wide association studies reported association between SCZ and the ZNF804A gene on chromosome 2q32.1 (rs1344706, rs7597593, rs1344706) [214]. The associated SNP rs1344706 lies in approximately 30 bp of conserved mammalian sequence, and the associated A allele is predicted to maintain binding sites for the brain- expressed transcription factors MYT1l and POU3F1/ OCT-6. In controls, expression is significantly increased from the A allele of rs1344706 compared with the C al- lele. Expression is increased in schizophrenic cases Copyright © 2013 SciRes. OPEN ACCESS
 R. Cacabelos et al. / Open Journal of Psychiatry 2 (2013) 46-139 107 compared with controls, but this difference does not achieve statistical significance. This study replicates the original reported association of ZNF804A with SCZ and suggests that there is a consistent link between the A al- lele of rs1344706, increased expression of ZNF804A and risk for SCZ [276]. ZNF804A rs1344706 is the first genetic risk variant to achieve genome-wide significance for psychosis. Fol- lowing earlier evidence that patients carrying the ZNF804A risk allele had relatively spared memory func- tion compared to patient non-carriers, Donohoe et al. [277] investigated whether ZNF804A was also associ- ated with variation in brain volume. In a sample of 70 patients and 38 healthy participants they used voxel- based morphometry to compare homozygous (AA) car- riers of the ZNF804A risk allele to heterozygous and homozygous (AC/CC) non-carriers for both whole brain volume and specific regions implicated in earlier ZNF804A studies (the dorsolateral pre-frontal cortex, the hippocampus, and the amygdala). Homozygous SCZ “AA” risk carriers had relatively larger gray matter vol- umes than heterozygous/homozygous non-carriers (AC/CC), particularly for hippocampal volumes. ZNF804A might be delineating a SCZ subtype characterized by relatively intact brain volume. Dwyer et al. [278] undertook a study to identify whether rare (frequency ~0.001%) coding variants in the SCZ susceptibility gene ZNF804A are involved in this psychotic disorder. No single rare variant was associated with SCZ, nor was the burden of rare, or even fairly common, non-synonymous variants. These results do not support the hypothesis that moderately rare non-syn- onymous variants at the ZNF804A locus are involved in schizophrenia susceptibility. As the first gene to have achieved genome-wide sig- nificance for psychosis, ZNF804A has predictably been a subject of intense research activity. Donohoe et al. [279] reviewed the evidence to date for the association be- tween SCZ and the original risk variant rs1344706, as well as additional common and rare variants at this locus, and concluded that ZNF804A is robustly, if modestly, associated with SCZ risk. For markers rs4667000 and rs1366842, significant differences in allele frequencies were found between cases and controls in the Chinese Han population. Ana- lysis of haplotype rs61739290-rs1366842 showed sig- nificant association with SCZ. A meta-analysis com- prised of studies that utilized sample sets of either Euro- pean and/or Han Chinese origin revealed statistically significant associations for two SNPs (rs1366842, rs3731834) and SCZ. MicroRNAs (miRNAs) are small non-coding RNAs that mainly function as negative regulators of gene ex- pression and have been shown to be involved in schizo- phrenia etiology through genetic and expression studies. A polymorphism (rs1625579) located in the primary transcript of a miRNA gene, hsa-miR-137, was reported to be strongly associated with SCZ. Four SCZ loci (CACNA1C, TCF4, CSMD1, C10orf26) were predicted as hsa-miR-137 targets, and Kim et al. [280] showed that ZNF804A is also a target for hsa-miR-137. 3.3. Copy Number Variants and Cytogenetic Anomalies The mechanisms underlying generation of neuronal va- riability and complexity still remain enigmatic, and rep- resent the central challenge for neuroscience. Structural variation in the neuronal genome is likely to be a rele- vant feature of neuronal diversity and brain dysfunction. Large-scale genomic variations due to loss or gain of whole chromosomes (aneuploidy) have been described in cells of the normal and diseased human brain, which are generated from neural stem cells during the intrauterine period of life. In studies with more than 600,000 neural cells, it has been found that the average aneuploidy fre- quency is 1.25% - 1.45% per chromosome, with the overall percentage of aneuploidy tending to approach 30% - 35%, and that mosaic aneuploidy may be exclu- sively confined to the brain. These findings might indi- cate that 1) aneuploidization could be an additional pathological mechanism for neuronal genome diversifi- cation; 2) aneuploidy is involved in brain development; and 3) a link between developmental chromosomal in- stability and intercellular/intertissular genome diversity might be associated with pathogenic mechanisms under- lying specific CNS disorders [281]. Using DNA probes for chromosomes 1, 7, 11, 13, 14, 17, 18, 21, X and Y, the mean rate of stochastic aneup- loidy per chromosome is 0.5% in the normal human brain. The overall proportion of aneuploid cells in the normal brain has been estimated at approximately 10%. The overall proportion of aneuploid cells in the brain of patients with ataxia-telangiectasia was estimated at ap- proximately 20% - 50%. A dramatic 10-fold increase of chromosome 21-specific aneuploidy (both hypoploidy and hyperploidy) was detected in the AD cerebral cortex (6% - 15% vs 0.8% - 1.8% in control). It appears that somatic mosaic aneuploidy differentially contributes to intercellular genomic variation in the brain, probably influencing brain dysfunction and neurodegeneration [282]. Brain aneuploidy was hypothesized to be involved in the pathogenesis of SCZ. In normal brains, average fre- quencies of stochastic chromosome 1 loss and gain were 0.3% and 0.3%, respectively, with a threshold level for stochastic chromosome gain and loss of 0.7%. Average rate of aneuploidy in SCZ brains is 0.9% for chromo- some 1 loss and 0.9% for chromosome 1 gain. Signifi- Copyright © 2013 SciRes. OPEN ACCESS
 R. Cacabelos et al. / Open Journal of Psychiatry 2 (2013) 46-139 108 cantly increased level of mosaic aneuploidy involving chromosome 1 was revealed in brains (3.6% and 4.7% of cells with chromosome 1 loss and gain, respectively). Stochastic aneuploidy rate for chromosome 1 in SCZ brains reached 0.6% for loss and 0.5% for gain and was higher than in controls, suggesting that subtle genomic imbalances manifesting as low-level mosaic aneuploidy may contribute to SCZ pathogenesis [283]. Copy number variants (CNVs) have been identified in individual patients with SCZ and also in neurodevelop- mental disorders [284]. A genome-wide survey of rare CNVs in 3391 patients with SCZ and 3181 ancestrally matched controls, using high-density microarrays, de- tected CNVs in less than 1% of the sample with 100 kb in length. The total burden was increased 1.15-fold in patients with SCZ in comparison with controls. Deletions were found within the region critical for velo-cardio- facial syndrome, which includes psychotic symptoms in 30% of patients. Associations with SCZ were also found for large deletions on chromosome 15q13.3 and 1q21.1 [184]. CNVs have been shown to increase the risk to develop SCZ. The best supported findings are at 1q21.1, 15q11.2, 15q13.3, 16p13.1, 16p13.11 and 22q11.2, and deletions at the gene neurexin 1 (NRXN1). In the Japa- nese population, as in other Western populations, there is a trend for excess of rare CNVs in SCZ; however, previ- ously implicated association for very large CNVs (>500 kb) could not be confirmed in this population [285]. In a genome-wide search for CNVs associating with SCZ, 66 de novo CNVs were identified in a sample of 1433 SCZ cases and 33250 controls. Three deletions at 1q21.1, 15q11.2 and 15q13.3 showing nominal association with SCZ in the first sample (phase I) were followed up in a second sample of 3285 cases and 7951 controls (phase II). All three deletions significantly associate with SCZ and related psychoses in the combined sample [208]. There are 484 annotated genes located on 8p; many are most likely oncogenes and tumor-suppressor genes. Molecular genetics and developmental studies have iden- tified 21 genes in this region (ADRA1A, ARHGEF10, CHRNA2, CHRNA6, CHRNB3, DKK4, DPYSL2, EGR3, FGF17, FGF20, FGFR1, FZD3, LDL, NAT2, NEF3, NRG1, PCM1, PLAT, PPP3CC, SFRP1 and VMAT1/SLC18A1) that are most likely to contribute to neuropsychiatric disorders (SCZ, autism, BD and de- pression), neurodegenerative disorders (Parkinson’s and Alzheimer diseases) and cancer. At least seven nonpro- tein-coding RNAs (microRNAs) are located at 8p. Structural variants on 8p, such as copy number variants, microdeletions or microduplications, might also contrib- ute to autism, SCZ and other human diseases including cancer [286]. A genome-wide assessment of SNPs and CNVs in 1460 patients with SCZ and 12,995 controls of European ancestry identified 8 cases and zero controls with deletions greater than 2 Mb, of which two, at 8p22 and 16p13.11-p12.4, were novel. A further evaluation of 1378 controls identified no deletions greater than 2 Mb, suggesting a high prior probability of disease involve- ment when such deletions are observed in cases. Further evidence for some smaller SCZ-associated CNVs, such as those in NRXN1 and APBA2, was confirmed; how- ever, this study could not provide strong support for the hypothesis that SCZ patients have a significantly greater “load” of large (>100 kb), rare CNVs, nor could it find common CNVs that associate with SCZ or SCZ-associ- ated CNVs that disrupt genes in neurodevelopmental pathways [287]. Kirov et al. [288] investigated the involvement of rare (<1%) copy number variants (CNVs) in 471 cases of SCZ and 2792 controls that had been genotyped using the Affymetrix GeneChip 500K Mapping Array. Large CNVs >1 Mb were 2.26 times more common in cases, with the effect coming mostly from deletions, although duplications were also more common. Two large dele- tions were found in two cases each, but in no controls: a deletion at 22q11.2 known to be a susceptibility factor for SCZ and a deletion on 17p12, at 14.0 - 15.4 Mb. The latter is known to cause hereditary neuropathy with li- ability to pressure palsies. The same deletion was found in 6 of 4618 (0.13%) cases and 6 of 36,092 (0.017%) controls in the re-analyzed data of two recent large CNV studies of SCZ. One large duplication on 16p13.1, which has been previously implicated as a susceptibility factor for autism, was found in three cases and six controls (0.6% vs 0.2%). This study also provided the first sup- port for a recently reported association between deletions at 15q11.2 and SCZ [288]. A susceptibility locus in 13q13-q14 is shared by SCZ and mood disorder, and that locus would be additional to another well-documented and more distal 13q locus where the G72/G30 gene is mapped [289]. Idiopathic generalized epilepsies account for 30% of all epilepsies. Microdeletions at 15q13.3 have recently been shown to constitute a strong genetic risk factor for common idiopathic generalized epilepsy syndromes, implicating that other recurrent microdeletions may also be involved in epileptogenesis. Five microdeletions at the genomic hotspot regions 1q21.1, 15q11.2, 16p11.2, 16p13.11 and 22q11.2 represent genetic risk to common idiopathic generalized epilepsy syndromes and other neuropsychiatric disorders [290]. Microdeletion at chromosomal position 15q13.3 has been described in intellectual disability, autism spectrum disorders, SCZ and in idiopathic generalized epilepsy [291]. The pheno- typic profile of children with microdeletions of 15q13.3 includes developmental delay, mental retardation, or borderline IQ, autistic spectrum disorder, speech delay, aggressiveness, attention-deficit hyperactivity disorder, Copyright © 2013 SciRes. OPEN ACCESS
 R. Cacabelos et al. / Open Journal of Psychiatry 2 (2013) 46-139 109 and other behavioral problems [292]. Positive genetic linkage to the 15q13-q14 region has been found in many studies, and several association reports support this locus as a candidate region for SCZ. A candidate gene in the region, the alpha7 nicotinic receptor CHRNA7, plays a seminal role in the linked endophenotype, and is de- creased in expression in the patient population. The 15q13-q14 region contains a partial duplication of the CHRNA7 gene that includes exons 5 - 10 and consider- able sequence downstream. Evidence from multiple studies supports a broad region of genetic linkage around the marker D15S1360 [293]. Autism and mental retardation show high rates of co- morbidity and potentially share genetic risk factors. A rare approximately 2 Mb microdeletion involving chro- mosome band 15q13.3 was detected in a multiplex au- tism family. This genomic loss lies between distal break points of the Prader-Willi/Angelman syndrome locus and was first described in association with mental retardation and epilepsy. Together with recent studies that have also implicated this genomic imbalance in SCZ, this CNV shows considerable phenotypic variability [294]. Deletions and reciprocal duplications of the chromo- some 16p13.1 region have been reported in several cases of autism, mental retardation and SCZ. Ingason et al. [295] found a threefold excess of duplications and dele- tions in SCZ cases compared with controls, with duplica- tions present in 0.30% of cases vs 0.09% of controls and deletions in 0.12% of cases and 0.04% of controls. The region can be divided into three intervals defined by flanking low copy repeats. Duplications spanning inter- vals I and II showed the most significant association with SCZ. The age of onset in duplication and deletion carri- ers among cases ranged from 12 to 35 years, and the majority were males with a family history of psychiatric disorders. Candidate genes in the region include NTAN1 and NDE1. Velocardiofacial syndrome, now known as 22q11.2 deletion syndrome (22qDS), is estimated to affect more than 700 children born in the United States each year. Some clinical studies have found increased rates of SCZ in adults with 22qDS. The psychiatric disorders most commonly reported in children and adolescents with 22qDS have been attention-deficit hyperactivity disorder, oppositional defiant disorder, anxiety disorders, and ma- jor depression. Psychotic symptoms have been observed in 14% to 28% of children with 22qDS [296]. Chromo- some 22q11.2 deletion syndrome (22q11DS) is associ- ated with cognitive deficits and morphometric brain ab- normalities in childhood and a markedly elevated risk of SCZ in adolescence/early adulthood. Children with 22q11DS demonstrate gray matter reductions in multiple brain regions that are thought to be relevant to SCZ. The correlation of these volumetric reductions with poor neurocognition indicates that these brain regions may mediate higher neurocognitive functions implicated in SCZ [297]. Recurrent or overlapping CNVs were found in cases at 39.3% of selected loci. The collective fre- quency of CNVs at these loci is significantly increased in cases with autism, in cases with SCZ, and in cases with mental retardation compared with controls in France. Individual significance was reached for the association between autism and a 350-kilobase deletion located at 22q11 and spanning the PRODH and DGCR6 genes [298]. The 22q11 deletion (or DiGeorge) syndrome (22q11DS), the result of a 1.5- to 3-megabase hemizy- gous deletion on human chromosome 22, results in dra- matically increased susceptibility for “diseases of corti- cal connectivity” thought to arise during development, including SCZ and autism. Diminished dosage of the genes deleted in the 1.5-megabase 22q11 minimal critical deleted region in a mouse model of 22q11DS specifically compromises neurogenesis and subsequent differentia- tion in the cerebral cortex [299]. There is overwhelming evidence that children and adults with 22q11.2 deletion syndrome (22q11.2DS) have a characteristic behavioral phenotype. In particular, there is a growing body of evi- dence that indicates an unequivocal association between 22q11.2DS and SCZ, especially in adulthood. Deletion of 22q11.2 is the third highest risk for the development of SCZ, with a greater risk only conferred by being the child of two parents with SCZ or the monozygotic co-twin of an affected individual. Both linkage and asso- ciation studies of people with SCZ have implicated sev- eral susceptibility genes, of which three are in the 22q11.2 region: catechol-o-methyltransferase (COMT), proline dehydrogenase (PRODH), and Gnb1L. In addi- tion, variation in Gnb1L is associated with the presence of psychosis in males with 22q11.2DS. In mouse models of 22q11.2DS, haploinsufficiency of Tbx1 and Gnb1L is associated with reduced prepulse inhibition, a SCZ endophenotype [300]. Initial studies of genome-wide trinucleotide repeats using the repeat expansion detection technique suggested possible association of large CAG/CTG repeat tracts with SCZ and bipolar affective disorder [301]. Tandem repeats, particularly with long (>50 bp) repeat units, are a relatively common yet underexplored type of CNV that may significantly contribute to human genomic variation and disease risk. A bacterial artificial chromosome-based array comparative genomic hybridization (aCGH) plat- form screen detected an apparent deletion on 5p15.1 in two probands, caused by the presence in each proband of two low copy number (short) alleles of a tandem repeat that ranges in length from fewer than 10 to greater than 50 3.4 kb units in the population examined. Short alleles partially segregate with SCZ in a small number of fami- lies [302]. Copyright © 2013 SciRes. OPEN ACCESS
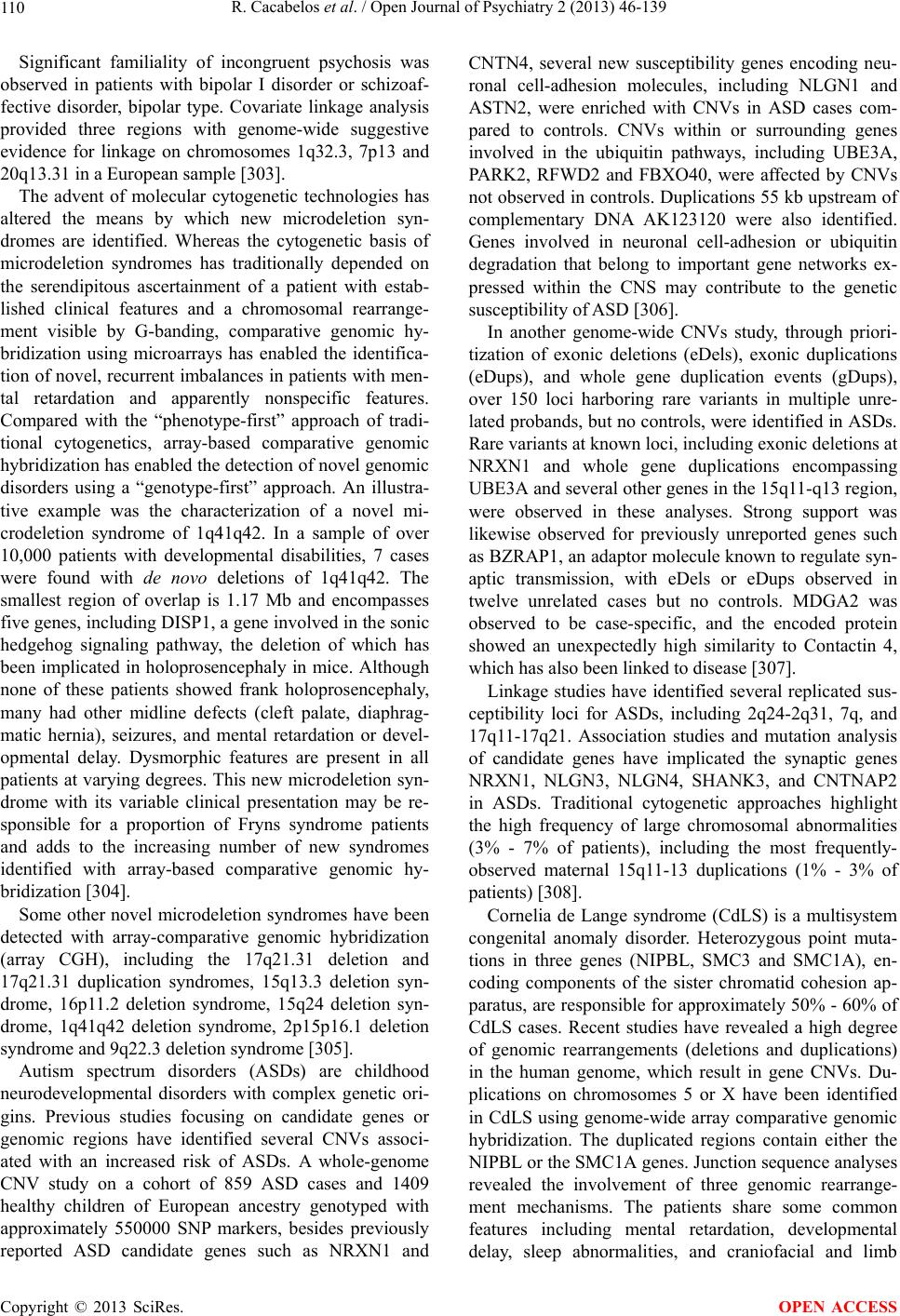 R. Cacabelos et al. / Open Journal of Psychiatry 2 (2013) 46-139 110 Significant familiality of incongruent psychosis was observed in patients with bipolar I disorder or schizoaf- fective disorder, bipolar type. Covariate linkage analysis provided three regions with genome-wide suggestive evidence for linkage on chromosomes 1q32.3, 7p13 and 20q13.31 in a European sample [303]. The advent of molecular cytogenetic technologies has altered the means by which new microdeletion syn- dromes are identified. Whereas the cytogenetic basis of microdeletion syndromes has traditionally depended on the serendipitous ascertainment of a patient with estab- lished clinical features and a chromosomal rearrange- ment visible by G-banding, comparative genomic hy- bridization using microarrays has enabled the identifica- tion of novel, recurrent imbalances in patients with men- tal retardation and apparently nonspecific features. Compared with the “phenotype-first” approach of tradi- tional cytogenetics, array-based comparative genomic hybridization has enabled the detection of novel genomic disorders using a “genotype-first” approach. An illustra- tive example was the characterization of a novel mi- crodeletion syndrome of 1q41q42. In a sample of over 10,000 patients with developmental disabilities, 7 cases were found with de novo deletions of 1q41q42. The smallest region of overlap is 1.17 Mb and encompasses five genes, including DISP1, a gene involved in the sonic hedgehog signaling pathway, the deletion of which has been implicated in holoprosencephaly in mice. Although none of these patients showed frank holoprosencephaly, many had other midline defects (cleft palate, diaphrag- matic hernia), seizures, and mental retardation or devel- opmental delay. Dysmorphic features are present in all patients at varying degrees. This new microdeletion syn- drome with its variable clinical presentation may be re- sponsible for a proportion of Fryns syndrome patients and adds to the increasing number of new syndromes identified with array-based comparative genomic hy- bridization [304]. Some other novel microdeletion syndromes have been detected with array-comparative genomic hybridization (array CGH), including the 17q21.31 deletion and 17q21.31 duplication syndromes, 15q13.3 deletion syn- drome, 16p11.2 deletion syndrome, 15q24 deletion syn- drome, 1q41q42 deletion syndrome, 2p15p16.1 deletion syndrome and 9q22.3 deletion syndrome [305]. Autism spectrum disorders (ASDs) are childhood neurodevelopmental disorders with complex genetic ori- gins. Previous studies focusing on candidate genes or genomic regions have identified several CNVs associ- ated with an increased risk of ASDs. A whole-genome CNV study on a cohort of 859 ASD cases and 1409 healthy children of European ancestry genotyped with approximately 550000 SNP markers, besides previously reported ASD candidate genes such as NRXN1 and CNTN4, several new susceptibility genes encoding neu- ronal cell-adhesion molecules, including NLGN1 and ASTN2, were enriched with CNVs in ASD cases com- pared to controls. CNVs within or surrounding genes involved in the ubiquitin pathways, including UBE3A, PARK2, RFWD2 and FBXO40, were affected by CNVs not observed in controls. Duplications 55 kb upstream of complementary DNA AK123120 were also identified. Genes involved in neuronal cell-adhesion or ubiquitin degradation that belong to important gene networks ex- pressed within the CNS may contribute to the genetic susceptibility of ASD [306]. In another genome-wide CNVs study, through priori- tization of exonic deletions (eDels), exonic duplications (eDups), and whole gene duplication events (gDups), over 150 loci harboring rare variants in multiple unre- lated probands, but no controls, were identified in ASDs. Rare variants at known loci, including exonic deletions at NRXN1 and whole gene duplications encompassing UBE3A and several other genes in the 15q11-q13 region, were observed in these analyses. Strong support was likewise observed for previously unreported genes such as BZRAP1, an adaptor molecule known to regulate syn- aptic transmission, with eDels or eDups observed in twelve unrelated cases but no controls. MDGA2 was observed to be case-specific, and the encoded protein showed an unexpectedly high similarity to Contactin 4, which has also been linked to disease [307]. Linkage studies have identified several replicated sus- ceptibility loci for ASDs, including 2q24-2q31, 7q, and 17q11-17q21. Association studies and mutation analysis of candidate genes have implicated the synaptic genes NRXN1, NLGN3, NLGN4, SHANK3, and CNTNAP2 in ASDs. Traditional cytogenetic approaches highlight the high frequency of large chromosomal abnormalities (3% - 7% of patients), including the most frequently- observed maternal 15q11-13 duplications (1% - 3% of patients) [308]. Cornelia de Lange syndrome (CdLS) is a multisystem congenital anomaly disorder. Heterozygous point muta- tions in three genes (NIPBL, SMC3 and SMC1A), en- coding components of the sister chromatid cohesion ap- paratus, are responsible for approximately 50% - 60% of CdLS cases. Recent studies have revealed a high degree of genomic rearrangements (deletions and duplications) in the human genome, which result in gene CNVs. Du- plications on chromosomes 5 or X have been identified in CdLS using genome-wide array comparative genomic hybridization. The duplicated regions contain either the NIPBL or the SMC1A genes. Junction sequence analyses revealed the involvement of three genomic rearrange- ment mechanisms. The patients share some common features including mental retardation, developmental delay, sleep abnormalities, and craniofacial and limb Copyright © 2013 SciRes. OPEN ACCESS
 R. Cacabelos et al. / Open Journal of Psychiatry 2 (2013) 46-139 111 defects. The systems affected are the same as in CdLS, but clinical manifestations are distinct from CdLS; par- ticularly the absence of the CdLS facial gestalt. The re- sults confirm the notion that duplication CNV of genes can be a common mechanism for human genetic diseases [309]. The rate of de novo mutations is of relevance to evolu- tion and disease. Kong et al. [310] conducted a study of genome-wide mutation rates by sequencing the entire genomes of 78 Icelandic parent-offspring trios at high coverage, and showed that with an average father’s age of 29.7, the average de novo mutation rate is 1.20 × 10−8 per nucleotide per generation. The diversity in mutation rate of single nucleotide polymorphisms is dominated by the age of the father at conception of the child. The effect is an increase of about two mutations per year with pa- ternal mutations doubling every 16.5 years. According to Steffanson’s group [310], father’s age is estimated to explain nearly all of the remaining variation in the de novo mutation counts with potential repercussion on the risk of diseases such as SCZ and autism. 3.4. SNPs in Human miRNA Genes MicroRNAs (miRNAs) are 21 - 25-nucleotide-long, non- coding RNAs involved in translational regulation. Most miRNAs derive from a two-step sequential processing: the generation of pre-miRNA from pri-miRNA by the Drosha/DGCR8 complex in the nucleus, and the genera- tion of mature miRNAs from pre-miRNAs by the Dicer/TRBP complex in the cytoplasm. Sequence varia- tion around the processing sites, and sequence variations in the mature miRNA, especially the seed sequence, may have profound effects on miRNA biogenesis and func- tion. Naturally occurring SNPs can impair or enhance miRNA processing as well as alter the sites of processing. Since miRNAs are small functional units, single base changes in both the precursor elements as well as the mature miRNA sequence may drive the evolution of new microRNAs by altering their biological function. At least 24 human X-linked miRNA variants with potential in- fluence in SCZ have been identified [311]. Individual microRNAs (miRNAs) affect moderate downregulation of gene expression. Components required for miRNA processing and/or function have also been implicated in X-linked mental retardation, neurological and neoplastic diseases, pointing to the wide-ranging involvement of miRNAs in disease. To explore the role of miRNAs in SCZ, 59 microRNA genes on the X-chromosome were amplified and sequenced in males with and without SCZ spectrum disorders to test the hypothesis that ultra-rare mutations in microRNA collectively contribute to the risk of SCZ. Feng et al. [312] provided the first associa- tion of microRNA gene dysfunction with SCZ. Eight ultra-rare variants in the precursor or mature miRNA were identified in eight distinct miRNA genes in 4% of analyzed males with SCZ. One ultra-rare variant was identified in a control sample. These variants were not found in an additional 7197 control X-chromosomes. Functional analyses of ectopically expressed copies of the variant miRNA precursors demonstrate loss of func- tion, gain of function or altered expression levels. These findings suggest that microRNA mutations can contrib- ute to SCZ [312]. At least one third of known miRNA genes are ex- pressed in the brain. Mutations disrupting MECP2 pro- tein lead to abnormal development of the brain and re- sulting behavior. MiR-130b expressed in the brain and potentially targeting MECP2 is located in the susceptibil- ity locus for SCZ (22q11). Screening for mutations has identified a population polymorphism in the 5-upstream miR-130b gene region containing DNA elements for putative transcription factors. Genetic association analy- sis of 300 schizophrenics and 316 controls revealed no statistically significant association of any of the miR- 130b allelic variants with SCZ [313]. For posttranscriptional gene silencing, one strand of the miRNA is used to guide components of the RNA interference machinery, including Argonaute 2, to mes- senger RNAs (mRNAs) with complementary sequences. Targeted mRNAs are either cleaved by the endonuclease Argonaute 2, or protein synthesis is blocked by a specific mechanism. Genes encoding miRNAs are transcribed as long primary miRNAs (pri-miRNAs) that are sequen- tially processed by components of the nucleus and cyto- plasm to yield a mature, approx 22-nucleotide (nt)-long miRNA. Two members of the ribonuclease (RNase) III endonuclease protein family, Drosha and Dicer, have been implicated in this two-step processing. Several pro- teins are required for the initial nuclear processing of pri-miRNAs to the approx 60- to 70-nt stem-loop inter- mediates known as precursor miRNAs (pre-miRNAs). A protein complex, termed Microprocessor by Gregory et al. [314], is necessary and sufficient for processing pri-miRNA to premiRNAs. The Microprocessor complex comprises Drosha and the double-stranded RNA-binding protein DiGeorge syndrome critical region 8 gene (DGCR8), which is deleted in DiGeorge syndrome [314]. Identification of known miRNA targets on all human genes indicates that miRNA-346 targets SCZ suscept- ibility genes listed in the Schizophrenia Gene database twice as frequently as expected, relative to other genes in the genome. The gene encoding this miRNA, miR-346, is located in intron 2 of the glutamate receptor ionotropic delta 1 (GRID1) gene, which has been previously impli- cated in SCZ susceptibility. Expression of both miR-346 and GRID1 is lower in SCZ patients than in normal con- trols; however, the expression of miR-346 and GRID1 is less correlated in SCZ patients than in bipolar patients or Copyright © 2013 SciRes. OPEN ACCESS
 R. Cacabelos et al. / Open Journal of Psychiatry 2 (2013) 46-139 112 in normal controls [315]. Cummings et al. [316] geno- typed 821 patients with confirmed DSM-IV diagnoses of SCZ, bipolar affective disorder I and schizoaffective dis- order for the risk SNP (rs1625579) of a micro-RNA, MIR-137, and found that carriers of the risk allele had lower scores for an OPCRIT-derived positive symptom factor and lower scores on a lifetime measure of psycho- sis incongruity. Risk allele carriers also had more cogni- tive deficits involving episodic memory and attentional control. The MIR-137 risk variant may be associated with a specific subgroup of psychosis patients. 3.5. Epigenetics Despite the promising results obtained with structural and functional genomic procedures to identify associa- tions with disease pathogenesis and potential drug targets in CNS disorders, it must be kept in mind that allelic mRNA expression is affected by genetic and epigenetic events, both with the potential to modulate neurotrans- mitter tone in the CNS [317]. Epigenetics is the study of how the environment can affect the genome of the indi- vidual during its development as well as the development of its descendants, all without changing the DNA se- quence, but inducing modifications in gene expression through DNA methylation-demethylation or through modification of histones by processes of methylation, deacetylation, and phosphorylation [318]. DNA methyla- tion is an epigenetic mechanism in which the methyl group is covalently coupled to the C5 position of the cytosine residue of CpG dinucleotides, generally leading to gene silencing. DNA methylation is catalyzed by a group of enzymes known as DNA methyltransferases (DNMT). DNA methylation changes only happen during DNA replication to maintain methylation patterns on hemimethylated DNA or establish new methylation. DNMT expression generally decreases after cell division, but significant levels of DNMTs are present in postmi- totic neurons. There is evidence that DNA methylation correlates with some neuropsychiatric disorders, influ- ences neural development, plasticity, learning, and memory, and is potentially reversible at certain genomic loci. This epigenetic mechanism of gene regulation gives support to a maintenance role of DNMT to prevent active demethylation in postmitotic neurons [319]. Genomic and epigenetic changes can affect complex cognitive functions, including learning and memory, and are causative in several developmental and psychiatric dis- orders affecting language, social functioning and IQ [320]. DNA methylation and histone deacetylation are two major epigenetic modifications that contribute to the stability of gene expression states. Perturbing DNA me- thylation, or disrupting the downstream response to DNA methylation-methyl-CpG-binding domain proteins (MBDs) and histone deacetylases (HDACs) by genetic or phar- macological means, has revealed a critical requirement for epigenetic regulation in brain development, learning, and mature nervous system stability, and has identified the first distinct gene sets that are epigenetically regu- lated within the nervous system [321]. Epigenetic mechanisms such as DNA methylation and modifications to histone proteins regulate high-order DNA structure and gene expression. Aberrant epigenetic mechanisms are involved in different CNS disorders (Rett syndrome, mental retardation disorders, alpha-tha- lassemia/mental retardation X-linked syndrome, Rubin- stein-Taybi syndrome, Coffin-Lowry syndrome, Alz- heimer’s disease, Parkinson’s disease, Huntington’s dis- ease, multiple sclerosis, epilepsy, amyotrophic lateral sclerosis) and also probably in mental disorders [322]. More than 150 post-translation modifications of his- tones have been reported, including methylations, acety- lations, ubiquitinations, SUMOylations and phosphoryla- tions. A macro-molecular complex, called ECREM for “Epigenetic Code REplication Machinery”, has been proposed as a potential mechanism involved in the in- heritance of the epigenetic code. The composition of ECREM may vary in a spatio-temporal manner accord- ing to the chromatin state, the cell phenotype and the development stage. Members of ECREM, responsible for the epigenetic code inheritance, include enzymes in- volved in DNA methylation and histone post-transla- tional modifications. Some of them, such as DNA me- thyltransferases (DNMTs), histone acetyltransferases (HATs) and histone deacetylases (HDACS, including sirtuins), have been found to be deregulated in several types of pathologies and are already targeted by inhibi- tors [323]. Epigenetic phenomena cannot be neglected in the pathogenesis and pharmacogenomics of CNS disorders. Studies in cancer research have demonstrated the anti- neoplastic effects of the DNA methylation inhibitor hy- dralazine and the histone deacetylase inhibitor valproic acid, of current use in epilepsy [324]. Novel effects of some pleiotropic drugs with activity on the CNS have to be explored to understand in full their mechanisms of action and adjust their dosages for new indications. Both hyper- and hypo-DNA methylation changes of the regu- latory regions play critical roles in defining the altered functionality of genes (MB-COMT, MAOA, DAT1, TH, DRD1, DRD2, RELN, BDNF) in major psychiatric dis- orders, such as SCZ and BD [325]. Histone deacetylases (HDACs), enzymes that affect the acetylation status of histones and other important cellular proteins, have been recognized as potentially useful therapeutic targets for a broad range of human disorders. Pharmacological manipulations using small- molecule HDAC inhibitors, which may restore trans- Copyright © 2013 SciRes. OPEN ACCESS
 R. Cacabelos et al. / Open Journal of Psychiatry 2 (2013) 46-139 113 criptional balance to neurons, modulate cytoskeletal function, affect immune responses and enhance protein degradation pathways, have been beneficial in various experimental models of brain diseases [326]. Recent advances in SCZ research indicate that the tel- encephalic gamma-aminobutyric acid (GABA)ergic neurotransmission deficit associated with this psychiatric disorder is probably mediated by the hypermethylation of the glutamic acid decarboxylase 67 (GAD67), reelin and other GABAergic promoters. A pharmacological strategy to reduce the hypermethylation of GABAergic promoters is to induce a DNA-cytosine demethylation by altering the chromatin remodeling with valproate (VPA). When co-administered with VPA, the clinical efficacy of atypi- cal antipsychotics is enhanced. VPA facilitates chromatin remodeling when it is associated with clozapine or sulpiride but not with haloperidol or olanzapine [327]. This remodeling might contribute to reelin- and GAD67- promoter demethylation and might reverse the GABAer- gic gene-expression downregulation associated with SCZ morbidity [328,329]. Reduction of prefrontal cortex glutamic acid decar- boxylase (GAD67) and reelin (mRNAs and proteins) expression is the most consistent finding reported by several studies of post-mortem SCZ brains. The reduced GAD67 and reelin expression in cortical GABAergic interneurons of SCZ brains is the consequence of an epigenetic hypermethylation of RELN and GAD67 pro- moters very likely mediated by the overexpression of DNA methyltransferase 1 in cortical GABAergic in- terneurons. RELN and GAD67 promoters express an in- creased recruitment of methyl-CpG binding domain pro- teins. The histone deacetylase inhibitor valproate, which increases acetylated histone content in cortical GABAer- gic interneurons, also prevents MET-induced RELN promoter hypermethylation and reduces the methyl-CpG binding domain protein binding to RELN and GAD67 promoters. DNA hypermethylation and the associated chromatin remodeling may be critically important in me- diating the epigenetic down-regulation of reelin and GAD67 expression detected in cortical GABAergic in- terneurons of SCZ patients [330,331]. Novel strategies in psychiatric epigenetics have been developed. With two novel approaches, it has been ob- served that valproic acid induced a 383% increase in GAD67 mRNA, an 89% increase in total acetylated his- tone 3 (H3K9, K14ac) levels, and a 482% increase in H3K9,K14ac attachment to the GAD67 promoter. Trichostatin A (TSA) induced comparable changes on all measures. Bipolar patients had significantly higher base- line levels of H3K9, K14ac compared to patients with SCZ. Subjects with clinically relevant serum levels of valproic acid (>65 μg/mL) showed a significant increase in mRNA expression. Separate approaches for examining chromatin remodeling in real clinical time provide evi- dence for differential epigenetic events in cultured lym- phocytes isolated from patients with SCZ and bipolar depression [332]. Li et al. [333] examined associations of structural mu- tability with germline DNA methylation and with non-allelic homologous recombination (NAHR) medi- ated by low-copy repeats (LCRs). Combined evidence from four human sperm methylome maps, human ge- nome evolution, structural polymorphisms in the human population, and previous genomic and disease studies consistently points to a strong association of germline hypomethylation and genomic instability. Methylation deserts, the ~1% fraction of the human genome with the lowest methylation in the germline, show a tenfold en- richment for structural rearrangements that occurred in the human genome since the branching of chimpanzee and are highly enriched for fast-evolving loci that regu- late tissue-specific gene expression. Analysis of copy number variants (CNVs) from 400 human samples indi- cates that association of structural mutability with germ- line hypomethylation is comparable in magnitude to the association of structural mutability with LCR-mediated NAHR. Rare CNVs occurring in the genomes of indi- viduals diagnosed with SCZ, bipolar disorder, and de- velopmental delay and de novo CNVs occurring in those diagnosed with autism are significantly more concen- trated within hypomethylated regions. 3.6. Mitochondrial DNA Mutations Mitochondria provide most of the energy for brain cells by the process of oxidative phosphorylation. Mito- chondrial oxidative phosphorylation is the major ATP- producing pathway, which supplies more than 95% of the total energy requirement in the cells. Damage to the mi- tochondrial electron transport chain has been suggested to be an important factor in the pathogenesis of a range of psychiatric disorders. Tissues with high energy de- mands, such as the brain, contain a large number of mi- tochondria, being therefore more susceptible to reduction of the aerobic metabolism. Mitochondrial abnormalities and deficiencies in oxidative phosphorylation have been reported in individuals with SCZ, BD, and major depres- sive disorder (MDD) in transcriptomic, proteomic, and metabolomic studies. The evidence includes impaired energy metabolism in the brain, detected using results of magnetic resonance spectroscopy, electron microscopy, co-morbidity with mitochondrial diseases, the effects of psychotropics on mitochondria, increased mitochondrial DNA (mtDNA) deletion in the brain, and association with mtDNA mutations/polymorphisms or nuclear-en- coded mitochondrial genes. Alterations of mitochondrial oxidative phosphorylation in SCZ have been reported in several brain regions and also in platelets. Abnormal mi- Copyright © 2013 SciRes. OPEN ACCESS
 R. Cacabelos et al. / Open Journal of Psychiatry 2 (2013) 46-139 114 tochondrial morphology, size and density have all been reported in the brains of schizophrenic individuals [334,335]. Several mutations in mtDNA sequence have been reported in SCZ and BD patients. The rate of syn- onymous base pair substitutions in the coding regions of the mtDNA genome is 22% higher in the dorsolateral prefrontal cortex of individuals with SCZ compared to controls [336]. Analyses of mitochondria-related genes using DNA microarray showed significantly increased LARS2 (mi- tochondrial leucyl-tRNA synthetase) in the post-mortem prefrontal cortices of patients with BD. LARS2 is a nu- clear gene encoding the enzyme catalyzing the aminoa- cylation of mitochondrial tRNA-Leu. A well-studied mi- tochondrial DNA point mutation, 3243A > G, in the re- gion of tRNA-LeuUUR, related with MELAS (mitochon- drial myopathy, encephalopathy, lactic acidosis, and stroke-like episodes), is known to decrease the efficiency of aminoacylation of tRNA-LeuUUR. The steady state level of LARS2 was examined in the transmitochondrial cybrids carrying 3243A > G. LARS2 was upregulated in the transmitochrondrial cybrids carrying 3243A > G. The 3243A > G was detected in the post-mortem brains of patients with BD and SCZ, who also showed higher lev- els of the mutation in their livers and significantly higher gene expression of LARS2. Upregulation of LARS2 is a hallmark of 324A > G mutation. The accumulation of 3243A > G mutation in the brain may have a pathophysi- ologic role in BD and SCZ [337]. A decreased expression of mitochondrial complex I subunit gene, NDUFV2 at 18p11, in lymphoblastoid cell lines (LCLs) from Japanese patients with BD, has been reported. No differences were found in NDUFV2 mRNA levels in LCLs of Caucasian BD patients compared with controls [338]. Park et al. [339] characterized Mitofilin, a mitochon- drial inner membrane protein, as a mediator of the mito- chondrial function of DISC1. A fraction of DISC1 was localized to the inside of mitochondria and directly in- teracts with Mitofilin. A reduction in DISC1 function induced mitochondrial dysfunction, evidenced by de- creased mitochondrial NADH dehydrogenase activities, reduced cellular ATP contents, and perturbed mitochon- drial Ca2+ dynamics. Deficiencies in DISC1 and Mitofilin induced a reduction in mitochondrial monoamine oxi- dase-A activity. The mitochondrial dysfunctions evoked by the deficiency of DISC1 were partially phenocopied by an overexpression of truncated DISC1 that is associ- ated with SCZ in humans. DISC1 deficiencies induced the ubiquitination of Mitofilin, suggesting that DISC1 is critical for the stability of Mitofilin. The mitochondrial dysfunction induced by DISC1 deficiency was partially reversed by coexpression of Mitofilin, confirming a functional link between DISC1 and Mitofilin for the normal mitochondrial function. DISC1 may play essen- tial roles for mitochondrial function in collaboration with the mitochondrial interacting partner Mitofilin. 3.7. Genotype-Phenotype Correlations, Transcriptomics, Proteomics, and Metabolomics Genotype-phenotype correlations represent a central is- sue in functional genomics to validate the impact of ge- nomic factors on disease pathogenesis and phenotypic expression of disease-related genes as well as in phar- macogenetics and pharmacogenomics [4]. Phenomics, the systematic study of phenotypes on a genome-wide scale, comprises a rate-limiting step on the road to ge- nomic discovery [340]. Novel strategies to assess geno- type-phenotype correlates have been developed. For in- stance, by using the parallel independent component analysis (para-ICA) of Liu to analyze a multimodal data set in which each subject was characterized on 24 dif- ferent SNP markers spanning multiple risk genes previ- ously associated with SCZ, Meda et al. [341] detected three fMRI components significantly correlated with two distinct gene components. The fMRI components, along with their significant genetic profile (dominant SNP) correlations were as follows: 1) Inferior frontal-anterior/ posterior cingulate-thalamus-caudate with SNPs from BDNF and DAT, 2) superior/middle temporal gyrus- cingulate-premotor with SLC6A4_PR and SLC6A4_PR_ AG (serotonin transporter promoter; 5HTTLPR), and 3) default mode-fronto-temporal gyrus with BDNF and DAT. These results reveal the effect/influence of specific interactions, between SCZ risk genes on imaging endo- phenotypes representing attention/working memory and goal-directed related brain function, thus establishing a useful methodology to probe multivariate genotype- phenotype relationships [341]. Bergen et al. [342] tested four genes [phenylalanine hydroxylase (PAH), the serotonin transporter (SLC6A4), monoamine oxidase B (MAOB), and the gamma-ami- nobutyric acid A receptor beta-3 subunit (GABRB3)] for their impact on five SCZ symptom factors: delusions, hallucinations, mania, depression, and negative symp- toms. The PAH 232 bp microsatellite allele demonstrated significant association with the delusions factor, and a significant association between the GABRB3 191 bp allele and the hallucinations factor was also detected [342]. Transcriptomics and gene expression studies are also helping to elucidate the role of the prefrontal cortex in SCZ and affective disorders. Owing to reciprocal con- nectivity, the thalamic nuclei and their cortical fields act as functional units. Chu et al. [343] screened the expres- sion of the entire human genome of neurons harvested by laser-capture microdissection (LCM) from the thalamic Copyright © 2013 SciRes. OPEN ACCESS
 R. Cacabelos et al. / Open Journal of Psychiatry 2 (2013) 46-139 115 primary relay to dorsolateral prefrontal cortex in three psychiatric disease states as compared with controls. Microarray analysis of gene expression showed the larg- est number of dysregulated genes in SCZ, followed by major depression and D8, respectively. Significantly, IGF1-mTOR-, AKT-, RAS-, VEGF-, Wnt- and immune- related signaling, eIF2- and proteasome-related genes were unique to SCZ. Vitamin D receptor and calcium signaling pathway were unique to BD. AKAP95 pathway and pantothenate and CoA biosynthesis were unique to major depression [343]. Studies on the human CNS transcriptome suggest changes in pro-inflammatory pathways and myelination in SCZ, whereas changes in the proteome suggest that pathways involved in energy and metabolism may be particularly stressed. There appear to be complex changes in the expression of proposed candidate genes for SCZ such as NRG1, DISC1, RGS4 and DTNB1, and there are continued reports of alterations in central gamma-aminobutyric acidergic, dopaminergic, glutama- tergic and cholinergic pathways in patients with the dis- order. Data on epigenetic mechanisms and transcriptome regulation suggest that at least some changes in gene expression may be due to changes in levels of gene pro- moter methylation or microRNAs in the CNS of patients with SCZ [344]. SCZ is likely to be a consequence of DNA alterations that, together with environmental factors, will lead to protein expression differences and the ultimate establish- ment of the illness. The superior temporal gyrus is im- plicated in SCZ and executes functions such as the proc- essing of speech, language skills and sound processing. Proteomics studies in the left posterior superior temporal gyrus (Wernicke’s area - BA22p) revealed 11 downregu- lated and 14 upregulated proteins, most of them related to energy metabolism. Whereas many of the identified proteins have been previously implicated in SCZ, such as fructose-bisphosphate aldolase C, creatine kinase and neuron-specific enolase, new putative disease markers were also identified such as dihydrolipoyl dehydrogenase, tropomyosin 3, breast cancer metastasis-suppressor 1, heterogeneous nuclear ribonucleoproteins C1/C2 and phosphate carrier protein, mitochondrial precursor [345]. Genome-wide expression analysis of peripheral blood identified candidate biomarkers for SCZ. Using Affy- metrix micoarrays, Kuzman et al. [346] identified sig- nificantly altered expression of 180 gene probes in psy- chotic patients compared to controls. The following genes were significantly altered in patients: glucose transporter, SLC2A3 and actin assembly factor DAAM2 were increased, whereas translation, zinc metallopepti- dase, neurolysin 1 and myosin C were significantly de- creased. DAAM2 polymorphic variants have been found significantly associated with SCZ [346]. The repertoire of biochemicals present in cells, tissue, and body fluids is known as the metabolome. State of the art metabolomic analytical platforms and informatics tools are being used to map potential biomarkers for CNS disorders. Early findings from metabolomic studies may help to identify promising biomarkers for SCZ and many other neuropsychiatric disorders [347]. 4. PHARMACOGENOMICS OF ANTIPSYCHOTIC DRUGS 4.1. Aripiprazole Aripiprazole is an arylpiperazine atypical antipsychotic with full agonist activity for 5-HT1A, 5-HT1B, 5-HT1D, 5-HT6, 5-HT receptors, acting also as a partial agonist of D2 and 5-HT1A receptors and antagonist of the 5-HT2A receptor. Aripiprazole is a major substrate of CYP2D6 and CYP3A4. Caution and personalized dose adjustment should be made in patients with the following genotypes: ADRA1A (Arg347Cys), CYP2D6 (CYP2D6*3, CYP2D6*4, CYP2D6*5, CYP2D6*6, CYP2D6*7, CYP2D6*8, CYP2D6*10, CYP2D6*17, CYP2D6*1xN), CYP3A4 and CYP3A5 (CYP3A4*1, CYP3A4*1B, CYP3A4*2, CYP3A4*3, CYP3A4*4, CYP3A4*5, CYP3A4*6, CYP3A4*8, CYP3A4*11, CYP3A4*12, CYP3A4*13, CYP3A4*15, CYP3A4*17, CYP3A4*18, CYP3A4*19, CYP3A5*3), DRD2 (TaqIA RFLP (rs1800497, ANKK1), TaqIB (rs1079597), rs6277), DRD3 (Ser9Gly), HTR2A (His452Tyr, His368Tyr, Ile197Val, Ile113Val, Ala447Val, Ala363Val, Thr25Asn, A-1438G (rs6311), T102C (rs6313)), HTR2C (Cys23Ser, −759C/T), and also in ABCB1 and HTR1A mutants [25] (Table 2). 4.2. Benperidol Benperidol is a butyrophenone antipsychotic which blocks postsynaptic mesolimbic dopaminergic D1 and D2 recep- tors. Genes potentially involved in efficacy and safety might be DRD1 and DRD2 [25] (Table 2). 4.3. Bromperidol Bromperidol is a butyrophenone with antagonistic ac- tivity on D2 receptors and a moderate antagonistic activ- ity on serotonin 5-HT2 receptors. Bromperidol is a major substrate of CYP3A4, a minor substrate of CYP2D6, a substrate of several UGTs, and a moderate inhibitor of CYP2D6. ABCB1 (C3435T and G2677T/A), DRD2 (Taq IA RFLP and rs1800497, ANKK1), and HTR2 vari- ants may influence its pharmacokinetics and pharmaco- dynamics [25] (Table 2). 4.4. Chlorpromazine Chlorpromazine is an aliphatic phenothiazine antipsy- Copyright © 2013 SciRes. OPEN ACCESS
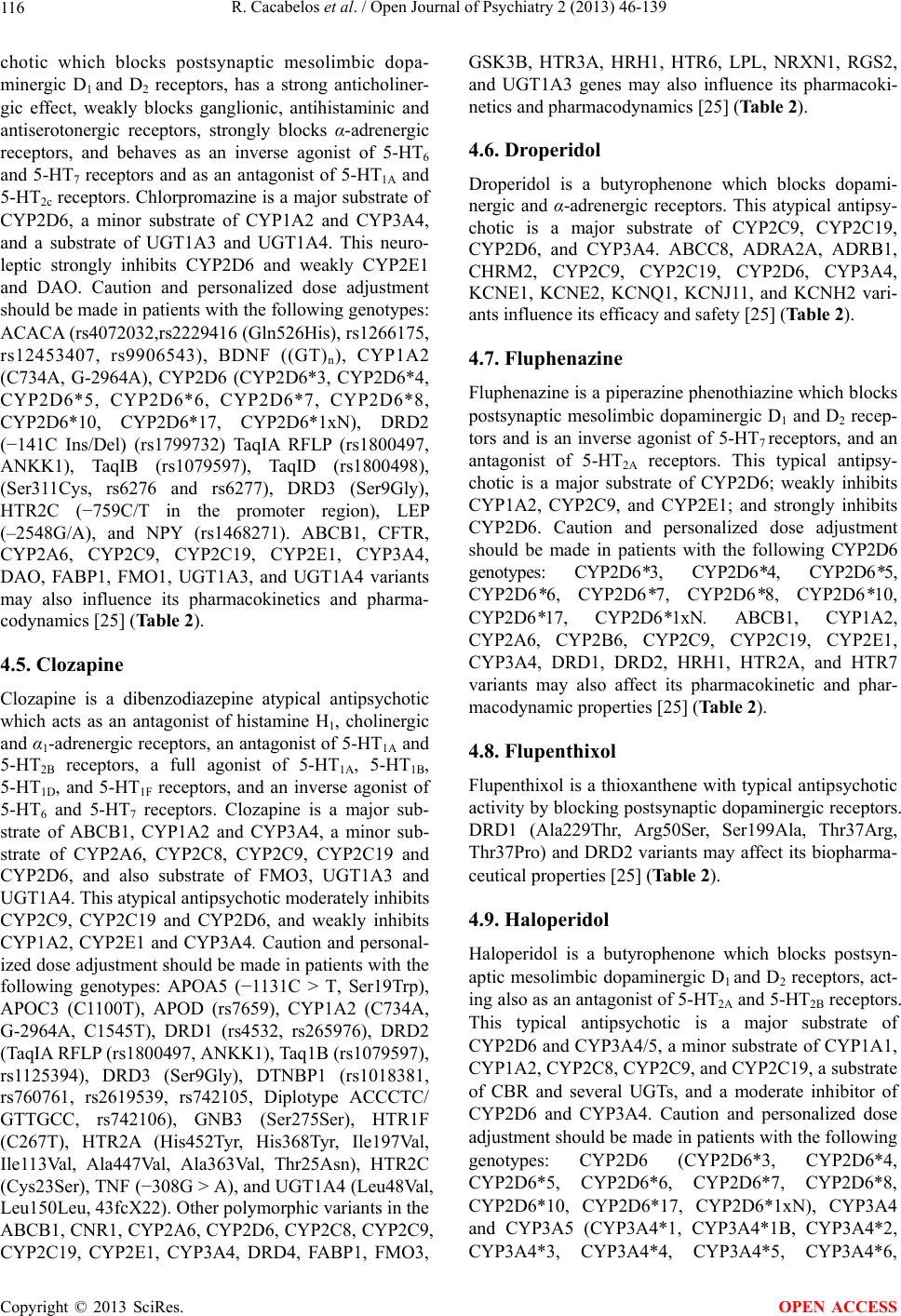 R. Cacabelos et al. / Open Journal of Psychiatry 2 (2013) 46-139 116 chotic which blocks postsynaptic mesolimbic dopa- minergic D1 and D2 receptors, has a strong anticholiner- gic effect, weakly blocks ganglionic, antihistaminic and antiserotonergic receptors, strongly blocks α-adrenergic receptors, and behaves as an inverse agonist of 5-HT6 and 5-HT7 receptors and as an antagonist of 5-HT1A and 5-HT2c receptors. Chlorpromazine is a major substrate of CYP2D6, a minor substrate of CYP1A2 and CYP3A4, and a substrate of UGT1A3 and UGT1A4. This neuro- leptic strongly inhibits CYP2D6 and weakly CYP2E1 and DAO. Caution and personalized dose adjustment should be made in patients with the following genotypes: ACACA (rs4072032,rs2229416 (Gln526His), rs1266175, rs12453407, rs9906543), BDNF ((GT)n), CYP1A2 (C734A, G-2964A), CYP2D6 (CYP2D6*3, CYP2D6*4, CYP2D6*5, CYP2D6*6, CYP2D6*7, CYP2D6*8, CYP2D6*10, CYP2D6*17, CYP2D6*1xN), DRD2 (−141C Ins/Del) (rs1799732) TaqIA RFLP (rs1800497, ANKK1), TaqIB (rs1079597), TaqID (rs1800498), (Ser311Cys, rs6276 and rs6277), DRD3 (Ser9Gly), HTR2C (−759C/T in the promoter region), LEP (–2548G/A), and NPY (rs1468271). ABCB1, CFTR, CYP2A6, CYP2C9, CYP2C19, CYP2E1, CYP3A4, DAO, FABP1, FMO1, UGT1A3, and UGT1A4 variants may also influence its pharmacokinetics and pharma- codynamics [25] (Table 2). 4.5. Clozapine Clozapine is a dibenzodiazepine atypical antipsychotic which acts as an antagonist of histamine H1, cholinergic and α1-adrenergic receptors, an antagonist of 5-HT1A and 5-HT2B receptors, a full agonist of 5-HT1A, 5-HT1B, 5-HT1D, and 5-HT1F receptors, and an inverse agonist of 5-HT6 and 5-HT7 receptors. Clozapine is a major sub- strate of ABCB1, CYP1A2 and CYP3A4, a minor sub- strate of CYP2A6, CYP2C8, CYP2C9, CYP2C19 and CYP2D6, and also substrate of FMO3, UGT1A3 and UGT1A4. This atypical antipsychotic moderately inhibits CYP2C9, CYP2C19 and CYP2D6, and weakly inhibits CYP1A2, CYP2E1 and CYP3A4. Caution and personal- ized dose adjustment should be made in patients with the following genotypes: APOA5 (−1131C > T, Ser19Trp), APOC3 (C1100T), APOD (rs7659), CYP1A2 (C734A, G-2964A, C1545T), DRD1 (rs4532, rs265976), DRD2 (TaqIA RFLP (rs1800497, ANKK1), Taq1B (rs1079597), rs1125394), DRD3 (Ser9Gly), DTNBP1 (rs1018381, rs760761, rs2619539, rs742105, Diplotype ACCCTC/ GTTGCC, rs742106), GNB3 (Ser275Ser), HTR1F (C267T), HTR2A (His452Tyr, His368Tyr, Ile197Val, Ile113Val, Ala447Val, Ala363Val, Thr25Asn), HTR2C (Cys23Ser), TNF (−308G > A), and UGT1A4 (Leu48Val, Leu150Leu, 43fcX22). Other polymorphic variants in the ABCB1, CNR1, CYP2A6, CYP2D6, CYP2C8, CYP2C9, CYP2C19, CYP2E1, CYP3A4, DRD4, FABP1, FMO3, GSK3B, HTR3A, HRH1, HTR6, LPL, NRXN1, RGS2, and UGT1A3 genes may also influence its pharmacoki- netics and pharmacodynamics [25] (Table 2). 4.6. Droperidol Droperidol is a butyrophenone which blocks dopami- nergic and α-adrenergic receptors. This atypical antipsy- chotic is a major substrate of CYP2C9, CYP2C19, CYP2D6, and CYP3A4. ABCC8, ADRA2A, ADRB1, CHRM2, CYP2C9, CYP2C19, CYP2D6, CYP3A4, KCNE1, KCNE2, KCNQ1, KCNJ11, and KCNH2 vari- ants influence its efficacy and safety [25] (Table 2). 4.7. Fluphenazine Fluphenazine is a piperazine phenothiazine which blocks postsynaptic mesolimbic dopaminergic D1 and D2 recep- tors and is an inverse agonist of 5-HT7 receptors, and an antagonist of 5-HT2A receptors. This typical antipsy- chotic is a major substrate of CYP2D6; weakly inhibits CYP1A2, CYP2C9, and CYP2E1; and strongly inhibits CYP2D6. Caution and personalized dose adjustment should be made in patients with the following CYP2D6 genotypes: CYP2D6*3, CYP2D6*4, CYP2D6*5, CYP2D6*6, CYP2D6*7, CYP2D6*8, CYP2D6*10, CYP2D6*17, CYP2D6*1xN. ABCB1, CYP1A2, CYP2A6, CYP2B6, CYP2C9, CYP2C19, CYP2E1, CYP3A4, DRD1, DRD2, HRH1, HTR2A, and HTR7 variants may also affect its pharmacokinetic and phar- macodynamic properties [25] (Table 2). 4.8. Flupenthixol Flupenthixol is a thioxanthene with typical antipsychotic activity by blocking postsynaptic dopaminergic receptors. DRD1 (Ala229Thr, Arg50Ser, Ser199Ala, Thr37Arg, Thr37Pro) and DRD2 variants may affect its biopharma- ceutical properties [25] (Table 2). 4.9. Haloperidol Haloperidol is a butyrophenone which blocks postsyn- aptic mesolimbic dopaminergic D1 and D2 receptors, act- ing also as an antagonist of 5-HT2A and 5-HT2B receptors. This typical antipsychotic is a major substrate of CYP2D6 and CYP3A4/5, a minor substrate of CYP1A1, CYP1A2, CYP2C8, CYP2C9, and CYP2C19, a substrate of CBR and several UGTs, and a moderate inhibitor of CYP2D6 and CYP3A4. Caution and personalized dose adjustment should be made in patients with the following genotypes: CYP2D6 (CYP2D6*3, CYP2D6*4, CYP2D6*5, CYP2D6*6, CYP2D6*7, CYP2D6*8, CYP2D6*10, CYP2D6*17, CYP2D6*1xN), CYP3A4 and CYP3A5 (CYP3A4*1, CYP3A4*1B, CYP3A4*2, CYP3A4*3, CYP3A4*4, CYP3A4*5, CYP3A4*6, Copyright © 2013 SciRes. OPEN ACCESS
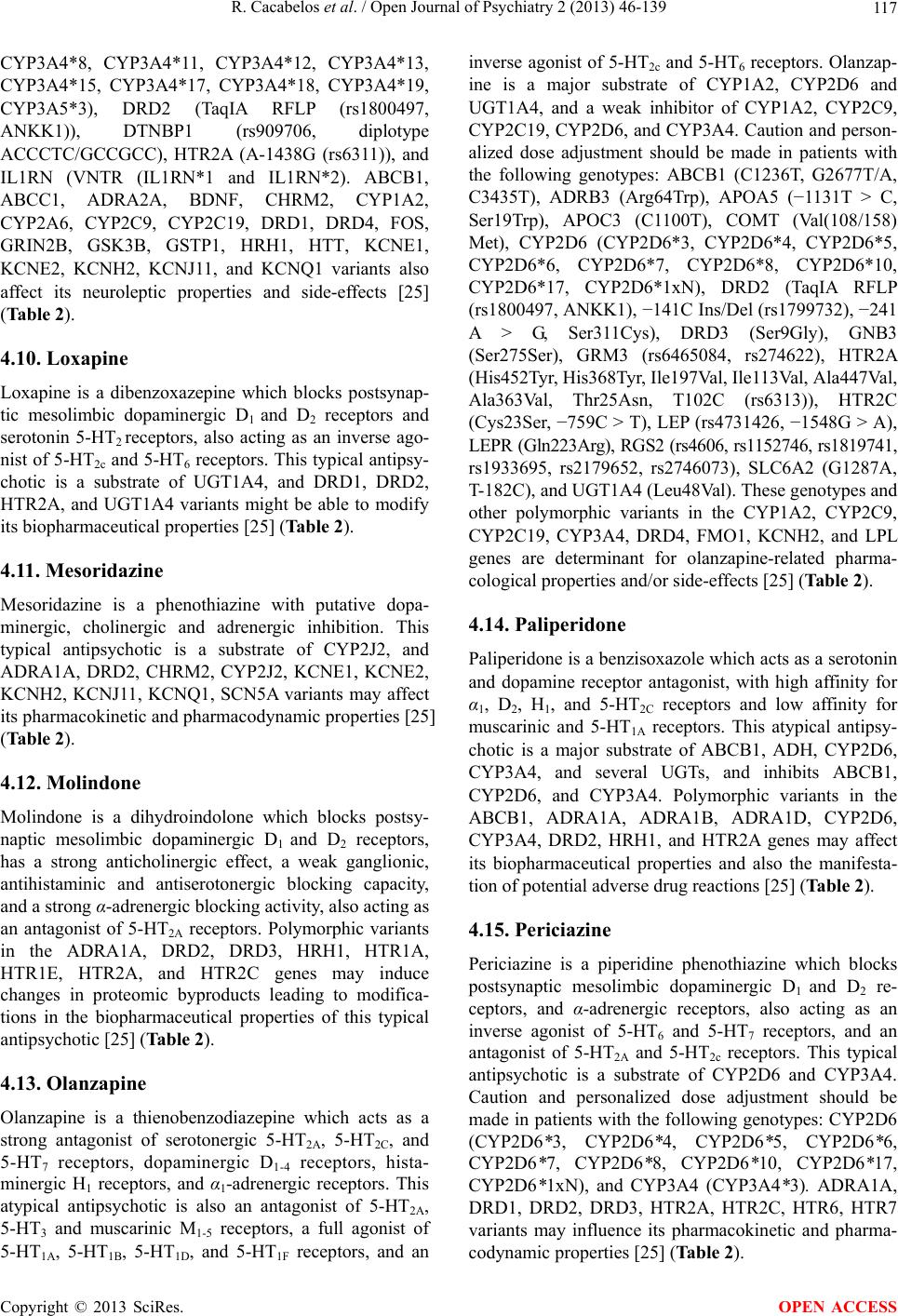 R. Cacabelos et al. / Open Journal of Psychiatry 2 (2013) 46-139 117 CYP3A4*8, CYP3A4*11, CYP3A4*12, CYP3A4*13, CYP3A4*15, CYP3A4*17, CYP3A4*18, CYP3A4*19, CYP3A5*3), DRD2 (TaqIA RFLP (rs1800497, ANKK1)), DTNBP1 (rs909706, diplotype ACCCTC/GCCGCC), HTR2A (A-1438G (rs6311)), and IL1RN (VNTR (IL1RN*1 and IL1RN*2). ABCB1, ABCC1, ADRA2A, BDNF, CHRM2, CYP1A2, CYP2A6, CYP2C9, CYP2C19, DRD1, DRD4, FOS, GRIN2B, GSK3B, GSTP1, HRH1, HTT, KCNE1, KCNE2, KCNH2, KCNJ11, and KCNQ1 variants also affect its neuroleptic properties and side-effects [25] (Table 2). 4.10. Loxapine Loxapine is a dibenzoxazepine which blocks postsynap- tic mesolimbic dopaminergic D1 and D2 receptors and serotonin 5-HT2 receptors, also acting as an inverse ago- nist of 5-HT2c and 5-HT6 receptors. This typical antipsy- chotic is a substrate of UGT1A4, and DRD1, DRD2, HTR2A, and UGT1A4 variants might be able to modify its biopharmaceutical properties [25] (Table 2). 4.11. Mesoridazine Mesoridazine is a phenothiazine with putative dopa- minergic, cholinergic and adrenergic inhibition. This typical antipsychotic is a substrate of CYP2J2, and ADRA1A, DRD2, CHRM2, CYP2J2, KCNE1, KCNE2, KCNH2, KCNJ11, KCNQ1, SCN5A variants may affect its pharmacokinetic and pharmacodynamic properties [25] (Table 2). 4.12. Molindone Molindone is a dihydroindolone which blocks postsy- naptic mesolimbic dopaminergic D1 and D2 receptors, has a strong anticholinergic effect, a weak ganglionic, antihistaminic and antiserotonergic blocking capacity, and a strong α-adrenergic blocking activity, also acting as an antagonist of 5-HT2A receptors. Polymorphic variants in the ADRA1A, DRD2, DRD3, HRH1, HTR1A, HTR1E, HTR2A, and HTR2C genes may induce changes in proteomic byproducts leading to modifica- tions in the biopharmaceutical properties of this typical antipsychotic [25] (Table 2). 4.13. Olanzapine Olanzapine is a thienobenzodiazepine which acts as a strong antagonist of serotonergic 5-HT2A, 5-HT2C, and 5-HT7 receptors, dopaminergic D1-4 receptors, hista- minergic H1 receptors, and α1-adrenergic receptors. This atypical antipsychotic is also an antagonist of 5-HT2A, 5-HT3 and muscarinic M1-5 receptors, a full agonist of 5-HT1A, 5-HT1B, 5-HT1D, and 5-HT1F receptors, and an inverse agonist of 5-HT2c and 5-HT6 receptors. Olanzap- ine is a major substrate of CYP1A2, CYP2D6 and UGT1A4, and a weak inhibitor of CYP1A2, CYP2C9, CYP2C19, CYP2D6, and CYP3A4. Caution and person- alized dose adjustment should be made in patients with the following genotypes: ABCB1 (C1236T, G2677T/A, C3435T), ADRB3 (Arg64Trp), APOA5 (−1131T > C, Ser19Trp), APOC3 (C1100T), COMT (Val(108/158) Met), CYP2D6 (CYP2D6*3, CYP2D6*4, CYP2D6*5, CYP2D6*6, CYP2D6*7, CYP2D6*8, CYP2D6*10, CYP2D6*17, CYP2D6*1xN), DRD2 (TaqIA RFLP (rs1800497, ANKK1), −141C Ins/Del (rs1799732), −241 A > G, Ser311Cys), DRD3 (Ser9Gly), GNB3 (Ser275Ser), GRM3 (rs6465084, rs274622), HTR2A (His452Tyr, His368Tyr, Ile197Val, Ile113Val, Ala447Val, Ala363Val, Thr25Asn, T102C (rs6313)), HTR2C (Cys23Ser, −759C > T), LEP (rs4731426, −1548G > A), LEPR (Gln223Arg), RGS2 (rs4606, rs1152746, rs1819741, rs1933695, rs2179652, rs2746073), SLC6A2 (G1287A, T-182C), and UGT1A4 (Leu48Val). These genotypes and other polymorphic variants in the CYP1A2, CYP2C9, CYP2C19, CYP3A4, DRD4, FMO1, KCNH2, and LPL genes are determinant for olanzapine-related pharma- cological properties and/or side-effects [25] (Table 2). 4.14. Paliperidone Paliperidone is a benzisoxazole which acts as a serotonin and dopamine receptor antagonist, with high affinity for α1, D2, H1, and 5-HT2C receptors and low affinity for muscarinic and 5-HT1A receptors. This atypical antipsy- chotic is a major substrate of ABCB1, ADH, CYP2D6, CYP3A4, and several UGTs, and inhibits ABCB1, CYP2D6, and CYP3A4. Polymorphic variants in the ABCB1, ADRA1A, ADRA1B, ADRA1D, CYP2D6, CYP3A4, DRD2, HRH1, and HTR2A genes may affect its biopharmaceutical properties and also the manifesta- tion of potential adverse drug reactions [25] (Table 2). 4.15. Periciazine Periciazine is a piperidine phenothiazine which blocks postsynaptic mesolimbic dopaminergic D1 and D2 re- ceptors, and α-adrenergic receptors, also acting as an inverse agonist of 5-HT6 and 5-HT7 receptors, and an antagonist of 5-HT2A and 5-HT2c receptors. This typical antipsychotic is a substrate of CYP2D6 and CYP3A4. Caution and personalized dose adjustment should be made in patients with the following genotypes: CYP2D6 (CYP2D6*3, CYP2D6*4, CYP2D6*5, CYP2D6*6, CYP2D6*7, CYP2D6*8, CYP2D6*10, CYP2D6*17, CYP2D6*1xN), and CYP3A4 (CYP3A4*3). ADRA1A, DRD1, DRD2, DRD3, HTR2A, HTR2C, HTR6, HTR7 variants may influence its pharmacokinetic and pharma- codynamic properties [25] (Table 2). Copyright © 2013 SciRes. OPEN ACCESS
 R. Cacabelos et al. / Open Journal of Psychiatry 2 (2013) 46-139 118 4.16. Perphenazine Perphenazine is a piperazine phenothiazine which blocks postsynaptic mesolimbic dopaminergic D1 and D2 recep- tors. This typical antipsychotic is a major substrate of CYP1A2, CYP2C8, CYP2C9, CYP2C18, CYP2C19, CYP2D6, and CYP3A4/5, and a weak inhibitor of CYP1A2 and CYP2D6. Caution and personalized dose adjustment should be made in patients with the following genotypes: ADRA1A (Arg347Cys), CYP2D6 (CYP2D6*3, CYP2D6*4, CYP2D6*5, CYP2D6*6, CYP2D6*7, CYP2D6*8, CYP2D6*10, CYP2D6*17), and DRD2 (−141C Ins/Del (rs1799732), TaqlA (rs1800497), TaqIB (rs1079597), rs1125394). ABCB1, CYP1A2, CYP2C9, CYP2C19, CYP3A4, DRD1, and RGS4 variants may affect its biopharmaceutical properties [25] (Table 2). 4.17. Pimozide Pimozide is a diphenylbutylpiperidine with antagonistic activity on dopaminergic receptors and 5-HT1A, 5-HT2A and 5-HT7 receptors. This typical antipsychotic is a ma- jor substrate of CYP3A4, a minor substrate of CYP1A2, a weak inhibitor of CYP2C19 and CYP2E1, a moderate inhibitor of CYP3A4, and a strong inhibitor of CYP2D6. Caution and personalized dose adjustment should be made in patients with the following genotypes: ADRA1A (Arg347Cys), CYP1A2 (C734A, G-2964A), CYP2D6 (CYP2D6*3, CYP2D6*4, CYP2D6*5, CYP2D6*6, CYP2D6*7, CYP2D6*8, CYP2D6*10, CYP2D6*17, CYP2D6*1xN), CYP3A4 (CYP3A4*3), KCNE2 (Gln9Glu, Met54Thr,Thr8Ala), KCNH2 (Arg784Trp), KCNQ1 (Arg583Cys), and SCN5A (Gly615Glu, Leu618Phe, Phe1250Leu, Leu1825Pro). Other genes potentially involved in its metabolism and pharmacological properties are ABCB1, CHRM2, CYP2C19, CYP2E1, DRD2, KCNE1, and KCNJ11 [25] (Table 2). 4.18. Pipotiazine Pipotiazine is a piperidine phenothiazine which blocks postsynaptic mesolimbic dopaminergic D1 and D2 recep- tors. This typical antipsychotic is a substrate of CYP2D6 and CYP3A4. Different polymorphic variants in the CYP2D6, CYP3A4, and DRD genes may affect its me- tabolism and pharmacological properties [25] (Table 2). 4.19. Prochlorperazine Prochlorperazine is a piperazine phenothiazine which strongly blocks postsynaptic mesolimbic dopaminergic D1 and D2 receptors, α-adrenergic receptors and cho- linergic receptors. The metabolism and pharmacologi- cal properties of this typical antipsychotic may be af- fected by polymorphic variants in the ABCB1, ADRA1A, CYPs, DRD1, and DRD2 genes, as well as other genes associated with histaminergic and cholinergic neuro- transmission [25] (Table 2). 4.20. Quetiapine Quetiapine is a dibenzothiazepine which acts as a sero- tonergic (5-HT1A, 5-HT2A, 5-HT2), dopaminergic (D1 and D2), histaminergic H1, and adrenergic (α1- and α2-) re- ceptor antagonist, and a full agonist of 5-HT1A, 5-HT1D, 5-HT1F, and 5-HT2A receptors. This atypical antipsy- chotic is a minor substrate of CYP2D6 and a major sub- strate of CYP3A4/5. Caution and personalized dose ad- justment should be made in patients with the following genotypes: ADRA1A (Arg347Cys), CYP3A4 (CYP3A4*3), HTR2A (His452Tyr, His368Tyr, Ile197Val, Ile113Val, Ala447Val, Ala363Val, Thr25Asn), KCNE2 (Gln9Glu, Met54Thr, Thr8Ala), KCNH2 (Arg784Trp), KCNQ1 (Arg583Cys), and SCN5A (Gly615Glu, Leu618Phe, Phe1250Leu, Leu1825Pro). These geno- types and other polymorphisms in the ABCB1, CYP2D6, DRD1, DRD2, DRD4, HRH1, HTR1A, HTR2B, KCNE1, and RGS4 genes are responsible for the me- tabolism and biopharmaceutical properties of quetiapine [25] (Table 2). 4.21. Risperidone Risperidone is a benzisoxazole with serotonergic, dopa- minergic, α1-, α2-adrenergic and histaminergic receptor antagonist activity, low-moderate affinity for 5-HT1C, 5-HT1D, and 5-HT1A receptors, low affinity for D1 recep- tors, and inverse agonist activity on 5-HT2c, 5-HT6, and 5-HT7 receptors. This atypical antipsychotic is a major substrate of ABCB1 and CYP2D6, a minor substrate of CYP3A4/5, and weakly inhibits ABCB1, CYP2D6, and CYP3A4. Caution and personalized dose adjustment should be made in patients with the following genotypes: ABCB1 (C1236T, G2677T, C3435T), COMT (rs4633, rs4680, rs737865, rs6269, rs4818, rs165599), CYP2D6 (CYP2D6*3, CYP2D6*4, CYP2D6*5, CYP2D6*6, CYP2D6*7, CYP2D6*8, CYP2D6*10, CYP2D6*17), DRD2 (TaqIA (rs1800497), −141C Ins/Del (rs1799732), A-241G), DRD3 (Gly9Ser (rs6280), rs167771), GRM3 (rs724226), HTR2A (His452Tyr, His368Tyr, Ile197Val, Ala447Val, Ala363Val, Thr25Asn, 102-T/C (rs6313)), HTR3A (rs1176713), and HTR6 (T267C). These geno- types and other variants in the ADRA1A, ADRA1B, APOA5, CYP3A4/5, DRD1, DRD4, FOS, HTR2C, KCNH2, RGS4, and SLC6A4 genes are responsible for the metabolism, pharmacological effects, and adverse drug events associated with risperidone administration to psychotic patients [25] (Table 2). 4.22. Sulpiride Sulpiride is a benzamide with postsynaptic D2 antagonist Copyright © 2013 SciRes. OPEN ACCESS
 R. Cacabelos et al. / Open Journal of Psychiatry 2 (2013) 46-139 119 activity. This atypical antipsychotic is a substrate of CYP2D6, and polymorphisms in the CYP2D6 and DRD2 genes influence its metabolism and pharmacological properties [25] (Table 2). 4.23. Thioridazine Thioridazine is a phenothiazine which blocks postsynap- tic mesolimbic dopaminergic receptors and α-adrenergic receptors, also acting as an inverse agonist of 5-HT6 and 5-HT7 receptors, and as an antagonist of 5-HT2c receptors. This typical antipsychotic is a major substrate of CYP1A2, CYP2D6, CYP2J2, and CYP3A4, a minor substrate of CYP2C19, a weak inhibitor of CYP1A2, CYP2C9, CYP2E1, and DRD1, a moderate inhibitor of CYP2D6, and a strong blocker of ADRA1s, ADRA2s, and ADRBs. Caution and personalized dose adjustment should be made in patients with the following genotypes: ADRA1A (Arg347Cys), CYP2D6 (CYP2D6*3, CYP2D6*4, CYP2D6*5, CYP2D6*6, CYP2D6*7, CYP2D6*8, CYP2D6*10, CYP2D6*17), and KCNE2 (Gln9Glu, Met54Thr, Thr8Ala). Other genes that may be involved in thioridazine metabolism and pharmacologi- cal effects include ABCB1, CHRM2, CYP1A2, CYP2A6, CYP2C9, CYP2C19, CYP2E1, CYP2J2, CYP3A4, DRD2, FABP1, HRH1, KCNE1, KCNH2, KCNQ1, and KCNJ11 [25] (Table 2). 4.24. Thiothixene Thiothixene is a thioxanthene which inhibits dopamine receptors, blocks α-adrenergic receptors, and is an an- tagonist of 5-HT2a receptors. This typical antipsychotic is a major substrate of CYP1A2 and a weak inhibitor of CYP2D6. Caution and personalized dose adjustment should be made in patients with the following genotypes: ADRA1A (Arg347Cys), CYP1A2 (C734A, G-2964A), KCNE2 (Gln9Glu, Met54Thr, Thr8Ala), KCNQ1 (Arg583Cys), KCNH6 (Arg784Trp), and SCN5A (Gly615Glu, Leu618Phe, Phe1250Leu, Leu1825Pro). Other genes potentially involved in thiothixene metabo- lism and pharmacological effects are CYP2D6, DRD2, and KCNE1 [25] (Table 2). 4.25. Trifluoperazine Trifluoperazine is a phenothiazine which blocks post- synaptic mesolimbic dopaminergic receptors and α-ad- renergic receptors. This typical antipsychotic is a major substrate of CYP1A2 and UGT1A4. Caution and per- sonalized dose adjustment should be made in patients with the following genotypes: ADRA1A (Arg347Cys), and CYP1A2 (C734A, G-2964A). Some other genes (ABCB1, DRD2, IL12B, UGT1A4) may affect trifluop- erazine pharmacokinetics and pharmacodynamics [25] (Table 2). 4.26. Ziprasidone Ziprasidone is a benzylisothiazolylpiperazine with high affinity for D2, D3, 5-HT2A, 5-HT1A, 5-HT2C, 5-HT1D and α1-adrenergic receptors; moderate affinity for histamine H1 receptors; antagonist of D2, 5-HT1A, 5-HT2A, and 5-HT1D receptors; full agonist of 5-HT1B and 5-HT1D receptors; partial agonist of 5-HT1A receptors; and in- verse agonist of 5-HT2c and 5-HT7 receptors. This atypi- cal antipsychotic is a major substrate of CYP3A4, a mi- nor substrate of CYP1A2, a substrate of AOXs and HTR1A, and an inhibitor of CYP2D6, CYP3A4, HTR2A, and DRD2. Caution and personalized dose adjustment should be made in in patients with the following geno- types: CYP3A4 (CYP3A4*1, CYP3A4*1B, CYP3A4*2, CYP3A4*3, CYP3A4*4, CYP3A4*5, CYP3A4*6, CYP3A4*8, CYP3A4*11, CYP3A4*12, CYP3A4*13, CYP3A4*15, CYP3A4*17, CYP3A4*18, CYP3A4*19), HTR2A (His452Tyr, His368Tyr, Ile197Val, Ile113Val, Ala447Val, Ala363Val, Thr25Asn), KCNH2 (Tyr652Ala, Phe656Ala), and RGS4 (rs951439, rs2661319, rs2842030). Other genes involved in ziprasidone pharmacokinetics and pharmacodynamics include AOX1, CYP1A2, CYP2D6, DRD2, DRD4, HTR1A, HTR2A, HRH1, and KCNH2 [25] (Table 2). 4.27. Zuclopenthixol Zuclopenthixol is a thioxanthene which blocks post- synaptic mesolimbic dopaminergic receptors. This typi- cal antipsychotic is a major substrate of CYP2D6; con- sequently, caution and personalized dose adjustment should be made in patients with the following CYP2D6 variants: CYP2D6*3, CYP2D6*4, CYP2D6*5, CYP2D6*6, CYP2D6*7, CYP2D6*8, CYP2D6*10, CYP2D6*17, CYP2D6*1xN. Other genes to take into account in the pharmacokinetics and pharmacodynamics of zuclopen- thixol are ADRA1A, DRD1, DRD2, KCNE2, and SCN5A [25] (Table 2). 5. FUTURE DIRECTIONS Historically, the vast majority of pharmacogenetic stud- ies of CNS disorders have been addressed to evaluate the impact of cytochrome P450 enzymes on drug metabo- lism, and conventional targets for psychotropic drugs were dopamine, serotonin, noradrenaline, GABA, ion channels, acetylcholine and their respective biosynthetic and catalyzing enzymes, receptors and transporters; however, in the past few years many different genes have been associated with both pathogenesis and pharmaco- genomics of neuropsychiatric disorders [1,2,5,6,17]. Some of these genes and their products constitute poten- tial targets for future treatments. New developments in genomics, including whole genome genotyping ap- proaches and comprehensive information on genomic Copyright © 2013 SciRes. OPEN ACCESS
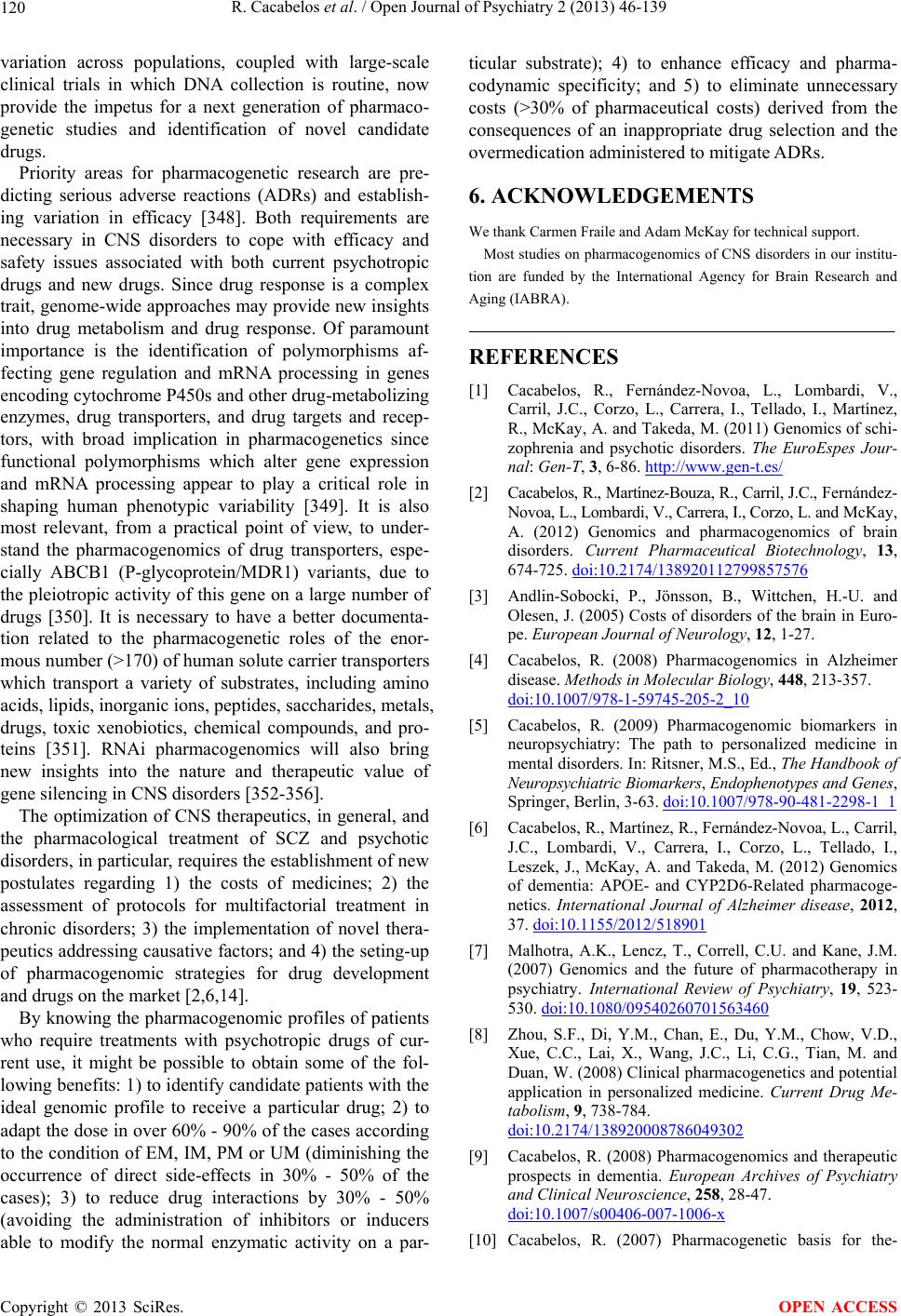 R. Cacabelos et al. / Open Journal of Psychiatry 2 (2013) 46-139 120 variation across populations, coupled with large-scale clinical trials in which DNA collection is routine, now provide the impetus for a next generation of pharmaco- genetic studies and identification of novel candidate drugs. Priority areas for pharmacogenetic research are pre- dicting serious adverse reactions (ADRs) and establish- ing variation in efficacy [348]. Both requirements are necessary in CNS disorders to cope with efficacy and safety issues associated with both current psychotropic drugs and new drugs. Since drug response is a complex trait, genome-wide approaches may provide new insights into drug metabolism and drug response. Of paramount importance is the identification of polymorphisms af- fecting gene regulation and mRNA processing in genes encoding cytochrome P450s and other drug-metabolizing enzymes, drug transporters, and drug targets and recep- tors, with broad implication in pharmacogenetics since functional polymorphisms which alter gene expression and mRNA processing appear to play a critical role in shaping human phenotypic variability [349]. It is also most relevant, from a practical point of view, to under- stand the pharmacogenomics of drug transporters, espe- cially ABCB1 (P-glycoprotein/MDR1) variants, due to the pleiotropic activity of this gene on a large number of drugs [350]. It is necessary to have a better documenta- tion related to the pharmacogenetic roles of the enor- mous number (>170) of human solute carrier transporters which transport a variety of substrates, including amino acids, lipids, inorganic ions, peptides, saccharides, metals, drugs, toxic xenobiotics, chemical compounds, and pro- teins [351]. RNAi pharmacogenomics will also bring new insights into the nature and therapeutic value of gene silencing in CNS disorders [352-356]. The optimization of CNS therapeutics, in general, and the pharmacological treatment of SCZ and psychotic disorders, in particular, requires the establishment of new postulates regarding 1) the costs of medicines; 2) the assessment of protocols for multifactorial treatment in chronic disorders; 3) the implementation of novel thera- peutics addressing causative factors; and 4) the seting-up of pharmacogenomic strategies for drug development and drugs on the market [2,6,14]. By knowing the pharmacogenomic profiles of patients who require treatments with psychotropic drugs of cur- rent use, it might be possible to obtain some of the fol- lowing benefits: 1) to identify candidate patients with the ideal genomic profile to receive a particular drug; 2) to adapt the dose in over 60% - 90% of the cases according to the condition of EM, IM, PM or UM (diminishing the occurrence of direct side-effects in 30% - 50% of the cases); 3) to reduce drug interactions by 30% - 50% (avoiding the administration of inhibitors or inducers able to modify the normal enzymatic activity on a par- ticular substrate); 4) to enhance efficacy and pharma- codynamic specificity; and 5) to eliminate unnecessary costs (>30% of pharmaceutical costs) derived from the consequences of an inappropriate drug selection and the overmedication administered to mitigate ADRs. 6. ACKNOWLEDGEMENTS We thank Carmen Fraile and Adam McKay for technical support. Most studies on pharmacogenomics of CNS disorders in our institu- tion are funded by the International Agency for Brain Research and Aging (IABRA). REFERENCES [1] Cacabelos, R., Fernández-Novoa, L., Lombardi, V., Carril, J.C., Corzo, L., Carrera, I., Tellado, I., Martínez, R., McKay, A. and Takeda, M. (2011) Genomics of schi- zophrenia and psychotic disorders. The EuroEspes Jour- nal: Gen-T, 3, 6-86. http://www.gen-t.es/ [2] Cacabelos, R., Martínez-Bouza, R., Carril, J.C., Fernández- Novoa, L., Lombardi, V., Carrera, I., Corzo, L. and McKay, A. (2012) Genomics and pharmacogenomics of brain disorders. Current Pharmaceutical Biotechnology, 13, 674-725. doi:10.2174/138920112799857576 [3] Andlin-Sobocki, P., Jönsson, B., Wittchen, H.-U. and Olesen, J. (2005) Costs of disorders of the brain in Euro- pe. European Journal of Neurology, 12, 1-27. [4] Cacabelos, R. (2008) Pharmacogenomics in Alzheimer disease. Methods in Molecular Biology, 448, 213-357. doi:10.1007/978-1-59745-205-2_10 [5] Cacabelos, R. (2009) Pharmacogenomic biomarkers in neuropsychiatry: The path to personalized medicine in mental disorders. In: Ritsner, M.S., Ed., The Handbook of Neuropsychiatric Biomarkers, Endophenotypes and Genes, Springer, Berlin, 3-63. doi:10.1007/978-90-481-2298-1_1 [6] Cacabelos, R., Martínez, R., Fernández-Novoa, L., Carril, J.C., Lombardi, V., Carrera, I., Corzo, L., Tellado, I., Leszek, J., McKay, A. and Takeda, M. (2012) Genomics of dementia: APOE- and CYP2D6-Related pharmacoge- netics. International Journal of Alzheimer disease, 2012, 37. doi:10.1155/2012/518901 [7] Malhotra, A.K., Lencz, T., Correll, C.U. and Kane, J.M. (2007) Genomics and the future of pharmacotherapy in psychiatry. International Review of Psychiatry, 19, 523- 530. doi:10.1080/09540260701563460 [8] Zhou, S.F., Di, Y.M., Chan, E., Du, Y.M., Chow, V.D., Xue, C.C., Lai, X., Wang, J.C., Li, C.G., Tian, M. and Duan, W. (2008) Clinical pharmacogenetics and potential application in personalized medicine. Current Drug Me- tabolism, 9, 738-784. doi:10.2174/138920008786049302 [9] Cacabelos, R. (2008) Pharmacogenomics and therapeutic prospects in dementia. European Archives of Psychiatry and Clinical Neuroscience, 258, 28-47. doi:10.1007/s00406-007-1006-x [10] Cacabelos, R. (2007) Pharmacogenetic basis for the- Copyright © 2013 SciRes. OPEN ACCESS
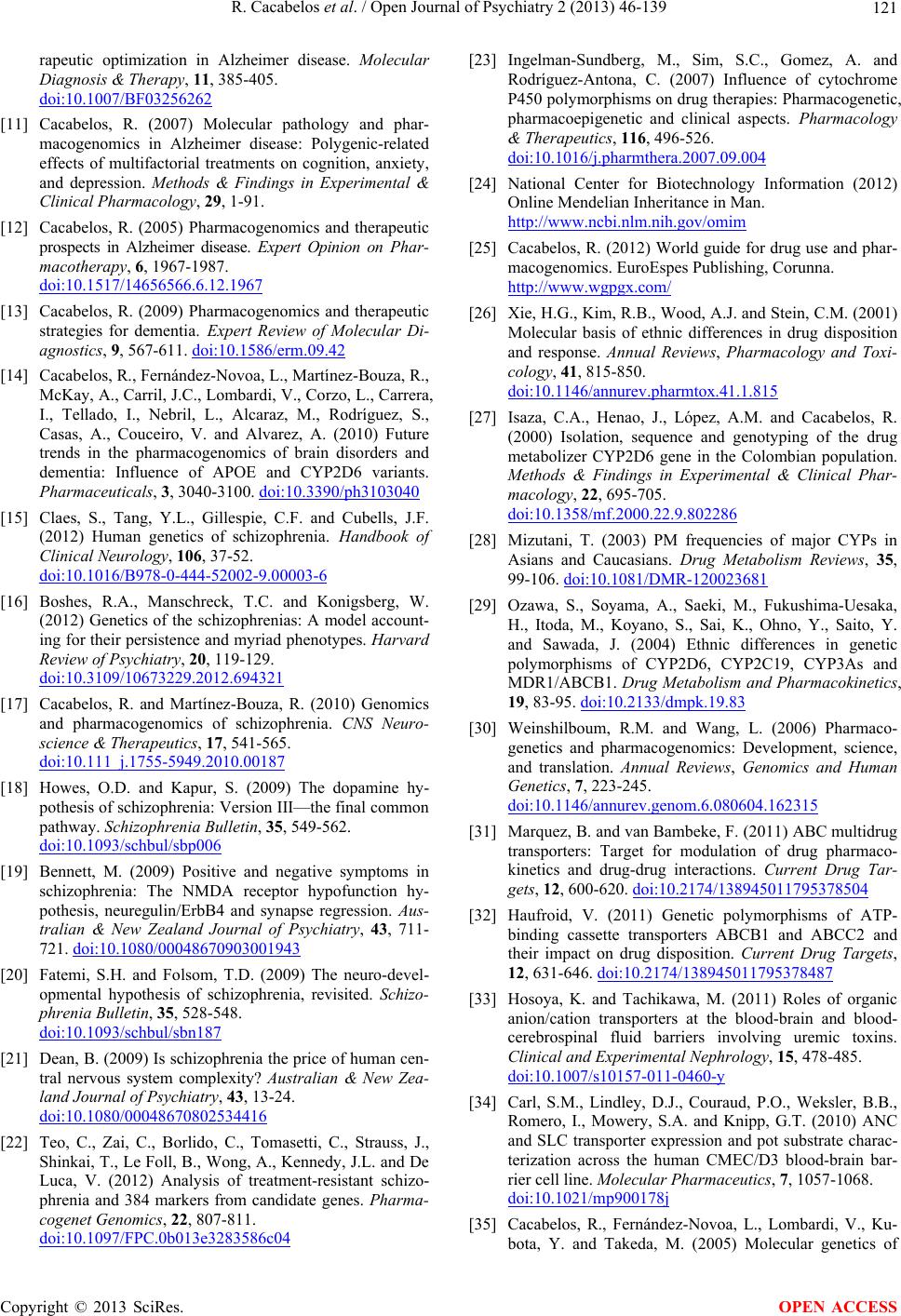 R. Cacabelos et al. / Open Journal of Psychiatry 2 (2013) 46-139 121 rapeutic optimization in Alzheimer disease. Molecular Diagnosis & Therapy, 11, 385-405. doi:10.1007/BF03256262 [11] Cacabelos, R. (2007) Molecular pathology and phar- macogenomics in Alzheimer disease: Polygenic-related effects of multifactorial treatments on cognition, anxiety, and depression. Methods & Findings in Experimental & Clinical Pharmacology, 29, 1-91. [12] Cacabelos, R. (2005) Pharmacogenomics and therapeutic prospects in Alzheimer disease. Expert Opinion on Phar- macotherapy, 6, 1967-1987. doi:10.1517/14656566.6.12.1967 [13] Cacabelos, R. (2009) Pharmacogenomics and therapeutic strategies for dementia. Expert Review of Molecular Di- agnostics, 9, 567-611. doi:10.1586/erm.09.42 [14] Cacabelos, R., Fernández-Novoa, L., Martínez-Bouza, R., McKay, A., Carril, J.C., Lombardi, V., Corzo, L., Carrera, I., Tellado, I., Nebril, L., Alcaraz, M., Rodríguez, S., Casas, A., Couceiro, V. and Alvarez, A. (2010) Future trends in the pharmacogenomics of brain disorders and dementia: Influence of APOE and CYP2D6 variants. Pharmaceuticals, 3, 3040-3100. doi:10.3390/ph3103040 [15] Claes, S., Tang, Y.L., Gillespie, C.F. and Cubells, J.F. (2012) Human genetics of schizophrenia. Handbook of Clinical Neurology, 106, 37-52. doi:10.1016/B978-0-444-52002-9.00003-6 [16] Boshes, R.A., Manschreck, T.C. and Konigsberg, W. (2012) Genetics of the schizophrenias: A model account- ing for their persistence and myriad phenotypes. Harvard Review of Psychiatry, 20, 119-129. doi:10.3109/10673229.2012.694321 [17] Cacabelos, R. and Martínez-Bouza, R. (2010) Genomics and pharmacogenomics of schizophrenia. CNS Neuro- science & Therapeutics, 17, 541-565. doi:10.111_j.1755-5949.2010.00187 [18] Howes, O.D. and Kapur, S. (2009) The dopamine hy- pothesis of schizophrenia: Version III—the final common pathway. Schizophrenia Bulletin, 35, 549-562. doi:10.1093/schbul/sbp006 [19] Bennett, M. (2009) Positive and negative symptoms in schizophrenia: The NMDA receptor hypofunction hy- pothesis, neuregulin/ErbB4 and synapse regression. Aus- tralian & New Zealand Journal of Psychiatry, 43, 711- 721. doi:10.1080/00048670903001943 [20] Fatemi, S.H. and Folsom, T.D. (2009) The neuro-devel- opmental hypothesis of schizophrenia, revisited. Schizo- phrenia Bulletin, 35, 528-548. doi:10.1093/schbul/sbn187 [21] Dean, B. (2009) Is schizophrenia the price of human cen- tral nervous system complexity? Australian & New Zea- land Journal of Psychiatry, 43, 13-24. doi:10.1080/00048670802534416 [22] Teo, C., Zai, C., Borlido, C., Tomasetti, C., Strauss, J., Shinkai, T., Le Foll, B., Wong, A., Kennedy, J.L. and De Luca, V. (2012) Analysis of treatment-resistant schizo- phrenia and 384 markers from candidate genes. Pharma- cogenet Genomics, 22, 807-811. doi:10.1097/FPC.0b013e3283586c04 [23] Ingelman-Sundberg, M., Sim, S.C., Gomez, A. and Rodríguez-Antona, C. (2007) Influence of cytochrome P450 polymorphisms on drug therapies: Pharmacogenetic, pharmacoepigenetic and clinical aspects. Pharmacology & Therapeutics, 116, 496-526. doi:10.1016/j.pharmthera.2007.09.004 [24] National Center for Biotechnology Information (2012) Online Mendelian Inheritance in Man. http://www.ncbi.nlm.nih.gov/omim [25] Cacabelos, R. (2012) World guide for drug use and phar- macogenomics. EuroEspes Publishing, Corunna. http://www.wgpgx.com/ [26] Xie, H.G., Kim, R.B., Wood, A.J. and Stein, C.M. (2001) Molecular basis of ethnic differences in drug disposition and response. Annual Reviews, Pharmacology and Toxi- cology, 41, 815-850. doi:10.1146/annurev.pharmtox.41.1.815 [27] Isaza, C.A., Henao, J., López, A.M. and Cacabelos, R. (2000) Isolation, sequence and genotyping of the drug metabolizer CYP2D6 gene in the Colombian population. Methods & Findings in Experimental & Clinical Phar- macology, 22, 695-705. doi:10.1358/mf.2000.22.9.802286 [28] Mizutani, T. (2003) PM frequencies of major CYPs in Asians and Caucasians. Drug Metabolism Reviews, 35, 99-106. doi:10.1081/DMR-120023681 [29] Ozawa, S., Soyama, A., Saeki, M., Fukushima-Uesaka, H., Itoda, M., Koyano, S., Sai, K., Ohno, Y., Saito, Y. and Sawada, J. (2004) Ethnic differences in genetic polymorphisms of CYP2D6, CYP2C19, CYP3As and MDR1/ABCB1. Drug Metabolism and Pharmacokinetics, 19, 83-95. doi:10.2133/dmpk.19.83 [30] Weinshilboum, R.M. and Wang, L. (2006) Pharmaco- genetics and pharmacogenomics: Development, science, and translation. Annual Reviews, Genomics and Human Genetics, 7, 223-245. doi:10.1146/annurev.genom.6.080604.162315 [31] Marquez, B. and van Bambeke, F. (2011) ABC multidrug transporters: Target for modulation of drug pharmaco- kinetics and drug-drug interactions. Current Drug Tar- gets, 12, 600-620. doi:10.2174/138945011795378504 [32] Haufroid, V. (2011) Genetic polymorphisms of ATP- binding cassette transporters ABCB1 and ABCC2 and their impact on drug disposition. Current Drug Targets, 12, 631-646. doi:10.2174/138945011795378487 [33] Hosoya, K. and Tachikawa, M. (2011) Roles of organic anion/cation transporters at the blood-brain and blood- cerebrospinal fluid barriers involving uremic toxins. Clinical and Experimental Nephrology, 15, 478-485. doi:10.1007/s10157-011-0460-y [34] Carl, S.M., Lindley, D.J., Couraud, P.O., Weksler, B.B., Romero, I., Mowery, S.A. and Knipp, G.T. (2010) ANC and SLC transporter expression and pot substrate charac- terization across the human CMEC/D3 blood-brain bar- rier cell line. Molecular Pharmaceutics, 7, 1057-1068. doi:10.1021/mp900178j [35] Cacabelos, R., Fernández-Novoa, L., Lombardi, V., Ku- bota, Y. and Takeda, M. (2005) Molecular genetics of Copyright © 2013 SciRes. OPEN ACCESS
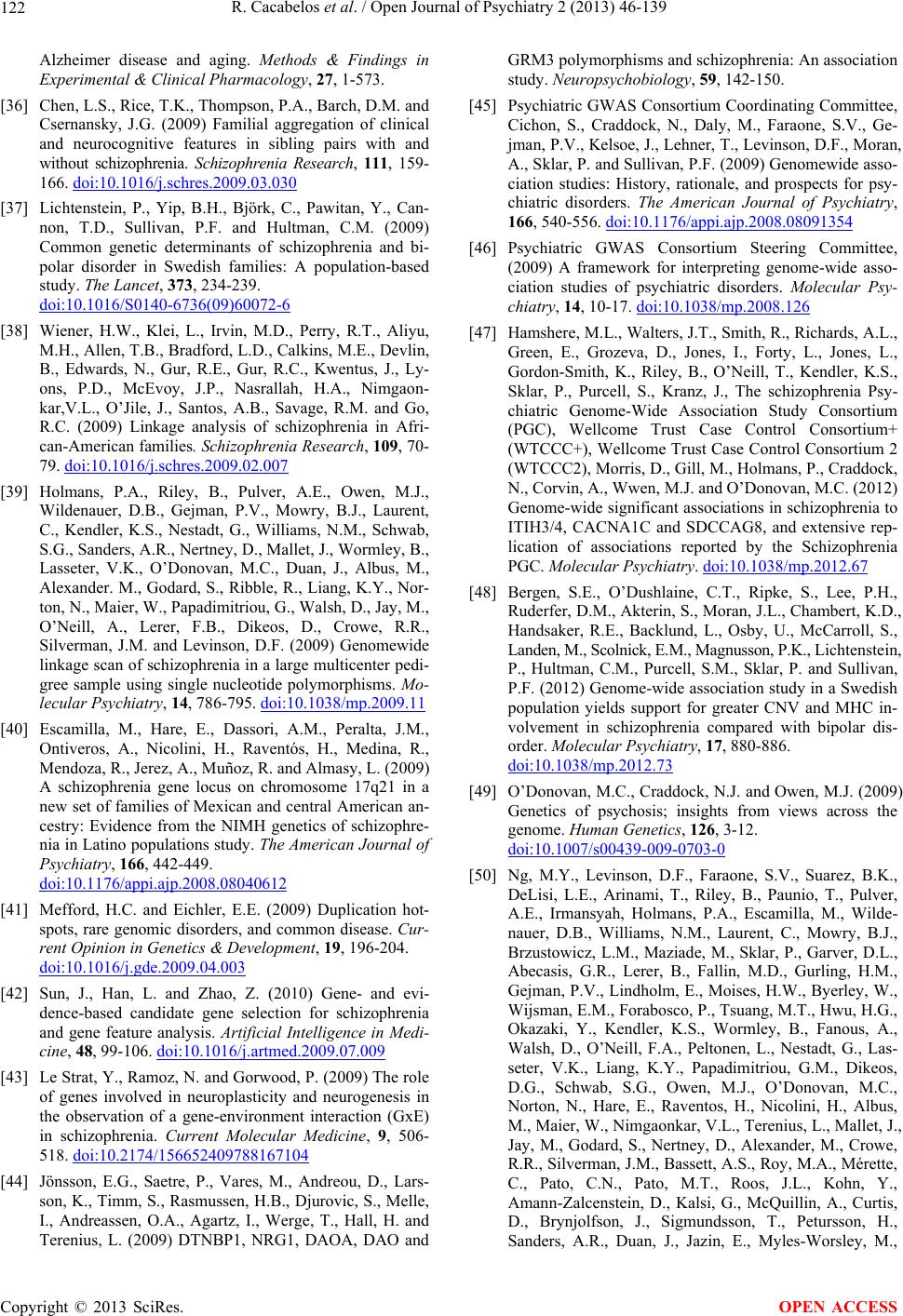 R. Cacabelos et al. / Open Journal of Psychiatry 2 (2013) 46-139 122 Alzheimer disease and aging. Methods & Findings in Experimental & Clinical Pharmacology, 27, 1-573. [36] Chen, L.S., Rice, T.K., Thompson, P.A., Barch, D.M. and Csernansky, J.G. (2009) Familial aggregation of clinical and neurocognitive features in sibling pairs with and without schizophrenia. Schizophrenia Research, 111, 159- 166. doi:10.1016/j.schres.2009.03.030 [37] Lichtenstein, P., Yip, B.H., Björk, C., Pawitan, Y., Can- non, T.D., Sullivan, P.F. and Hultman, C.M. (2009) Common genetic determinants of schizophrenia and bi- polar disorder in Swedish families: A population-based study. The Lancet, 373, 234-239. doi:10.1016/S0140-6736(09)60072-6 [38] Wiener, H.W., Klei, L., Irvin, M.D., Perry, R.T., Aliyu, M.H., Allen, T.B., Bradford, L.D., Calkins, M.E., Devlin, B., Edwards, N., Gur, R.E., Gur, R.C., Kwentus, J., Ly- ons, P.D., McEvoy, J.P., Nasrallah, H.A., Nimgaon- kar,V.L., O’Jile, J., Santos, A.B., Savage, R.M. and Go, R.C. (2009) Linkage analysis of schizophrenia in Afri- can-American families. Schizophrenia Research, 109, 70- 79. doi:10.1016/j.schres.2009.02.007 [39] Holmans, P.A., Riley, B., Pulver, A.E., Owen, M.J., Wildenauer, D.B., Gejman, P.V., Mowry, B.J., Laurent, C., Kendler, K.S., Nestadt, G., Williams, N.M., Schwab, S.G., Sanders, A.R., Nertney, D., Mallet, J., Wormley, B., Lasseter, V.K., O’Donovan, M.C., Duan, J., Albus, M., Alexander. M., Godard, S., Ribble, R., Liang, K.Y., Nor- ton, N., Maier, W., Papadimitriou, G., Walsh, D., Jay, M., O’Neill, A., Lerer, F.B., Dikeos, D., Crowe, R.R., Silverman, J.M. and Levinson, D.F. (2009) Genomewide linkage scan of schizophrenia in a large multicenter pedi- gree sample using single nucleotide polymorphisms. Mo- lecular Psychiatry, 14, 786-795. doi:10.1038/mp.2009.11 [40] Escamilla, M., Hare, E., Dassori, A.M., Peralta, J.M., Ontiveros, A., Nicolini, H., Raventós, H., Medina, R., Mendoza, R., Jerez, A., Muñoz, R. and Almasy, L. (2009) A schizophrenia gene locus on chromosome 17q21 in a new set of families of Mexican and central American an- cestry: Evidence from the NIMH genetics of schizophre- nia in Latino populations study. The American Journal of Psychiatry, 166, 442-449. doi:10.1176/appi.ajp.2008.08040612 [41] Mefford, H.C. and Eichler, E.E. (2009) Duplication hot- spots, rare genomic disorders, and common disease. Cur- rent Opinion in Genetics & Development, 19, 196-204. doi:10.1016/j.gde.2009.04.003 [42] Sun, J., Han, L. and Zhao, Z. (2010) Gene- and evi- dence-based candidate gene selection for schizophrenia and gene feature analysis. Artificial Intelligence in Medi- cine, 48, 99-106. doi:10.1016/j.artmed.2009.07.009 [43] Le Strat, Y., Ramoz, N. and Gorwood, P. (2009) The role of genes involved in neuroplasticity and neurogenesis in the observation of a gene-environment interaction (GxE) in schizophrenia. Current Molecular Medicine, 9, 506- 518. doi:10.2174/156652409788167104 [44] Jönsson, E.G., Saetre, P., Vares, M., Andreou, D., Lars- son, K., Timm, S., Rasmussen, H.B., Djurovic, S., Melle, I., Andreassen, O.A., Agartz, I., Werge, T., Hall, H. and Terenius, L. (2009) DTNBP1, NRG1, DAOA, DAO and GRM3 polymorphisms and schizophrenia: An association study. Neuropsychobiology, 59, 142-150. [45] Psychiatric GWAS Consortium Coordinating Committee, Cichon, S., Craddock, N., Daly, M., Faraone, S.V., Ge- jman, P.V., Kelsoe, J., Lehner, T., Levinson, D.F., Moran, A., Sklar, P. and Sullivan, P.F. (2009) Genomewide asso- ciation studies: History, rationale, and prospects for psy- chiatric disorders. The American Journal of Psychiatry, 166, 540-556. doi:10.1176/appi.ajp.2008.08091354 [46] Psychiatric GWAS Consortium Steering Committee, (2009) A framework for interpreting genome-wide asso- ciation studies of psychiatric disorders. Molecular Psy- chiatry, 14, 10-17. doi:10.1038/mp.2008.126 [47] Hamshere, M.L., Walters, J.T., Smith, R., Richards, A.L., Green, E., Grozeva, D., Jones, I., Forty, L., Jones, L., Gordon-Smith, K., Riley, B., O’Neill, T., Kendler, K.S., Sklar, P., Purcell, S., Kranz, J., The schizophrenia Psy- chiatric Genome-Wide Association Study Consortium (PGC), Wellcome Trust Case Control Consortium+ (WTCCC+), Wellcome Trust Case Control Consortium 2 (WTCCC2), Morris, D., Gill, M., Holmans, P., Craddock, N., Corvin, A., Wwen, M.J. and O’Donovan, M.C. (2012) Genome-wide significant associations in schizophrenia to ITIH3/4, CACNA1C and SDCCAG8, and extensive rep- lication of associations reported by the Schizophrenia PGC. Molecular Psychiatry. doi:10.1038/mp.2012.67 [48] Bergen, S.E., O’Dushlaine, C.T., Ripke, S., Lee, P.H., Ruderfer, D.M., Akterin, S., Moran, J.L., Chambert, K.D., Handsaker, R.E., Backlund, L., Osby, U., McCarroll, S., Landen, M., Scolnick, E.M., Magnusson, P.K., Lichtenstein, P., Hultman, C.M., Purcell, S.M., Sklar, P. and Sullivan, P.F. (2012) Genome-wide association study in a Swedish population yields support for greater CNV and MHC in- volvement in schizophrenia compared with bipolar dis- order. Molecular Psychiatry, 17, 880-886. doi:10.1038/mp.2012.73 [49] O’Donovan, M.C., Craddock, N.J. and Owen, M.J. (2009) Genetics of psychosis; insights from views across the genome. Human Genetics, 126, 3-12. doi:10.1007/s00439-009-0703-0 [50] Ng, M.Y., Levinson, D.F., Faraone, S.V., Suarez, B.K., DeLisi, L.E., Arinami, T., Riley, B., Paunio, T., Pulver, A.E., Irmansyah, Holmans, P.A., Escamilla, M., Wilde- nauer, D.B., Williams, N.M., Laurent, C., Mowry, B.J., Brzustowicz, L.M., Maziade, M., Sklar, P., Garver, D.L., Abecasis, G.R., Lerer, B., Fallin, M.D., Gurling, H.M., Gejman, P.V., Lindholm, E., Moises, H.W., Byerley, W., Wijsman, E.M., Forabosco, P., Tsuang, M.T., Hwu, H.G., Okazaki, Y., Kendler, K.S., Wormley, B., Fanous, A., Walsh, D., O’Neill, F.A., Peltonen, L., Nestadt, G., Las- seter, V.K., Liang, K.Y., Papadimitriou, G.M., Dikeos, D.G., Schwab, S.G., Owen, M.J., O’Donovan, M.C., Norton, N., Hare, E., Raventos, H., Nicolini, H., Albus, M., Maier, W., Nimgaonkar, V.L., Terenius, L., Mallet, J., Jay, M., Godard, S., Nertney, D., Alexander, M., Crowe, R.R., Silverman, J.M., Bassett, A.S., Roy, M.A., Mérette, C., Pato, C.N., Pato, M.T., Roos, J.L., Kohn, Y., Amann-Zalcenstein, D., Kalsi, G., McQuillin, A., Curtis, D., Brynjolfson, J., Sigmundsson, T., Petursson, H., Sanders, A.R., Duan, J., Jazin, E., Myles-Worsley, M., Copyright © 2013 SciRes. OPEN ACCESS
 R. Cacabelos et al. / Open Journal of Psychiatry 2 (2013) 46-139 123 Karayiorgou, M. and Lewis, C.M. (2009) Meta-analysis of 32 genome-wide linkage studies of schizophrenia. Molecular Psychiatry, 14, 774-785. doi:10.1038/mp.2008.135 [51] Moskvina, V., Craddock, N., Holmans, P., Nikolov, I., Pahwa, J.S., Green, E., Wellcome Trust Case Control Consortium, Owen, M.J. and O’Donovan, M.C. (2009) Gene-wide analyses of genome-wide association data sets: Evidence for multiple common risk alleles for schizo- phrenia and bipolar disorder and for overlap in genetic risk. Molecular Psychiatry, 14, 252-260. doi:10.1038/mp.2008.133 [52] Knight, H.M., Pickard, B.S., Maclean, A., Malloy, M.P., Soares, D.C., McRae, A.F., Condie, A., White, A., Haw- kins, W., McGhee, K., van Beck, M., MacIntyre, D.J., Starr, J.M., Deary, I.J., Visscher, P.M., Porteous, D.J., Cannon, R.E., St Clair, D., Muir, W.J. and Blackwood, D.H. (2009) A cytogenetic abnormality and rare coding variants identify ABCA13 as a candidate gene in schizo- phrenia, bipolar disorder, and depression. The American Journal of Human Genetics, 85, 833-846. doi:10.1016/j.ajhg.2009.11.003 [53] Ingason, A., Giegling, I., Cichon, S., Hansen, T., Ras- mussen, H.B., Nielsen, J., Jürgens, G., Muglia, P., Hart- mann, A.M., Strengman, E., Vasilescu, C., Mühleisen, T.W., Djurovic, S., Melle, I., Lerer, B., M?ller, H.J., Francks, C., Pietiläinen, O.P., Lonnqvist, J., Suvisaari, J., Tuulio-Henriksson, A., Walshe, M., Vassos, E., Di Forti, M., Murray, R., Bonetto, C., Tosato, S.; GROUP Investi- gators, Cantor, R.M., Rietschel, M., Craddock, N., Owen, M.J., Peltonen, L., Andreassen, O.A., Nöthen, M.M., St Clair, D., Ophoff, R.A., O’Donovan, M.C., Collier, D.A., Werge, T. and Rujescu, D. (2010) A large replication study and meta-analysis in European samples provides further support for association of AHI1 markers with schizophrenia. Human Molecular Genetics, 19, 1379- 1386. doi:10.1093/hmg/ddq009 [54] Ikeda, M., Tomita, Y., Mouri, A., Koga, M., Okochi, T., Yoshimura, R., Yamanouchi, Y., Kinoshita, Y., Hashi- moto, R., Williams, H.J., Takeda, M., Nakamura, J., Na- beshima, T., Owen, M.J., O’Donovan, M.C., Honda, H., Arinami, T., Ozaki, N. and Iwata, N. (2010) Identification of novel candidate genes for treatment response to risperidone and susceptibility for schizophrenia: Inte- grated analysis among pharmacogenomics, mouse ex- pression, and genetic case-control association approaches. Biological Psychiatry, 67, 263-269. doi:10.1016/j.biopsych.2009.08.030 [55] Rivero, O., Reif, A., Sanjuán, J., Moltó, M.D., Kittel- Schneider, S., Nájera, S., Töpner, T. and Lesch, K.P. (2010) Impact of the AHI1 gene on the vulnerability to schizophrenia: A case-control association study. PLoS One, 5, e12254. doi:10.1371/journal.pone.0012254 [56] Zhang, F., Xu, Y., Liu, P., Fan, H., Huang, X., Sun, G., Song, Y. and Sham, P.C. (2008) Association analyses of the interaction between the ADSS and ATM genes with schizophrenia in a Chinese population. BMC Medical Genetics, 9, 119. doi:10.1186/1471-2350-9-119 [57] Yamaguchi, W., Shinkai, T., Inoue, Y., Utsunomiya, K., Sakata, S., Fukunaka, Y., Yamada, K., Chen, H.I., Hwang, R., Ohmori, O. and Nakamura, J. (2009) Association analysis between the C-1291G polymorphism in the pro- moter region of the adrenergic alpha2A receptor gene and polydipsia in schizophrenia. Progress in Neuro-Psy- chopharmacology Biological Psychiatry, 33, 499-502. doi:10.1016/j.pnpbp.2009.01.012 [58] Wan, C., Shi, Y., Zhao, X., Tang, W., Zhang, M., Ji, B., Zhu, H., Xu, Y., Li, H., Feng, G. and He, L. (2009) Posi- tive association between ALDH1A2 and schizophrenia in the Chinese population. Progress in Neuro-Psychophar- macology Biological Psychiatry, 33, 1491-1495. doi:10.1016/j.pnpbp.2009.08.008 [59] Noori-Daloii, M.R., Kheirollahi, M., Mahbod, P., Mo- hammadi, F., Astaneh, A.N., Zarindast, M.R., Azimi, C. and Mohammadi, M.R. (2010) Alpha- and beta-synu- cleins mRNA expression in lymphocytes of schizophre- nia patients. Genetic Testing and Molecular Biomarkers, 14, 725-729. doi:10.1089/gtmb.2010.0050 [60] Kucukali, C.I., Aydin, M., Ozkok, E., Bilge, E., Zengin, A., Cakir, U. and Kara, I. (2010) Angiotensin-converting enzyme polymorphism in schizophrenia, bipolar disor- ders, and their first-degree relatives. Psychiatric Genetics, 20, 14-19. doi:10.1097/YPG.0b013e3283351194 [61] Hashimoto, R., Ohi, K., Okada, T., Yasuda, Y., Yama- mori, H., Hori, H., Hikita, T., Taya, S., Saitoh, O., Ko- suga, A., Tatsumi, M., Kamijima, K., Kaibuchi, K., Ta- keda, M. and Kunugi, H. (2009) Association analysis between schizophrenia and the AP-3 complex genes. Neuroscience Research, 65, 113-115. doi:10.1016/j.neures.2009.05.008 [62] Vik-Mo, A.O., Fernø, J., Skrede, S. and Steen, V.M. (2009) Psychotropic drugs up-regulate the expression of cholesterol transport proteins including ApoE in cultured human CNS- and liver cells. BMC Pharmacology, 9, 10. doi:10.1186/1471-2210-9-10 [63] Chen, X., Sun, C., Chen, Q., O’Neill, F.A., Walsh, D., Fanous, A.H., Chowdari, K.V., Nimgaonkar, V.L., Scott, A., Schwab, S.G., Wildenauer, D.B., Che, R., Tang, W., Shi, Y., He, L., Luo, X.J., Su, B., Edwards, T.L., Zhao, Z. and Kendler, K.S. (2009) Apoptotic engulfment pathway and schizophrenia. PLoS One, 4, e6875. doi:10.1371/journal.pone.0006875 [64] Levin, R., Heresco-Levy, U., Bachner-Melman, R., Israel, S., Shalev, I. and Ebstein, R.P. (2009) Association be- tween arginine vasopressin 1a receptor (AVPR1a) pro- moter region polymorphisms and prepulse inhibition. Psychoneuroendocrinology, 34, 901-908. doi:10.1016/j.psyneuen.2008.12.014 [65] Israel, S., Lerer, E., Shalev, I., Uzefovsky, F., Reibold, M., Bachner-Melman, R., Granot, R., Bornstein, G., Knafo, A., Yirmiya, N. and Ebstein, R.P. (2008) Molecu- lar genetic studies of the arginine vasopressin 1a receptor (AVPR1a) and the oxytocin receptor (OXTR) in human behaviour: From autism to altruism with some notes in between. Progress in Brain Research, 170, 435-449. doi:10.1016/S0079-6123(08)00434-2 [66] Hammock, E.A. and Young, L.J. (2006) Oxytocin, vaso- pressin and pair bonding: Implications for autism. Phi- losophical Transactions of the Royal Society B Biological Sciences, 361, 2187-2198. doi:10.1098/rstb.2006.1939 Copyright © 2013 SciRes. OPEN ACCESS
 R. Cacabelos et al. / Open Journal of Psychiatry 2 (2013) 46-139 124 [67] Liou, Y.J., Wang, Y.C., Chen, J.Y., Chen, M.L., Chen, T.T., Bai, Y.M., Lin, C.C., Liao, D.L. and Lai, I.C. (2008) The coding-synonymous polymorphism rs1045280 (Ser- 280Ser) in beta-arrestin 2 (ARRB2) gene is associated with tardive dyskinesia in Chinese patients with schizo- phrenia. European Journal of Neurology, 15, 1406-1408. doi:10.1111/j.1468-1331.2008.02316.x [68] Ohi, K., Hashimoto, R., Yasuda, Y., Yamamori, H., Hori, H., Saitoh, O., Tatsumi, M., Takeda, M., Iwata, N., Ozaki, N., Kamijima, K. and Kunugi, H. (2009) No association between the Bcl2-interacting killer (BIK) gene and schi- zophrenia. Neuroscience Letters, 463, 60-63. doi:10.1016/j.neulet.2009.07.063 [69] Cheli, V.T., Daniels, R.W., Godoy, R., Hoyle, D.J., Kan- dachar, V., Starcevic, M., Martinez-Agosto, J.A., Poole, S., DiAntonio, A., Lloyd, V.K., Chang, H.C., Krantz, D.E. and Dell’Angelica, E.C. (2009) Genetic modifiers of ab- normal organelle biogenesis in a Drosophila model of BLOC-1 deficiency. Human Molecular Genetics, 19, 861-878. doi:10.1093/hmg/ddp555 [70] Kawashima, K., Ikeda, M., Kishi, T., Kitajima, T., Ya- manouchi, Y., Kinoshita, Y., Okochi, T., Aleksic, B., Tomita, M., Okada, T., Kunugi, H., Inada, T., Ozaki, N. and Iwata, N. (2009) BDNF is not associated with schi- zophrenia: Data from a Japanese population study and meta-analysis. Schizophrenia Research, 112, 72-79. doi:10.1016/j.schres.2009.03.040 [71] Chang, H.A., Lu, R.B., Shy, M.J., Chang, C.C., Lee, M.S. and Huang, S.Y. (2009) Brain-derived neurotrophic fac- tor Val66Met polymorphism: Association with psycho- pathological symptoms of schizophrenia? The Journal of Neuropsychiatry & Clinical Neurosciences, 21, 30-37. doi:10.1176/appi.neuropsych.21.1.30 [72] Lencz, T., Lipsky, R.H., DeRosse, P., Burdick, K.E., Kane, J.M. and Malhotra, A.K. (2009) Molecular differ- entiation of schizoaffective disorder from schizophrenia using BDNF haplotypes. The British Journal of Psychia- try, 194, 313-318. doi:10.1192/bjp.bp.108.050401 [73] Wong, J., Webster, M.J., Cassano, H. and Weickert, C.S. (2009) Changes in alternative brain-derived neurotrophic factor transcript expression in the developing human pre- frontal cortex. European Journal of Neuroscience, 29, 1311-1322. doi:10.1111/j.1460-9568.2009.06669.x [74] Dunham, J.S., Deakin, J.F., Miyajima, F., Payton, A. and Toro, C.T. (2009) Expression of hippocampal brain-de- rived neurotrophic factor and its receptors in Stanley consortium brains. Journal of Psychiatric Research, 43, 1175-1184. doi:10.1016/j.jpsychires.2009.03.008 [75] Park, S.W., Lee, J.G., Kong, B.G., Lee, S.J., Lee, C.H., Kim, J.I. and Kim, Y.H. (2009) Genetic association of BDNF Val66Met and GSK-3beta-50T/C polymorphisms with tardive dyskinesia. Psychiatry and Clinical Neuro- sciences, 63, 433-439. doi:10.1111/j.1440-1819.2009.01976.x [76] Nyegaard, M., Severinsen, J.E., Als, T.D., Hedemand, A., Straarup, S., Nordentoft, M., McQuillin, A., Bass, N., Lawrence, J., Thirumalai, S., Pereira, A.C., Kandaswamy, R., Lydall, G.J., Sklar, P., Scolnick, E., Purcell, S., Curtis, D., Gurling, H.M., Mortensen, P.B., Mors, O. and Bør- glum, A.D. (2010) Support of association between BRD1 and both schizophrenia and bipolar affective disorder. American Journal of Medical Genetics. Part B: Neuro- psychiatric Genetics, 153B, 582-591. [77] Green, E.K., Grozeva, D., Jones, I., Jones, L., Kirov, G., Caesar, S., Gordon-Smith, K., Fraser, C., Forty, L., Rus- sell, E., Hamshere, M.L., Moskvina, V., Nikolov, I., Farmer, A., McGuffin, P., Wellcome Trust Case Control Consortium, Holmans, P.A., Owen, M.J., O’Donovan, M.C. and Craddock, N. (2010) The bipolar disorder risk allele at CACNA1C also confers risk of recurrent major depression and of schizophrenia. Molecular Psychiatry, 15, 1016-1022. doi:10.1038/mp.2009.49 [78] McMahon, F.J., Akula, N., Schulze, T.G., Muglia, P., Tozzi, F., Detera-Wadleigh, S.D., Steele, C.J., Breuer, R., Strohmaier, J., Wendland, J.R., Mattheisen, M., Mühle- isen, T.W., Maier, W., Nöthen, M.M., Cichon, S., Farmer, A., Vincent, J.B., Holsboer, F., Preisig, M., Rietschel, M. and Bipolar Disorder and Genome Study (BiGS) Consor- tium (2010) Meta-analysis of genome-wide association data identifies a risk locus for major mood disorders on 3p21.1. Nature Genetics, 42, 128-131. doi:10.1038/ng.523 [79] Bigos, K.L., Mattay, V.S., Callicott, J.H., Straub, R.E., Vakkalanka, R., Kolachana, B., Hyde, T.M., Lipska, B.K., Kleinman, J.E. and Weinberger, D.R. (2010) Genetic variation in CACNA1C affects brain circuitries related to mental illness. Archives of General Psychiatry, 67, 939- 945. doi:10.1001/archgenpsychiatry.2010.96 [80] Farokhashtiani, T., Mirabzadeh, A., Olad Nabi, M., Magham, Z.G, Khorshid, H.R., Najmabadi, H. and Ohadi, M. (2010) Reversion of the human calreticulin gene pro- moter to the ancestral type as a result of a novel psycho- sis-associated mutation. Progress in Neuro-Psychophar- macology and Biological Psychiatry, 35, 541-544. doi:10.1016/j.pnpbp.2010.12.012 [81] Ishiguro, H., Horiuchi, Y., Ishikawa, M., Koga, M., Imai, K., Suzuki, Y., Morikawa, M., Inada, T., Watanabe, Y., Takahashi, M., Someya, T., Ujike, H., Iwata, N., Ozaki, N., Onaivi, E.S., Kunugi, H., Sasaki, T., Itokawa, M., Arai, M., Niizato, K., Iritani, S., Naka, I., Ohashi, J., Kakita, A., Takahashi, H., Nawa, H. and Arinami, T. (2010) Brain cannabinoid CB2 receptor in schizophrenia. Biological Psychiatry, 67, 974-982. doi:10.1016/j.biopsych.2009.09.024 [82] Lendeckel, U., Kähne, T., Ten Have, S., Bukowska, A., Wolke, C., Bogerts, B., Keilhoff, G. and Bernstein, H.G. (2009) Cathepsin K generates enkephalin from beta-en- dorphin: A new mechanism with possible relevance for schizophrenia. Neurochemistry International, 54, 410- 417. doi:10.1016/j.neuint.2009.01.011 [83] Minato, T., Tochigi, M., Kato, N. and Sasaki, T. (2007) Association study between the cholecystokinin A recap- tor gene and schizophrenia in the Japanese population. Psychiatric Genetics, 17, 117-119. doi:10.1097/YPG.0b013e328011c02e [84] Sinkus, M.L., Lee, M.J., Gault, J., Logel, J., Short, M., Freedman, R., Christian, S.L., Lyon, J. and Leonard, S. (2009) A 2-base pair deletion polymorphism in the partial duplication of the alpha7 nicotinic acetylcholine gene (CHRFAM7A) on chromosome 15q14 is associated with Copyright © 2013 SciRes. OPEN ACCESS
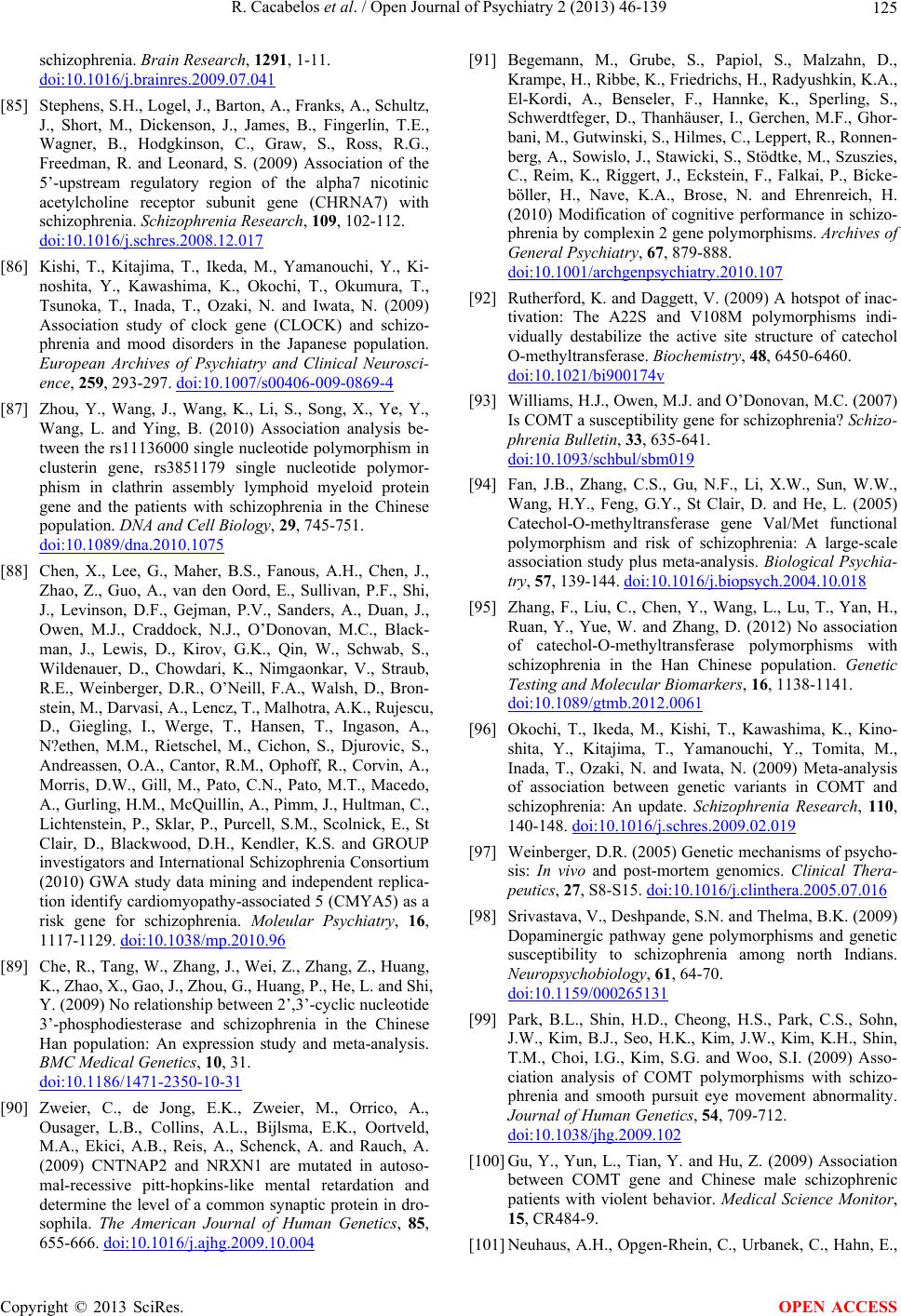 R. Cacabelos et al. / Open Journal of Psychiatry 2 (2013) 46-139 125 schizophrenia. Brain Research, 1291, 1-11. doi:10.1016/j.brainres.2009.07.041 [85] Stephens, S.H., Logel, J., Barton, A., Franks, A., Schultz, J., Short, M., Dickenson, J., James, B., Fingerlin, T.E., Wagner, B., Hodgkinson, C., Graw, S., Ross, R.G., Freedman, R. and Leonard, S. (2009) Association of the 5’-upstream regulatory region of the alpha7 nicotinic acetylcholine receptor subunit gene (CHRNA7) with schizophrenia. Schizophrenia Research, 109, 102-112. doi:10.1016/j.schres.2008.12.017 [86] Kishi, T., Kitajima, T., Ikeda, M., Yamanouchi, Y., Ki- noshita, Y., Kawashima, K., Okochi, T., Okumura, T., Tsunoka, T., Inada, T., Ozaki, N. and Iwata, N. (2009) Association study of clock gene (CLOCK) and schizo- phrenia and mood disorders in the Japanese population. European Archives of Psychiatry and Clinical Neurosci- ence, 259, 293-297. doi:10.1007/s00406-009-0869-4 [87] Zhou, Y., Wang, J., Wang, K., Li, S., Song, X., Ye, Y., Wang, L. and Ying, B. (2010) Association analysis be- tween the rs11136000 single nucleotide polymorphism in clusterin gene, rs3851179 single nucleotide polymor- phism in clathrin assembly lymphoid myeloid protein gene and the patients with schizophrenia in the Chinese population. DNA and Cell Biology, 29, 745-751. doi:10.1089/dna.2010.1075 [88] Chen, X., Lee, G., Maher, B.S., Fanous, A.H., Chen, J., Zhao, Z., Guo, A., van den Oord, E., Sullivan, P.F., Shi, J., Levinson, D.F., Gejman, P.V., Sanders, A., Duan, J., Owen, M.J., Craddock, N.J., O’Donovan, M.C., Black- man, J., Lewis, D., Kirov, G.K., Qin, W., Schwab, S., Wildenauer, D., Chowdari, K., Nimgaonkar, V., Straub, R.E., Weinberger, D.R., O’Neill, F.A., Walsh, D., Bron- stein, M., Darvasi, A., Lencz, T., Malhotra, A.K., Rujescu, D., Giegling, I., Werge, T., Hansen, T., Ingason, A., N?ethen, M.M., Rietschel, M., Cichon, S., Djurovic, S., Andreassen, O.A., Cantor, R.M., Ophoff, R., Corvin, A., Morris, D.W., Gill, M., Pato, C.N., Pato, M.T., Macedo, A., Gurling, H.M., McQuillin, A., Pimm, J., Hultman, C., Lichtenstein, P., Sklar, P., Purcell, S.M., Scolnick, E., St Clair, D., Blackwood, D.H., Kendler, K.S. and GROUP investigators and International Schizophrenia Consortium (2010) GWA study data mining and independent replica- tion identify cardiomyopathy-associated 5 (CMYA5) as a risk gene for schizophrenia. Moleular Psychiatry, 16, 1117-1129. doi:10.1038/mp.2010.96 [89] Che, R., Tang, W., Zhang, J., Wei, Z., Zhang, Z., Huang, K., Zhao, X., Gao, J., Zhou, G., Huang, P., He, L. and Shi, Y. (2009) No relationship between 2’,3’-cyclic nucleotide 3’-phosphodiesterase and schizophrenia in the Chinese Han population: An expression study and meta-analysis. BMC Medical Genetics, 10, 31. doi:10.1186/1471-2350-10-31 [90] Zweier, C., de Jong, E.K., Zweier, M., Orrico, A., Ousager, L.B., Collins, A.L., Bijlsma, E.K., Oortveld, M.A., Ekici, A.B., Reis, A., Schenck, A. and Rauch, A. (2009) CNTNAP2 and NRXN1 are mutated in autoso- mal-recessive pitt-hopkins-like mental retardation and determine the level of a common synaptic protein in dro- sophila. The American Journal of Human Genetics, 85, 655-666. doi:10.1016/j.ajhg.2009.10.004 [91] Begemann, M., Grube, S., Papiol, S., Malzahn, D., Krampe, H., Ribbe, K., Friedrichs, H., Radyushkin, K.A., El-Kordi, A., Benseler, F., Hannke, K., Sperling, S., Schwerdtfeger, D., Thanhäuser, I., Gerchen, M.F., Ghor- bani, M., Gutwinski, S., Hilmes, C., Leppert, R., Ronnen- berg, A., Sowislo, J., Stawicki, S., Stödtke, M., Szuszies, C., Reim, K., Riggert, J., Eckstein, F., Falkai, P., Bicke- böller, H., Nave, K.A., Brose, N. and Ehrenreich, H. (2010) Modification of cognitive performance in schizo- phrenia by complexin 2 gene polymorphisms. Archives of General Psychiatry, 67, 879-888. doi:10.1001/archgenpsychiatry.2010.107 [92] Rutherford, K. and Daggett, V. (2009) A hotspot of inac- tivation: The A22S and V108M polymorphisms indi- vidually destabilize the active site structure of catechol O-methyltransferase. Biochemistry, 48, 6450-6460. doi:10.1021/bi900174v [93] Williams, H.J., Owen, M.J. and O’Donovan, M.C. (2007) Is COMT a susceptibility gene for schizophrenia? Schizo- phrenia Bulletin, 33, 635-641. doi:10.1093/schbul/sbm019 [94] Fan, J.B., Zhang, C.S., Gu, N.F., Li, X.W., Sun, W.W., Wang, H.Y., Feng, G.Y., St Clair, D. and He, L. (2005) Catechol-O-methyltransferase gene Val/Met functional polymorphism and risk of schizophrenia: A large-scale association study plus meta-analysis. Biological Psychia- try, 57, 139-144. doi:10.1016/j.biopsych.2004.10.018 [95] Zhang, F., Liu, C., Chen, Y., Wang, L., Lu, T., Yan, H., Ruan, Y., Yue, W. and Zhang, D. (2012) No association of catechol-O-methyltransferase polymorphisms with schizophrenia in the Han Chinese population. Genetic Testing and Molecular Biomarkers, 16, 1138-1141. doi:10.1089/gtmb.2012.0061 [96] Okochi, T., Ikeda, M., Kishi, T., Kawashima, K., Kino- shita, Y., Kitajima, T., Yamanouchi, Y., Tomita, M., Inada, T., Ozaki, N. and Iwata, N. (2009) Meta-analysis of association between genetic variants in COMT and schizophrenia: An update. Schizophrenia Research, 110, 140-148. doi:10.1016/j.schres.2009.02.019 [97] Weinberger, D.R. (2005) Genetic mechanisms of psycho- sis: In vivo and post-mortem genomics. Clinical Thera- peutics, 27, S8-S15. doi:10.1016/j.clinthera.2005.07.016 [98] Srivastava, V., Deshpande, S.N. and Thelma, B.K. (2009) Dopaminergic pathway gene polymorphisms and genetic susceptibility to schizophrenia among north Indians. Neuropsychobiology, 61, 64-70. doi:10.1159/000265131 [99] Park, B.L., Shin, H.D., Cheong, H.S., Park, C.S., Sohn, J.W., Kim, B.J., Seo, H.K., Kim, J.W., Kim, K.H., Shin, T.M., Choi, I.G., Kim, S.G. and Woo, S.I. (2009) Asso- ciation analysis of COMT polymorphisms with schizo- phrenia and smooth pursuit eye movement abnormality. Journal of Human Genetics, 54, 709-712. doi:10.1038/jhg.2009.102 [100] Gu, Y., Yun, L., Tian, Y. and Hu, Z. (2009) Association between COMT gene and Chinese male schizophrenic patients with violent behavior. Medical Science Monitor, 15, CR484-9. [101] Neuhaus, A.H., Opgen-Rhein, C., Urbanek, C., Hahn, E., Copyright © 2013 SciRes. OPEN ACCESS
 R. Cacabelos et al. / Open Journal of Psychiatry 2 (2013) 46-139 126 Ta, T.M., Seidelsohn, M., Strathmann, S., Kley, F., Wie- seke, N., Sander, T. and Dettling, M. (2009) COMT Val 158 Met polymorphism is associated with cognitive flexibility in a signal discrimination task in schizophrenia. Pharmacopsychiatry, 42, 141-144. doi:10.1055/s-0028-1112132 [102] Takizawa, R., Tochigi, M., Kawakubo, Y., Marumo, K., Sasaki, T., Fukuda, M. and Kasai, K. (2009) Association between catechol-O-methyltrasferase Val108/158Met ge- notype and prefrontal hemodynamic response in schizo- phrenia. PLoS One, 4, e5495. doi:10.1371/journal.pone.0005495 [103] Chien, Y.L., Liu, C.M., Fann, C.S., Liu, Y.L. and Hwu, H.G. (2009) Association of the 3’ region of COMT with schizophrenia in Taiwan. Journal of the Formosan Medi- cal Association, 108, 301-309. doi:10.1016/S0929-6646(09)60070-X [104] Liao, S.Y., Lin, S.H., Liu, C.M., Hsieh, M.H., Hwang, T.J., Liu, S.K., Guo, S.C., Hwu, H.G. and Chen, W.J. (2009) Genetic variants in COMT and neurocognitive impairment in families of patients with schizophrenia. Genes, Brain and Behavior, 8, 228-237. doi:10.1111/j.1601-183X.2008.00467.x [105] Wang, Y., Hu, Y., Fang, Y., et al. (2009) Evidence of epistasis between the catechol-O-methyltransferase and aldehyde dehydrogenase 3B1 genes in paranoid schizo- phrenia. Biological Psychiatry, 65, 1048-1054. doi:10.1016/j.biopsych.2008.11.027 [106] Chen, P., Huang, K., Zhou, G., Zeng, Z., Wang, T., Li, B., Wang, Y., He, L., Feng, G. and Shi, Y. (2011) Common SNPs in CSF2RB are associated with major depression and schizophrenia in the Chinese Han population. World Journal of Biological Psychiatry, 12, 233-238. doi:10.3109/15622975.2010.544328 [107] Liu, J., Li, J., Li, T., Wang, T., Li, Y., Zeng, Z., Li, Z., Chen, P., Hu, Z., Zheng, L., Ji, J., Lin, H., Feng, G. and Shi, Y. (2011) CTLA-4 confers a risk of recurrent schizo- phrenia, major depressive disorder and bipolar disorder in the Chinese Han population. Brain, Behavior, and Immu- nity, 25, 429-433. doi:10.1016/j.bbi.2010.10.024 [108] Du, J., Xu, Y., Duan, S., Zhang, A., Xuan, J., Wang, L., Yu, L., Wang, H., Li, X., Feng, G., He, L. and Xing, Q. (2009) A case-control association study between the CYP3A4 and CYP3A5 genes and schizophrenia in the Chinese Han population. Progress in Neuro-Psycho- pharmacology and Biological Psychiatry, 33, 1200-1204. doi:10.1016/j.pnpbp.2009.06.023 [109] Ohi, K., Hashimoto, R., Yasuda, Y., Yoshida, T., Taka- hashi, H., Iike, N., Fukumoto, M., Takamura, H., Iwase, M., Kamino, K., Ishii, R., Kazui, H., Sekiyama, R., Ki- tamura, Y., Azechi, M., Ikezawa, K., Kurimoto, R., Kamagata, E., Tanimukai, H., Tagami, S., Morihara, T., Ogasawara, M., Okochi, M., Tokunaga, H., Numata, S., Ikeda, M., Ohnuma, T., Ueno, S., Fukunaga, T., Tanaka, T., Kudo, T., Arai, H., Ohmori, T., Iwata, N., Ozaki, N. and Takeda, M. (2009) Association study of the G72 gene with schizophrenia in a Japanese population: A multi- center study. Schizophrenia Research, 109, 80-85. doi:10.1016/j.schres.2009.01.019 [110] Detera-Wadleigh, S.D. and McMahon, F.J. (2006) G72/G30 in schizophrenia and bipolar disorder: Review and meta- analysis. Biological Psychiatry, 60, 106-114. doi:10.1016/j.biopsych.2006.01.019 [111] Gomez, L., Wigg, K., Feng, Y., Kiss, E., Kapornai, K., Tamás, Z., Mayer, L., Baji, I., Daróczi, G., Benák, I., Kothencné, V.O., Dombovári, E., Kaczvinszk, E., Besnyo, M., Gádoros, J., King, N., Székely, J., Kovacs, M., Vetró, A., Kennedy, J.L. and Barr, C.L. (2009) G72/G30 (DAOA) and juvenile-onset mood disorders. American Journal of Medical Genetics Part B: Neuropsychiatric Genetics, 150, 1007-1012. doi:10.1002/ajmg.b.30904 [112] Benzel, I., Kew, J.N., Viknaraja, R., Kelly, F., de Belle- roche, J., Hirsch, S., Sanderson, T.H. and Maycox, P.R. (2008) Investigation of G72 (DAOA) expression in the human brain. BMC Psychiatry, 8, 94. doi:10.1186/1471-244X-8-94 [113] Chubb, J.E., Bradshaw, N.J., Soares, D.C., Porteous, D.J. and Millar, J.K. (2008) The DISC locus in psychiatric illness. Molecular Psychiatry, 13, 36-64. doi:10.1038/sj.mp.4002106 [114] Nakata, K., Lipska, B.K., Hyde, T.M., Ye, T., Newburn, E.N., Morita, Y., Vakkalanka, R., Barenboim, M., Sei, Y., Weinberger, D.R. and Kleinman, J.E. (2009) DISC1 splice variants are upregulated in schizophrenia and asso- ciated with risk polymorphisms. Proceedings of the Na- tional Academy of Sciences of USA, 106, 15873-15878. doi:10.1073/pnas.0903413106 [115] Crepel, A., Breckpot, J., Fryns, J.P., De la Marche, W., Steyaert, J., Devriendt, K. and Peeters, H. (2010) DISC1 duplication in two brothers with autism and mild mental retardation. Clinical Genetics, 77, 389-394. doi:10.1111/j.1399-0004.2009.01318.x [116] Enomoto, A., Asai, N., Namba, T., Wang, Y., Kato, T., Tanaka, M., Tatsumi, H., Taya, S., Tsuboi, D., Kuroda, K., Kaneko, N., Sawamoto, K., Miyamoto, R., Jijiwa, M., Murakumo, Y., Sokabe, M., Seki, T., Kaibuchi, K. and Takahashi, M. (2009) Roles of disrupted-in-schizophrenia 1-interacting protein girdin in postnatal development of the dentate gyrus. Neuron, 63, 774-787. doi:10.1016/j.neuron.2009.08.015 [117] Kim, J.Y., Duan, X., Liu, C.Y., Jang, M.H., Guo, J.U., Pow-anpongkul, N., Kang, E., Song, H. and Ming, G.L. (2009) DISC1 regulates new neuron development in the adult brain via modulation of AKT-mTOR signaling through KIAA1212. Neuron, 63, 761-773. doi:10.1016/j.neuron.2009.08.008 [118] Matsuzaki, S. and Tohyama, M. (2007) Molecular me- chanism of schizophrenia with reference to disrupted- in-schizophrenia 1 (DISC1). Neurochemistry Interna- tional, 51, 165-172. doi:10.1016/j.neuint.2007.06.018 [119] Millar, J.K., Mackie, S., Clapcote, S.J., Murdoch, H., Pickard, B.S., Christie, S., Muir, W.J., Blackwood, D.H., Roder, J.C., Houslay, M.D. and Porteous, D.J. (2007) Disrupted in schizophrenia 1 and phosphodiesterase 4B: Towards an understanding of psychiatric illness. The Journal of Physiology, 584, 401-405. doi:10.1113/jphysiol.2007.140210 [120] Bradshaw, N.J., Ogawa, F., Antolin-Fontes, B., Chubb, Copyright © 2013 SciRes. OPEN ACCESS
 R. Cacabelos et al. / Open Journal of Psychiatry 2 (2013) 46-139 127 J.E., Carlyle, B.C., Christie, S., Claessens, A., Porteous, D.J. and Millar, J.K. (2008) DISC1, PDE4B, and NDE1 at the centrosome and synapse. Biochemical and Bio- physical Research Communications, 377, 1091-1096. doi:10.1016/j.bbrc.2008.10.120 [121] Tomppo, L., Hennah, W., Lahermo, P., Loukola, A., Tu- ulio-Henriksson, A., Suvisaari, J., Partonen, T., Ekelund, J., Lännqvist, J. and Peltonen, L. (2009) Association be- tween genes of disrupted in schizophrenia 1 (DISC1) in- teractors and schizophrenia supports the role of the DISC1 pathway in the etiology of major mental illnesses. Biological Psychiatry, 65, 1055-1062. doi:10.1016/j.biopsych.2009.01.014 [122] Drerup, C.M., Wiora, H.M., Topczewski, J. and Morris, J.A. (2009) Disc1 regulates foxd3 and sox10 expression, affecting neural crest migration and differentiation. De- velopment, 136, 2623-2632. doi:10.1242/dev.030577 [123] Takahashi, T., Suzuki, M., Tsunoda, M., Maeno, N., Ka- wasaki, Y., Zhou, S.Y., Hagino, H., Niu, L., Tsuneki, H., Kobayashi, S., Sasaoka, T., Seto, H., Kurachi, M. and Ozaki, N. (2009) The disrupted-in-schizophrenia-1 Ser- 704Cys polymorphism and brain morphology in schizo- phrenia. Psychiatry Research, 172, 128-135. doi:10.1016/j.pscychresns.2009.01.005 [124] Schumacher, J., Laje, G., Abou Jamra, R., Becker, T., Mühleisen, T.W., Vasilescu, C., Mattheisen, M., Herms, S., Hoffmann, P., Hillmer, A.M., Georgi, A., Herold, C., Schulze, T.G., Propping, P., Rietschel, M., McMahon, F.J., Nöthen, M.M. and Cichon, S. (2009) The DISC lo- cus and schizophrenia: evidence from an association study in a central European sample and from a meta- analysis across different European populations. Human Molecular Genetics, 18, 2719-2727. doi:10.1093/hmg/ddp204 [125] Zhang, C., Fang, Y., Xie, B., Cheng, W., Du, Y., Wang, D. and Yu, S. (2009) DNA methyltransferase 3B gene increases risk of early onset schizophrenia. Neuroscience Letters, 462, 308-311. doi:10.1016/j.neulet.2009.06.085 [126] Zhubi, A., Veldic, M., Puri, N.V., Kadriu, B., Caruncho, H., Loza, I., Sershen, H., Lajtha, A., Smith, R.C., Gui- dotti, A., Davis, J.M. and Costa, E. (2009) An upregula- tion of DNA-methyltransferase 1 and 3a expressed in telencephalic GABAergic neurons of schizophrenia pa- tients is also detected in peripheral blood lymphocytes. Schizophrenia Research, 111, 115-122. doi:10.1016/j.schres.2009.03.020 [127] Reuter, M., Weber, B., Fiebach, C.J., Elger, C., Montag, C. (2009) The biological basis of anger: Associations with the gene coding for DARPP-32 (PPP1R1B) and with amygdala volume. Behavioural Brain Research, 202, 179-183. doi:10.1016/j.bbr.2009.03.032 [128] Prata, D.P., Mechelli, A., Fu, C.H., Picchioni, M., Tou- lopoulou, T., Bramon, E., Walshe, M., Murray, R.M., Collier, D.A. and McGuire, P. (2009) Epistasis between the DAT 3’ UTR VNTR and the COMT Val158Met SNP on cortical function in healthy subjects and patients with schizophrenia. Proceedings of the National Academy of Sciences of the USA, 106, 13600-13605. doi:10.1073/pnas.0903007106 [129] Prata, D.P., Mechelli, A., Picchioni, M.M., Fu, C.H., Toulopoulou, T., Bramon, E., Walshe, M., Murray, R.M., Collier, D.A. and McGuire, P. (2009) Altered effect of dopamine transporter 3’UTR VNTR genotype on pre- frontal and striatal function in schizophrenia. Archives of General Psychiatry, 66, 1162-1172. doi:10.1001/archgenpsychiatry.2009.147 [130] Gupta, M., Chauhan, C., Bhatnagar, P., Gupta, S., Grover, S., Singh, P.K., Purushottam, M., Mukherjee, O., Jain, S., Brahmachari, S.K. and Kukreti, R. (2009) Genetic sus- ceptibility to schizophrenia: role of dopaminergic path- way gene polymorphisms. Pharmacogenomics, 10, 277- 291. doi:10.2217/14622416.10.2.277 [131] Hattori, E., Nakajima, M., Yamada, K., Iwayama, Y., Toyota, T., Saitou, N. and Yoshikawa, T. (2009) Variable number of tandem repeat polymorphisms of DRD4: Re-evaluation of selection hypothesis and analysis of as- sociation with schizophrenia. European Journal of Hu- man Genetics, 17, 793-801. doi:10.1038/ejhg.2008.247 [132] Zai, C.C., Tiwari, A.K., De Luca, V., Müller, D.J., Bulgin, N., Hwang, R., Zai, G.C., King, N., Voineskos, A.N., Meltzer, H.Y., Lieberman, J.A., Potkin, S.G., Remington, G. and Kennedy, J.L. (2009) Genetic study of BDNF, DRD3, and their interaction in tardive dyskinesia. Euro- pean Neuropsychopharmacology, 19, 317-328. doi:10.1016/j.euroneuro.2009.01.001 [133] Zai, C.C., Tiwari, A.K., Basile, V., De Luca, V., Müller, D.J., King, N., Voineskos, A.N., Remington, G., Meltzer, H.Y., Lieberman, J.A., Potkin, S.G. and Kennedy, J.L. (2009) Association study of tardive dyskinesia and five DRD4 polymorphisms in schizophrenia patients. Journal of Pharmacogenomics, 9, 168-174. doi:10.1038/tpj.2009.2 [134] Maher, B.S., Reimers, M.A., Riley, B.P. and Kendler, K.S. (2009) Allelic heterogeneity in genetic association meta-analysis: An application to DTNBP1 and schizo- phrenia. Human Heredity, 69, 71-79. doi:10.1159/000264445 [135] Dwyer, S., Carroll, L., Mantripragada, K.K., Owen, M.J., O’Donovan, M.C. and Williams, N.M. (2010) Mutation screening of the DTNBP1 exonic sequence in 669 schi- zophrenics and 710 controls using high-resolution melt- ing analysis. American Journal of Medical Genetics Part B: Neuropsychiatric Genetics, 153B, 766-774. doi:10.1002/ajmg.b.31045 [136] Ryder, P.V. and Faundez, V. (2009) Schizophrenia: The “BLOC” may be in the endosomes. Science Signaling, 2, e66. doi:10.1126/scisignal.293pe66 [137] Taneichi-Kuroda, S., Taya, S., Hikita, T., Fujino, Y. and Kaibuchi, K. (2009) Direct interaction of Dysbindin with the AP-3 complex via its mu subunit. Neurochemistry In- ternational, 54, 431-438. doi:10.1016/j.neuint.2009.01.014 [138] Hikita, T., Taya, S., Fujino, Y., Taneichi-Kuroda, S., Ohta, K., Tsuboi, D., Shinoda, T., Kuroda, K., Funahashi, Y., Uraguchi-Asaki, J., Hashimoto, R. and Kaibuchi, K. (2009) Proteomic analysis reveals novel binding partners of dysbindin, a schizophrenia-related protein. Journal of Neurochemistry, 110, 1567-1574. doi:10.1111/j.1471-4159.2009.06257.x Copyright © 2013 SciRes. OPEN ACCESS
 R. Cacabelos et al. / Open Journal of Psychiatry 2 (2013) 46-139 128 [139] Donohoe, G., Frodl, T., Morris, D., Spoletini, I., Cannon, D.M., Cherubini, A., Caltagirone, C., Bossù, P., McDon- ald, C., Gill, M., Corvin, A.P. and Spalletta, G. (2010) Reduced occipital and prefrontal brain volumes in dys- bindin-associated schizophrenia. Neuropsychopharma- cology, 35, 368-373. doi:10.1038/npp.2009.140 [140] Pae, C.U., Mandelli, L., De Ronchi, D., Kim, J.J., Jun, T.Y., Patkar, A.A. and Serretti, A. (2009) Dysbindin gene (DTNBP1) and schizophrenia in Korean population. European Archives of Psychiatry and Clinical Neurosci- ence, 259, 137-142. doi:10.1007/s00406-008-0830-y [141] Gaysina, D., Cohen-Woods, S., Chow, P.C., Martucci, L., Schosser, A., Ball, H.A., Tozzi, F., Perry, J., Muglia, P., Craig, I.W., McGuffin, P. and Farmer, A. (2009) Asso- ciation of the dystrobrevin binding protein 1 gene (DTNBP1) in a bipolar case-control study (BACCS). American Journal of Medical Genetics Part B: Neuro- psychiatric Genetics, 150, 836-844. doi:10.1002/ajmg.b.30906 [142] Tan, W., Dean, M. and Law, A.J. (2010) Molecular clon- ing and characterization of the human ErbB4 gene: Iden- tification of novel splice isoforms in the developing and adult brain. PLoS One, 5, e12924. doi:10.1371/journal.pone.0012924 [143] Wong, J. and Weickert, C.S. (2009) Transcriptional in- teraction of an estrogen receptor splice variant and ErbB4 suggests convergence in gene susceptibility pathways in schizophrenia. The Journal of Biological Chemisty, 284, 18824-18832. doi:10.1074/jbc.M109.013243 [144] Li, D., Feng, G. and He, L. (2009) Case-control study of association between the functional candidate gene ERBB3 and schizophrenia in Caucasian population. World Jour- nal of Biological Psychiatry, 10, 595-598. doi:10.1080/15622970903304442 [145] McNamara, R.K., Jandacek, R., Rider, T., Tso, P., Hahn, C.G., Richtand, N.M. and Stanford, K.E. (2007) Abnor- malities in the fatty acid composition of the post-mortem orbitofrontal cortex of schizophrenic patients: Gender differences and partial normalization with antipsychotic medications. Schizophrenia Research, 91, 37-50. doi:10.1016/j.schres.2006.11.027 [146] Liu, Y., Jandacek, R., Rider, T., Tso, P. and McNamara, R.K. (2009) Elevated delta-6 desaturase (FADS2) ex- pression in the post-mortem prefrontal cortex of schizo- phrenic patients: Relationship with fatty acid composition. Schizophrenia Research, 109, 113-120. doi:10.1016/j.schres.2008.12.027 [147] Perez-Iglesias, R., Mata, I., Amado, J.A., Berja, A., Gar- cia-Unzueta, M.T., Martínez, G.O., Arranz, M.J., Vaz- quez-Barquero, J.L. and Crespo-Facorro, B. (2010) Effect of FTO, SH2B1, LEP, and LEPR polymorphisms on weight gain associated with antipsychotic treatment. Journal of Clinical Psychopharmacology, 30, 661-666. doi:10.1097/JCP.0b013e3181fae248 [148] Zhong, N., Zhang, R., Qiu, C., Yan, H., Valenzuela, R.K., Zhang, H., Kang, W., Lu, S., Guo, T. and Ma, J. (2011) A novel replicated association between FXYD6 gene and schizophrenia. Biochemical and Biophysical Research Communications, 405, 118-121. doi:10.1016/j.bbrc.2011.01.005 [149] Hattori, K., Fukuzako, H., Hashiguchi, T., Hamada, S., Murata, Y., Isosaka, T., Yuasa, S. and Yagi, T. (2009) Decreased expression of Fyn protein and disbalanced al- ternative splicing patterns in platelets from patients with schizophrenia. Psychiatry Research, 168, 119-128. doi:10.1016/j.psychres.2008.04.014 [150] Szczepankiewicz, A., Rybakowski, J.K., Skibinska, M., Dmitrzak-Weglarz, M., Leszczynska-Rodziewicz, A., Wilkosc, M. and Hauser, J. (2009) FYN kinase gene: Another glutamatergic gene associated with bipolar dis- order? Neuropsychobiology, 59, 178-183. doi:10.1159/000219305 [151] Mellios, N., Huang, H.S., Baker, S.P., Galdzicka, M., Ginns, E. and Akbarian, S. (2009) Molecular determi- nants of dysregulated GABAergic gene expression in the prefrontal cortex of subjects with schizophrenia. Biologi- cal Psychiatry, 65, 1006-1014. doi:10.1016/j.biopsych.2008.11.019 [152] Hirunsatit, R., George, E.D., Lipska, B.K., Elwafi, H.M., Sander, L., Yrigollen, C.M., Gelernter, J., Grigorenko, E.L., Lappalainen, J., Mane, S., Nairn, A.C., Kleinman, J.E. and Simen, A.A. (2009) Twenty-one-base-pair inser- tion polymorphism creates an enhancer element and po- tentiates SLC6A1 GABA transporter promoter activity. Pharmacogenetics Genomics, 19, 53-65. doi:10.1097/FPC.0b013e328318b21a [153] Butticaz, C., Werge, T., Beckmann, J.S., Cuénod, M., Do, K.Q. and Rivolta, C. (2009) Mutation screening of the glutamate cysteine ligase modifier (GCLM) gene in pa- tients with schizophrenia. Psychiatric Genetics, 19, 201- 208. doi:10.1097/YPG.0b013e32832cef21 [154] Segnitz, N., Schmitt, A., Gebicke-Härter, P.J. and Zink, M. (2009) Differential expression of glutamate transpor- ter genes after chronic oral treatment with aripiprazole in rats. Neurochemistry International, 55, 619-628. doi:10.1016/j.neuint.2009.06.003 [155] Arai, S., Shibata, H., Sakai, M., Ninomiya, H., Iwata, N., Ozaki, N. and Fukumaki, Y. (2009) Association analysis of the glutamic acid decarboxylase 2 and the glutamine synthetase genes (GAD2, GLUL) with schizophrenia. Psychiatric Genetics, 19, 6-13. [156] Kilic, G., Ismail, K.C., Orhan, N., Ozkok, E., Zengin, A., Aydin, M. and Kara, I. (2009) Are GRIK3 (T928G) gene variants in schizophrenia patients different from those in their first-degree relatives? Psychiatry Research, 175, 43-46. [157] Gaisler-Salomon, I., Miller, G.M., Chuhma, N., Lee, S., Zhang, H., Ghoddoussi, F., Lewandowski, N., Fairhurst, S., Wang, Y., Conjard-Duplany, A., Masson, J., Balsam, P., Hen, R., Arancio, O., Galloway, M.P., Moore, H.M., Small, S.A. and Rayport, S. (2009) Glutaminase-deficient mice display hippocampal hypoactivity, insensitivity to pro-psychotic drugs and potentiated latent inhibition: Relevance to schizophrenia. Neuropsychopharmacology, 34, 2305-2322. doi:10.1038/npp.2009.58 [158] Treutlein, J., Mühleisen, T.W., Frank, J., Mattheisen, M., Herms, S., Ludwig, K.U., Treutlein, T., Schmael, C., Strohmaier, J., Bösshenz, K.V., Breuer, R., Paul, T., Witt, S.H., Schulze, T.G., Schlösser, R.G., Nenadic, I., Sauer, Copyright © 2013 SciRes. OPEN ACCESS
 R. Cacabelos et al. / Open Journal of Psychiatry 2 (2013) 46-139 129 H., Becker, T., Maier, W., Cichon, S., Nöthen, M.M. and Rietschel, M. (2009) Dissection of phenotype reveals possible association between schizophrenia and glutamate receptor delta 1 (GRID1) gene promoter. Schizophrenia Research, 111, 123-130. doi:10.1016/j.schres.2009.03.011 [159] Ghose, S., Chin, R., Gallegos, A., Roberts, R., Coyle, J. and Tamminga, C. (2009) Localization of NAAG-related gene expression deficits to the anterior hippocampus in schizophrenia. Schizophrenia Research, 111, 131-137. doi:10.1016/j.schres.2009.03.038 [160] Shibata, H., Tani, A., Chikuhara, T., Kikuta, R., Sakai, M., Ninomiya, H., Tashiro, N., Iwata, N., Ozaki, N. and Fukumaki, Y. (2009) Association study of polymorphi- sms in the group III metabotropic glutamate receptor genes, GRM4 and GRM7, with schizophrenia. Psychiatry Research, 167, 88-96. doi:10.1016/j.psychres.2007.12.002 [161] Ohnuma, T., Shibata, N., Maeshima, H., Baba, H., Hata- no, T., Hanzawa, R. and Arai, H. (2009) Association analysis of glycine- and serine-related genes in a Japa- nese population of patients with schizophrenia. Progress in Neuro-Psychopharmacology and Biological Psychiatry, 33, 511-518. doi:10.1016/j.pnpbp.2009.02.004 [162] Belforte, J.E., Zsiros, V., Sklar, E.R., Jiang, Z., Yu, G., Li, Y., Quinlan, E.M. and Nakazawa, K. (2009) Postnatal NMDA receptor ablation in corticolimbic interneurons confers schizophrenia-like phenotypes. Nature Neuro- science, 13, 76-83. doi:10.1038/nn.2447 [163] Li, B., Devidze, N., Barengolts, D., Prostak, N., Sphicas, E., Apicella, A.J., Malinow, R. and Emamian, E.S. (2009) NMDA receptor phosphorylation at a site affected in schizophrenia controls synaptic and behavioral plasticity. The Journal of Neuroscience, 29, 11965-11972. doi:10.1523/JNEUROSCI.2109-09.2009 [164] Kang, S.G., Lee, H.J., Choi, J.E., An, H., Rhee, M. and Kim, L. (2009) Association study between glutathione S-transferase GST-M1, GST-T1, and GST-P1 polymor- phisms and tardive dyskinesia. Human Psychopharma- cology, 24, 55-60. doi:10.1002/hup.988 [165] Eom, T.Y. and Jope, R.S. (2009) Blocked inhibitory ser- ine-phosphorylation of glycogen synthase kinase-3al- pha/beta impairs in vivo neural precursor cell prolifera- tion. Biological Psychiatry, 66, 494-502. doi:10.1016/j.biopsych.2009.04.015 [166] Dewachter, I., Ris, L., Jaworski, T., Seymour, C.M., Kremer, A., Borghgraef, P., De Vijver, H., Godaux, E. and Van Leuven, F. (2009) GSK3beta, a centre-staged kinase in neuropsychiatric disorders, modulates long term memory by inhibitory phosphorylation at serine-9. Neu- robiology of Disease, 35, 193-200. doi:10.1016/j.nbd.2009.04.003 [167] Baruch, K., Silberberg, G., Aviv, A., Shamir, E., Bening- Abu-Shach, U., Baruch, Y., Darvasi, A. and Navon, R. (2009) Association between golli-MBP and schizophrenia in the Jewish Ashkenazi population: Are regulatory re- gions involved? The International Journal of Neuropsy- chopharmacology, 12, 885-894. doi:10.1017/S1461145708009887 [168] Kalkman, H.O. (2009) Altered growth factor signaling pathways as the basis of aberrant stem cell maturation in schizophrenia. Pharmacology & Therapeutics, 121, 115- 122. doi:10.1016/j.pharmthera.2008.11.002 [169] Klejbor, I., Kucinski, A., Wersinger, S.R., Corso, T., Spodnik, J.H., Dziewiatkowski, J., Moryś, J., Hesse, R.A., Rice, K.C., Miletich, R., Stachowiak, E.K. and Sta- chowiak, M.K. (2009) Serotonergic hyperinnervation and effective serotonin blockade in an FGF receptor devel- opmental model of psychosis. Schizophrenia Research, 113, 308-321. doi:10.1016/j.schres.2009.06.006 [170] Pae, C.U., Drago, A., Kim, J.J., Mandelli, L., De Ronchi, D. and Serretti, A. (2009) The impact of heat shock pro- tein 70 gene variations on clinical presentation and out- come in schizophrenic inpatients. Neuropsychobiology, 59, 135-141. doi:10.1159/000218075 [171] Gilks, W.P., Allott, E.H., Donohoe, G., Cummings, E., International Schizophrenia Consortium, Gill, M., Corvin, A.P. and Morris, D.W. (2009) Replicated genetic evi- dence supports a role for HOMER2 in schizophrenia. Neuroscience Letters, 468, 229-233. doi:10.1016/j.neulet.2009.11.003 [172] Paul-Samojedny, M., Owczarek, A., Suchanek, R., Kowalczyk, M., Fila-Danilow, A., Borkowska, P., Kucia, K. and Kowalski, J. (2011) Association study of inter- feron gamma (IFN-γ) +874T/A gene polymorphism in patients with paranoid schizophrenia. Journal of Moleuar Neuroscience, 43, 309-315. doi:10.1007/s12031-010-9442-x [173] Frezza, D., Giambra, V., Mattioli, C., Piccoli, K., Mas- soud, R., Siracusano, A., Di Giannantonio, M., Birshtein, B.K. and Rubino, I.A. (2009) Allelic frequencies of 3’ Ig heavy chain locus enhancer HS1,2-A associated with Ig levels in patients with schizophrenia. International Jour- nal of Immunopathology and Pharmacology, 22, 115-123. [174] Bonvicini, C., Gennarelli, M., Scassellati, C., Bignotti, S., Gardella, R., Barlati, S., Valsecchi, P. and Sacchetti, E. (2010) Polymorphic CA repeat in IGF-I gene: Lack of association with schizophrenia. Psychiatric Genetics, 20, 44-45. doi:10.1097/YPG.0b013e3283351167 [175] Bernstein, H.G., Ernst, T., Lendeckel, U., Bukowska, A., Ansorge, S., Stauch, R., Have, S.T., Steiner, J., Do- browolny, H. and Bogerts, B. (2009) Reduced neuronal expression of insulin-degrading enzyme in the dorsolat- eral prefrontal cortex of patients with haloperidol-treated, chronic schizophrenia. Journal of Psychiatric Research, 43, 1095-1105. doi:10.1016/j.jpsychires.2009.03.006 [176] Sun, S., Wei, J., Li, H., Jin, S., Li, P., Ju, G., Liu, Y. and Zhang, X.Y. (2009) A family-based study of the IL3RA gene on susceptibility to schizophrenia in a Chinese Han population. Brain Research, 1268, 13-16. doi:10.1016/j.brainres.2009.02.071 [177] Ozbey, U., Tug, E. and Namli, M. (2009) Interleukin-10 gene promoter polymorphism in patients with schizo- phrenia in a region of East Turkey. World Journal of Biological Psychiatry, 10, 461-468. doi:10.1080/15622970802626580 [178] Liu, Y., Chen, G. and Norton, N. (2009) Whole genome association study in a homogenous population in Shan- dong peninsula of China reveals JARID2 as a susceptibil- Copyright © 2013 SciRes. OPEN ACCESS
 R. Cacabelos et al. / Open Journal of Psychiatry 2 (2013) 46-139 130 ity gene for schizophrenia. Journal of Biomedicine and Biotechnology, 2009, 536918. doi:10.1155/2009/536918 [179] Huffaker, S.J., Chen, J., Nicodemus, K.K., Sambataro, F., Yang, F., Mattay, V., Lipska, B.K., Hyde, T.M., Song, J., Rujescu, D., Giegling, I., Mayilyan, K., Proust, M.J., Soghoyan, A., Caforio, G., Callicott, J.H., Bertolino, A., Meyer-Lindenberg A., Chang, J., Ji, Y., Egan, M.F., Goldberg, T.E., Kleinman, J.E., Lu, B. and Weinberger, D.R. (2009) A primate-specific, brain isoform of KCNH2 affects cortical physiology, cognition, neuronal repolari- zation and risk of schizophrenia. Nature Medicine, 15, 509-18. doi:10.1038/nm.1962 [180] Shen, Q., Zhang, J., Wang, Y., Liu, B., Li, X., Zhao, Q., Chen, S., Ji, J., Yang, F., Wan, C., Gao, L., Xu, Y., Feng, G., He, L. and He, G. (2011) No association between the KCNH1, KCNJ10 and KCNN3 genes and schizophrenia in the Han Chinese population. Neuroscience Letters, 487, 61-65. doi:10.1016/j.neulet.2010.09.074 [181] Miller, C.L., Murakami, P., Ruczinski, I., Ross, R.G., Sinkus, M., Sullivan, B. and Leonard, S. (2009) Two complex genotypes relevant to the kynurenine pathway and melanotropin function show association with schizo- phrenia and bipolar disorders. Schizophrenia Research, 113, 259-267. doi:10.1016/j.schres.2009.05.014 [182] Koide, T., Banno, M., Aleksic, B., Yamashita, S., Kiku- chi, T., Kohmura, K., Adachi, Y., Kawano, N., Kushima, I., Nakamura, Y., Okada, T., Ikeda, M., Ohi, K., Yasuda, Y., Hashimoto, R., Inada, T., Ujike, H., Iidaka, T., Suzuki, M., Takeda, M., Iwata, N. and Ozaki, N. (2012) Common variants in MAGI2 gene are associated with increased risk for cognitive impairment in schizophrenic patients. PLoS One, 7, e36836. doi:10.1371/journal.pone.0036836 [183] International Schizophrenia Consortium. (2008) Rare chromosomal deletions and duplications increase risk of schizophrenia. Nature, 455, 237-241. doi:10.1038/nature07239 [184] Stefansson, H., Ophoff, R.A., Steinberg, S., Andreassen, O.A., Cichon, S., Rujescu, D., Werge, T., Pietiläinen, O.P., Mors, O., Mortensen, P.B., Sigurdsson, E., Gustafsson, O., Nyegaard, M., Tuulio-Henriksson, A., Ingason, A., Hansen, T., Suvisaari, J., Lonnqvist, J., Paunio, T., Børglum, A.D., Hartmann, A., Fink-Jensen, A., Nordentoft, M., Hougaard, D., Norgaard-Pedersen, B., Böttcher, Y., Olesen, J., Breuer, R., Möller, H.J., Gieg- ling, I., Rasmussen, H.B., Timm, S., Mattheisen, M., Bit- ter, I., Réthelyi, J.M., Magnusdottir, B.B., Sigmundsson, T., Olason, P., Masson, G., Gulcher, J.R., Haraldsson, M., Fossdal, R., Thorgeirsson, T.E., Thorsteinsdottir, U., Ruggeri, M., Tosato, S., Franke, B., Strengman, E., Kie- meney, L.A.; Genetic Risk and Outcome in Psychosis (GROUP), Melle, I., Djurovic, S., Abramova, L., Kaleda, V., Sanjuan, J., de Frutos R., Bramon, E., Vassos, E., Fraser, G., Ettinger, U., Picchioni, M., Walker, N., Tou- lopoulou, T., Need, A.C., Ge, D., Yoon, J.L., Shianna, K.V., Freimer, N.B., Cantor, R.M., Murray, R., Kong, A., Golimbet, V., Carracedo, A., Arango, C., Costas, J., Jönsson, E.G., Terenius, L., Agartz, I., Petursson, H., N?then, M.M., Rietschel, M., Matthews, P.M., Muglia, P., Peltonen, L., St Clair D., Goldstein, D.B., Stefansson, K. and Collier, D. A. (2009) Common variants conferring risk of schizophrenia. Nature, 460, 744-747. http://www.ncbi.nlm.nih.gov/pubmed/19571808 [185] Lee, B.D., Walss-Bass, C., Thompson, P.M., Dassori, A., Montero, P.A., Medina, R., Contreras, S., Armas, R., Ramirez, M., Pereira, M., Salazar, R., Leach, R.J, Qu- ezada, P., Raventos, H. and Escamilla, M.A. (2007) Ma- lic enzyme 2 and susceptibility to psychosis and mania. Psychiatry Researchearch, 150, 1-11. doi:10.1016/j.psychres.2006.06.001 [186] Kucukali, C.I., Aydin, M., Ozkok, E., Bilge, E., Orhan, N., Zengin, A. and Kara, I. (2009) Do schizophrenia and bipolar disorders share a common disease susceptibility variant at the MMP3 gene? Progress in Neuro-Psycho- pharmacology and Biological Psychiatry, 33, 557-561. doi:10.1016/j.pnpbp.2009.02.012 [187] Rybakowski, J.K., Skibinska, M., Leszczynska-Rodzie- wicz, A., Kaczmarek, L. and Hauser, J. (2009) Matrix metalloproteinase-9 gene and bipolar mood disorder. NeuroMolecular Medicineicine, 11, 128-132. doi:10.1007/s12017-009-8072-3 [188] Djurovic, S., Le Hellard, S., Kähler, A.K., Jönsson, E.G., Agartz, I., Steen, V.M., Hall, H., Wang, A.G., Rasmussen, H.B., Melle, I., Werge, T. and Andreassen, O.A. (2009) Association of MCTP2 gene variants with schizophrenia in three independent samples of Scandinavian origin (SCOPE). Psychiatry Researchearch, 168, 256-258. doi:10.1016/j.psychres.2008.08.007 [189] Feng, L.G., Song, Z.W., Xin, F, and Hu, J. (2009) Asso- ciation of plasma homocysteine and methylenetetrahy- drofolate reductase C677T gene variant with schizophre- nia: A Chinese Han population-based case-control study. Psychiatry Research, 168, 205-208. doi:10.1016/j.psychres.2008.05.009 [190] Zhang, C., Xie, B., Du, Y., Cheng, W., Fang, Y. and Yu, S. (2010) Further evidence that methylenetetrahydro- folate reductase A1298C polymorphism is a risk factor for schizophrenia. Journal of Neural Transmission, 117, 1115-1117. doi:10.1007/s00702-010-0442-3 [191] Kähler, A.K., Djurovic, S., Kulle, B., Jönsson, E.G., Agartz, I., Hall, H., Opjordsmoen, S., Jakobsen, K.D., Hansen, T., Melle, I., Werge, T., Steen, V.M. and Andreassen, O.A. (2008) Association analysis of schizophrenia on 18 genes involved in neuronal migration: MDGA1 as a new sus- ceptibility gene. American Journal of Medical Genetics, Part B, Neuropsychiatric Genetics, 147B, 1089-1100. http://www.ncbi.nlm.nih.gov/pubmed/18384059 [192] Li, J., Liu, J., Feng, G., Li, T., Zhao, Q., Li, Y., Hu, Z., Zheng, L., Zeng, Z., He, L., Wang, T. and Shi, Y. (2011) The MDGA1 gene confers risk to schizophrenia and bi- polar disorders. Schizophrenia Research, 125, 194-200. doi:10.1016/j.schres.2010.11.002 [193] Sorce, S., Schiavone, S., Tucci, P., Colaianna, M., Jaquet, V., Cuomo, V., Dubois-Dauphin, M., Trabace, L. and Krause, K.H. (2010) The NADPH oxidase NOX2 con- trols glutamate release: A novel mechanism involved in psychosis-like ketamine responses. The Journal of Neu- roscience, 30, 11317-11325. doi:10.1523/JNEUROSCI.1491-10.2010 [194] Gibbons, A.S., Thomas, E.A. and Dean, B. (2009) Re- gional and duration of illness differences in the alteration Copyright © 2013 SciRes. OPEN ACCESS
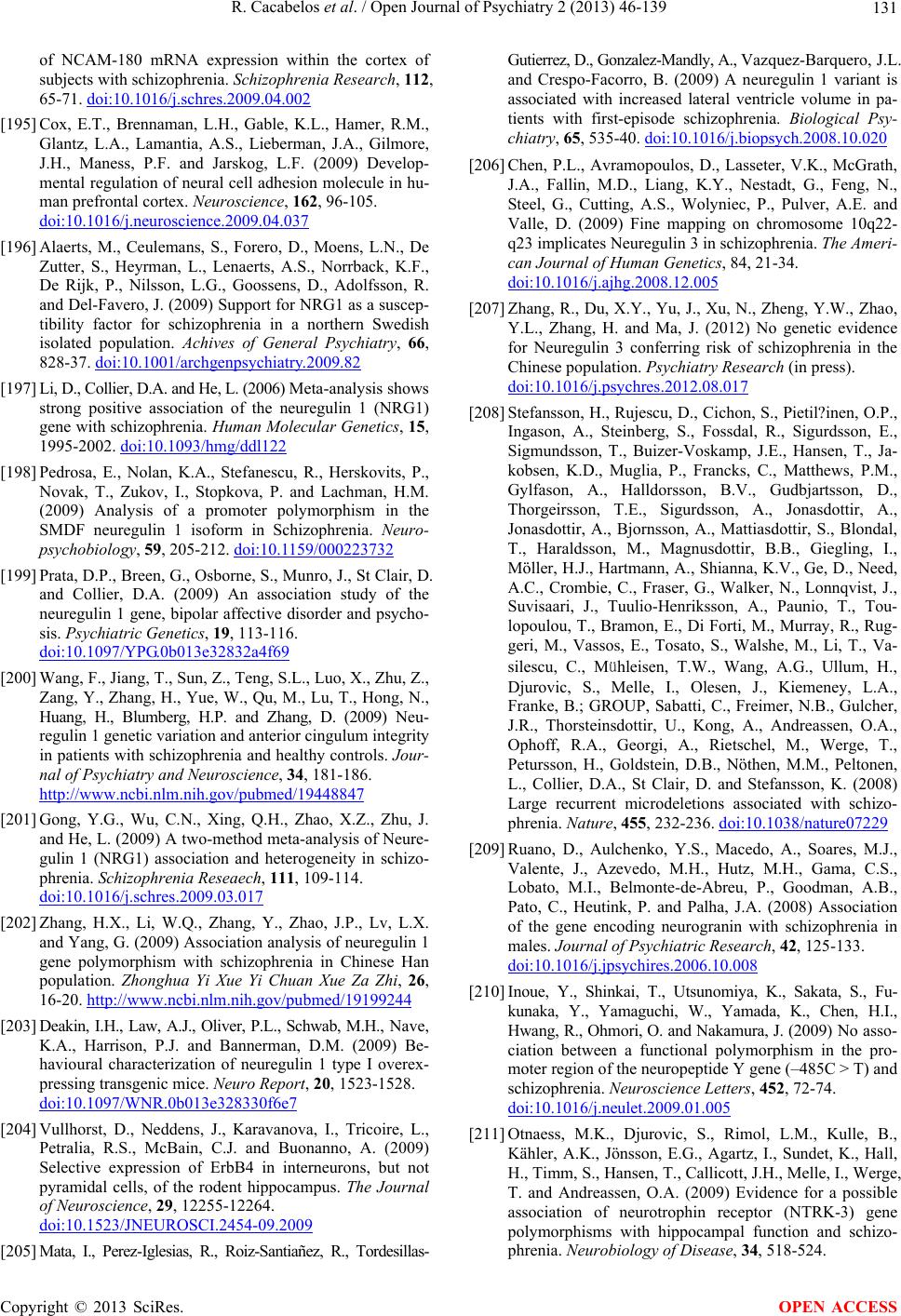 R. Cacabelos et al. / Open Journal of Psychiatry 2 (2013) 46-139 131 of NCAM-180 mRNA expression within the cortex of subjects with schizophrenia. Schizophrenia Research, 112, 65-71. doi:10.1016/j.schres.2009.04.002 [195] Cox, E.T., Brennaman, L.H., Gable, K.L., Hamer, R.M., Glantz, L.A., Lamantia, A.S., Lieberman, J.A., Gilmore, J.H., Maness, P.F. and Jarskog, L.F. (2009) Develop- mental regulation of neural cell adhesion molecule in hu- man prefrontal cortex. Neuroscience, 162, 96-105. doi:10.1016/j.neuroscience.2009.04.037 [196] Alaerts, M., Ceulemans, S., Forero, D., Moens, L.N., De Zutter, S., Heyrman, L., Lenaerts, A.S., Norrback, K.F., De Rijk, P., Nilsson, L.G., Goossens, D., Adolfsson, R. and Del-Favero, J. (2009) Support for NRG1 as a suscep- tibility factor for schizophrenia in a northern Swedish isolated population. Achives of General Psychiatry, 66, 828-37. doi:10.1001/archgenpsychiatry.2009.82 [197] Li, D., Collier, D.A. and He, L. (2006) Meta-analysis shows strong positive association of the neuregulin 1 (NRG1) gene with schizophrenia. Human Molecular Genetics, 15, 1995-2002. doi:10.1093/hmg/ddl122 [198] Pedrosa, E., Nolan, K.A., Stefanescu, R., Herskovits, P., Novak, T., Zukov, I., Stopkova, P. and Lachman, H.M. (2009) Analysis of a promoter polymorphism in the SMDF neuregulin 1 isoform in Schizophrenia. Neuro- psychobiology, 59, 205-212. doi:10.1159/000223732 [199] Prata, D.P., Breen, G., Osborne, S., Munro, J., St Clair, D. and Collier, D.A. (2009) An association study of the neuregulin 1 gene, bipolar affective disorder and psycho- sis. Psychiatric Genetics, 19, 113-116. doi:10.1097/YPG.0b013e32832a4f69 [200] Wang, F., Jiang, T., Sun, Z., Teng, S.L., Luo, X., Zhu, Z., Zang, Y., Zhang, H., Yue, W., Qu, M., Lu, T., Hong, N., Huang, H., Blumberg, H.P. and Zhang, D. (2009) Neu- regulin 1 genetic variation and anterior cingulum integrity in patients with schizophrenia and healthy controls. Jour- nal of Psychiatry and Neuroscience, 34, 181-186. http://www.ncbi.nlm.nih.gov/pubmed/19448847 [201] Gong, Y.G., Wu, C.N., Xing, Q.H., Zhao, X.Z., Zhu, J. and He, L. (2009) A two-method meta-analysis of Neure- gulin 1 (NRG1) association and heterogeneity in schizo- phrenia. Schizophrenia Reseaech, 111, 109-114. doi:10.1016/j.schres.2009.03.017 [202] Zhang, H.X., Li, W.Q., Zhang, Y., Zhao, J.P., Lv, L.X. and Yang, G. (2009) Association analysis of neuregulin 1 gene polymorphism with schizophrenia in Chinese Han population. Zhonghua Yi Xue Yi Chuan Xue Za Zhi, 26, 16-20. http://www.ncbi.nlm.nih.gov/pubmed/19199244 [203] Deakin, I.H., Law, A.J., Oliver, P.L., Schwab, M.H., Nave, K.A., Harrison, P.J. and Bannerman, D.M. (2009) Be- havioural characterization of neuregulin 1 type I overex- pressing transgenic mice. Neuro Report, 20, 1523-1528. doi:10.1097/WNR.0b013e328330f6e7 [204] Vullhorst, D., Neddens, J., Karavanova, I., Tricoire, L., Petralia, R.S., McBain, C.J. and Buonanno, A. (2009) Selective expression of ErbB4 in interneurons, but not pyramidal cells, of the rodent hippocampus. The Journal of Neuroscience, 29, 12255-12264. doi:10.1523/JNEUROSCI.2454-09.2009 [205] Mata, I., Perez-Iglesias, R., Roiz-Santiañez, R., Tordesillas- Gutierrez, D., Gonzalez-Mandly, A., Vazquez-Barquero, J.L. and Crespo-Facorro, B. (2009) A neuregulin 1 variant is associated with increased lateral ventricle volume in pa- tients with first-episode schizophrenia. Biological Psy- chiatry, 65, 535-40. doi:10.1016/j.biopsych.2008.10.020 [206] Chen, P.L., Avramopoulos, D., Lasseter, V.K., McGrath, J.A., Fallin, M.D., Liang, K.Y., Nestadt, G., Feng, N., Steel, G., Cutting, A.S., Wolyniec, P., Pulver, A.E. and Valle, D. (2009) Fine mapping on chromosome 10q22- q23 implicates Neuregulin 3 in schizophrenia. The Ameri- can Journal of Human Genetics, 84, 21-34. doi:10.1016/j.ajhg.2008.12.005 [207] Zhang, R., Du, X.Y., Yu, J., Xu, N., Zheng, Y.W., Zhao, Y.L., Zhang, H. and Ma, J. (2012) No genetic evidence for Neuregulin 3 conferring risk of schizophrenia in the Chinese population. Psychiatry Research (in press). doi:10.1016/j.psychres.2012.08.017 [208] Stefansson, H., Rujescu, D., Cichon, S., Pietil?inen, O.P., Ingason, A., Steinberg, S., Fossdal, R., Sigurdsson, E., Sigmundsson, T., Buizer-Voskamp, J.E., Hansen, T., Ja- kobsen, K.D., Muglia, P., Francks, C., Matthews, P.M., Gylfason, A., Halldorsson, B.V., Gudbjartsson, D., Thorgeirsson, T.E., Sigurdsson, A., Jonasdottir, A., Jonasdottir, A., Bjornsson, A., Mattiasdottir, S., Blondal, T., Haraldsson, M., Magnusdottir, B.B., Giegling, I., Möller, H.J., Hartmann, A., Shianna, K.V., Ge, D., Need, A.C., Crombie, C., Fraser, G., Walker, N., Lonnqvist, J., Suvisaari, J., Tuulio-Henriksson, A., Paunio, T., Tou- lopoulou, T., Bramon, E., Di Forti, M., Murray, R., Rug- geri, M., Vassos, E., Tosato, S., Walshe, M., Li, T., Va- silescu, C., Mühleisen, T.W., Wang, A.G., Ullum, H., Djurovic, S., Melle, I., Olesen, J., Kiemeney, L.A., Franke, B.; GROUP, Sabatti, C., Freimer, N.B., Gulcher, J.R., Thorsteinsdottir, U., Kong, A., Andreassen, O.A., Ophoff, R.A., Georgi, A., Rietschel, M., Werge, T., Petursson, H., Goldstein, D.B., Nöthen, M.M., Peltonen, L., Collier, D.A., St Clair, D. and Stefansson, K. (2008) Large recurrent microdeletions associated with schizo- phrenia. Nature, 455, 232-236. doi:10.1038/nature07229 [209] Ruano, D., Aulchenko, Y.S., Macedo, A., Soares, M.J., Valente, J., Azevedo, M.H., Hutz, M.H., Gama, C.S., Lobato, M.I., Belmonte-de-Abreu, P., Goodman, A.B., Pato, C., Heutink, P. and Palha, J.A. (2008) Association of the gene encoding neurogranin with schizophrenia in males. Journal of Psychiatric Research, 42, 125-133. doi:10.1016/j.jpsychires.2006.10.008 [210] Inoue, Y., Shinkai, T., Utsunomiya, K., Sakata, S., Fu- kunaka, Y., Yamaguchi, W., Yamada, K., Chen, H.I., Hwang, R., Ohmori, O. and Nakamura, J. (2009) No asso- ciation between a functional polymorphism in the pro- moter region of the neuropeptide Y gene (–485C > T) and schizophrenia. Neuroscience Letters, 452, 72-74. doi:10.1016/j.neulet.2009.01.005 [211] Otnaess, M.K., Djurovic, S., Rimol, L.M., Kulle, B., Kähler, A.K., Jönsson, E.G., Agartz, I., Sundet, K., Hall, H., Timm, S., Hansen, T., Callicott, J.H., Melle, I., Werge, T. and Andreassen, O.A. (2009) Evidence for a possible association of neurotrophin receptor (NTRK-3) gene polymorphisms with hippocampal function and schizo- phrenia. Neurobiology of Disease, 34, 518-524. Copyright © 2013 SciRes. OPEN ACCESS
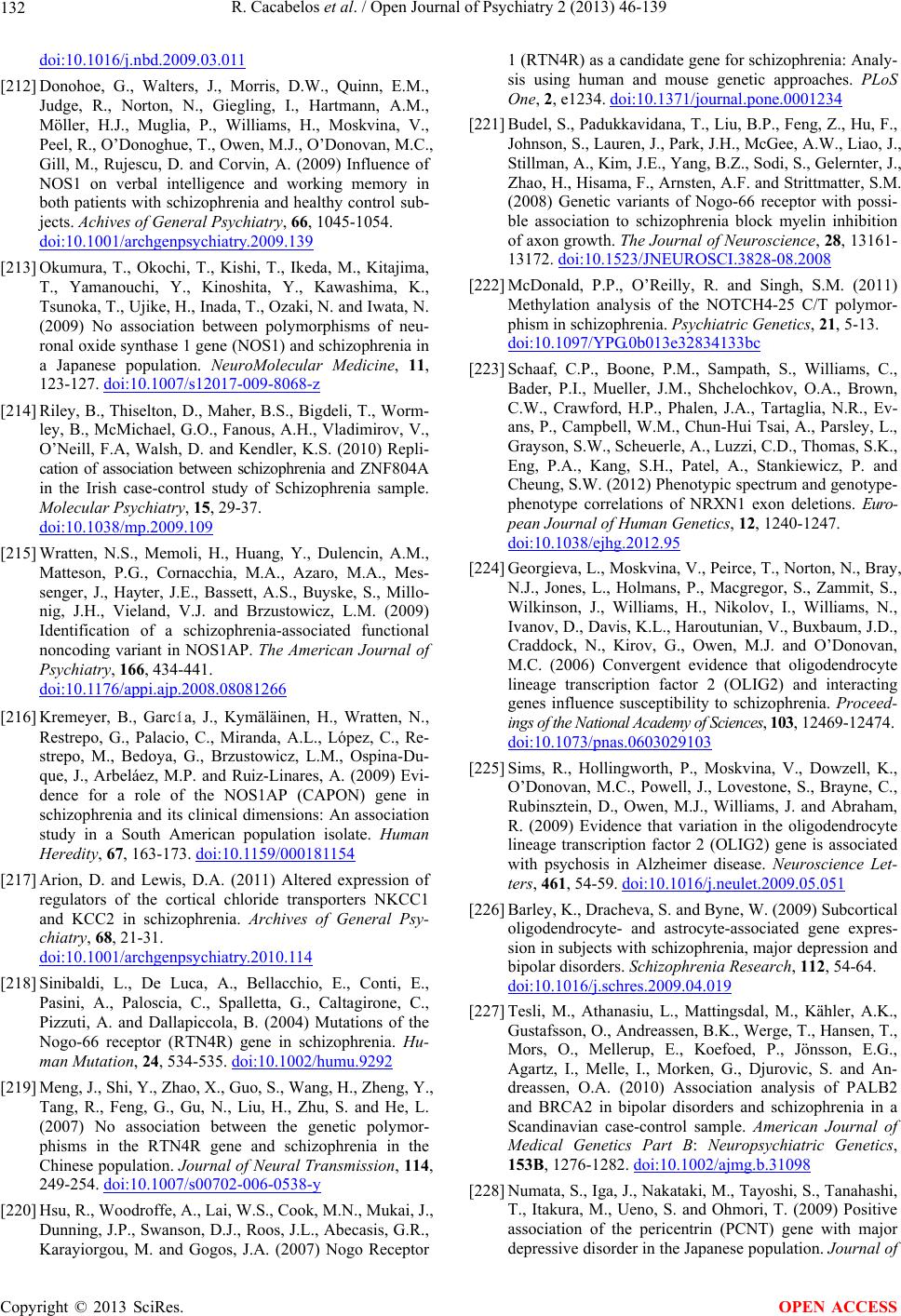 R. Cacabelos et al. / Open Journal of Psychiatry 2 (2013) 46-139 132 doi:10.1016/j.nbd.2009.03.011 [212] Donohoe, G., Walters, J., Morris, D.W., Quinn, E.M., Judge, R., Norton, N., Giegling, I., Hartmann, A.M., Möller, H.J., Muglia, P., Williams, H., Moskvina, V., Peel, R., O’Donoghue, T., Owen, M.J., O’Donovan, M.C., Gill, M., Rujescu, D. and Corvin, A. (2009) Influence of NOS1 on verbal intelligence and working memory in both patients with schizophrenia and healthy control sub- jects. Achives of General Psychiatry, 66, 1045-1054. doi:10.1001/archgenpsychiatry.2009.139 [213] Okumura, T., Okochi, T., Kishi, T., Ikeda, M., Kitajima, T., Yamanouchi, Y., Kinoshita, Y., Kawashima, K., Tsunoka, T., Ujike, H., Inada, T., Ozaki, N. and Iwata, N. (2009) No association between polymorphisms of neu- ronal oxide synthase 1 gene (NOS1) and schizophrenia in a Japanese population. NeuroMolecular Medicine, 11, 123-127. doi:10.1007/s12017-009-8068-z [214] Riley, B., Thiselton, D., Maher, B.S., Bigdeli, T., Worm- ley, B., McMichael, G.O., Fanous, A.H., Vladimirov, V., O’Neill, F.A, Walsh, D. and Kendler, K.S. (2010) Repli- cation of association between schizophrenia and ZNF804A in the Irish case-control study of Schizophrenia sample. Molecular Psychiatry, 15, 29-37. doi:10.1038/mp.2009.109 [215] Wratten, N.S., Memoli, H., Huang, Y., Dulencin, A.M., Matteson, P.G., Cornacchia, M.A., Azaro, M.A., Mes- senger, J., Hayter, J.E., Bassett, A.S., Buyske, S., Millo- nig, J.H., Vieland, V.J. and Brzustowicz, L.M. (2009) Identification of a schizophrenia-associated functional noncoding variant in NOS1AP. The American Journal of Psychiatry, 166, 434-441. doi:10.1176/appi.ajp.2008.08081266 [216] Kremeyer, B., García, J., Kymäläinen, H., Wratten, N., Restrepo, G., Palacio, C., Miranda, A.L., López, C., Re- strepo, M., Bedoya, G., Brzustowicz, L.M., Ospina-Du- que, J., Arbeláez, M.P. and Ruiz-Linares, A. (2009) Evi- dence for a role of the NOS1AP (CAPON) gene in schizophrenia and its clinical dimensions: An association study in a South American population isolate. Human Heredity, 67, 163-173. doi:10.1159/000181154 [217] Arion, D. and Lewis, D.A. (2011) Altered expression of regulators of the cortical chloride transporters NKCC1 and KCC2 in schizophrenia. Archives of General Psy- chiatry, 68, 21-31. doi:10.1001/archgenpsychiatry.2010.114 [218] Sinibaldi, L., De Luca, A., Bellacchio, E., Conti, E., Pasini, A., Paloscia, C., Spalletta, G., Caltagirone, C., Pizzuti, A. and Dallapiccola, B. (2004) Mutations of the Nogo-66 receptor (RTN4R) gene in schizophrenia. Hu- man Mutation, 24, 534-535. doi:10.1002/humu.9292 [219] Meng, J., Shi, Y., Zhao, X., Guo, S., Wang, H., Zheng, Y., Tang, R., Feng, G., Gu, N., Liu, H., Zhu, S. and He, L. (2007) No association between the genetic polymor- phisms in the RTN4R gene and schizophrenia in the Chinese population. Journal of Neural Transmission, 114, 249-254. doi:10.1007/s00702-006-0538-y [220] Hsu, R., Woodroffe, A., Lai, W.S., Cook, M.N., Mukai, J., Dunning, J.P., Swanson, D.J., Roos, J.L., Abecasis, G.R., Karayiorgou, M. and Gogos, J.A. (2007) Nogo Receptor 1 (RTN4R) as a candidate gene for schizophrenia: Analy- sis using human and mouse genetic approaches. PLoS One, 2, e1234. doi:10.1371/journal.pone.0001234 [221] Budel, S., Padukkavidana, T., Liu, B.P., Feng, Z., Hu, F., Johnson, S., Lauren, J., Park, J.H., McGee, A.W., Liao, J., Stillman, A., Kim, J.E., Yang, B.Z., Sodi, S., Gelernter, J., Zhao, H., Hisama, F., Arnsten, A.F. and Strittmatter, S.M. (2008) Genetic variants of Nogo-66 receptor with possi- ble association to schizophrenia block myelin inhibition of axon growth. The Journal of Neuroscience, 28, 13161- 13172. doi:10.1523/JNEUROSCI.3828-08.2008 [222] McDonald, P.P., O’Reilly, R. and Singh, S.M. (2011) Methylation analysis of the NOTCH4-25 C/T polymor- phism in schizophrenia. Psychiatric Genetics, 21, 5-13. doi:10.1097/YPG.0b013e32834133bc [223] Schaaf, C.P., Boone, P.M., Sampath, S., Williams, C., Bader, P.I., Mueller, J.M., Shchelochkov, O.A., Brown, C.W., Crawford, H.P., Phalen, J.A., Tartaglia, N.R., Ev- ans, P., Campbell, W.M., Chun-Hui Tsai, A., Parsley, L., Grayson, S.W., Scheuerle, A., Luzzi, C.D., Thomas, S.K., Eng, P.A., Kang, S.H., Patel, A., Stankiewicz, P. and Cheung, S.W. (2012) Phenotypic spectrum and genotype- phenotype correlations of NRXN1 exon deletions. Euro- pean Journal of Human Genetics, 12, 1240-1247. doi:10.1038/ejhg.2012.95 [224] Georgieva, L., Moskvina, V., Peirce, T., Norton, N., Bray, N.J., Jones, L., Holmans, P., Macgregor, S., Zammit, S., Wilkinson, J., Williams, H., Nikolov, I., Williams, N., Ivanov, D., Davis, K.L., Haroutunian, V., Buxbaum, J.D., Craddock, N., Kirov, G., Owen, M.J. and O’Donovan, M.C. (2006) Convergent evidence that oligodendrocyte lineage transcription factor 2 (OLIG2) and interacting genes influence susceptibility to schizophrenia. Proceed- ings of the National Academy of Sciences, 103, 12469-12474. doi:10.1073/pnas.0603029103 [225] Sims, R., Hollingworth, P., Moskvina, V., Dowzell, K., O’Donovan, M.C., Powell, J., Lovestone, S., Brayne, C., Rubinsztein, D., Owen, M.J., Williams, J. and Abraham, R. (2009) Evidence that variation in the oligodendrocyte lineage transcription factor 2 (OLIG2) gene is associated with psychosis in Alzheimer disease. Neuroscience Let- ters, 461, 54-59. doi:10.1016/j.neulet.2009.05.051 [226] Barley, K., Dracheva, S. and Byne, W. (2009) Subcortical oligodendrocyte- and astrocyte-associated gene expres- sion in subjects with schizophrenia, major depression and bipolar disorders. Schizophrenia Research, 112, 54-64. doi:10.1016/j.schres.2009.04.019 [227] Tesli, M., Athanasiu, L., Mattingsdal, M., Kähler, A.K., Gustafsson, O., Andreassen, B.K., Werge, T., Hansen, T., Mors, O., Mellerup, E., Koefoed, P., Jönsson, E.G., Agartz, I., Melle, I., Morken, G., Djurovic, S. and An- dreassen, O.A. (2010) Association analysis of PALB2 and BRCA2 in bipolar disorders and schizophrenia in a Scandinavian case-control sample. American Journal of Medical Genetics Part B: Neuropsychiatric Genetics, 153B, 1276-1282. doi:10.1002/ajmg.b.31098 [228] Numata, S., Iga, J., Nakataki, M., Tayoshi, S., Tanahashi, T., Itakura, M., Ueno, S. and Ohmori, T. (2009) Positive association of the pericentrin (PCNT) gene with major depressive disorder in the Japanese population. Journal of Copyright © 2013 SciRes. OPEN ACCESS
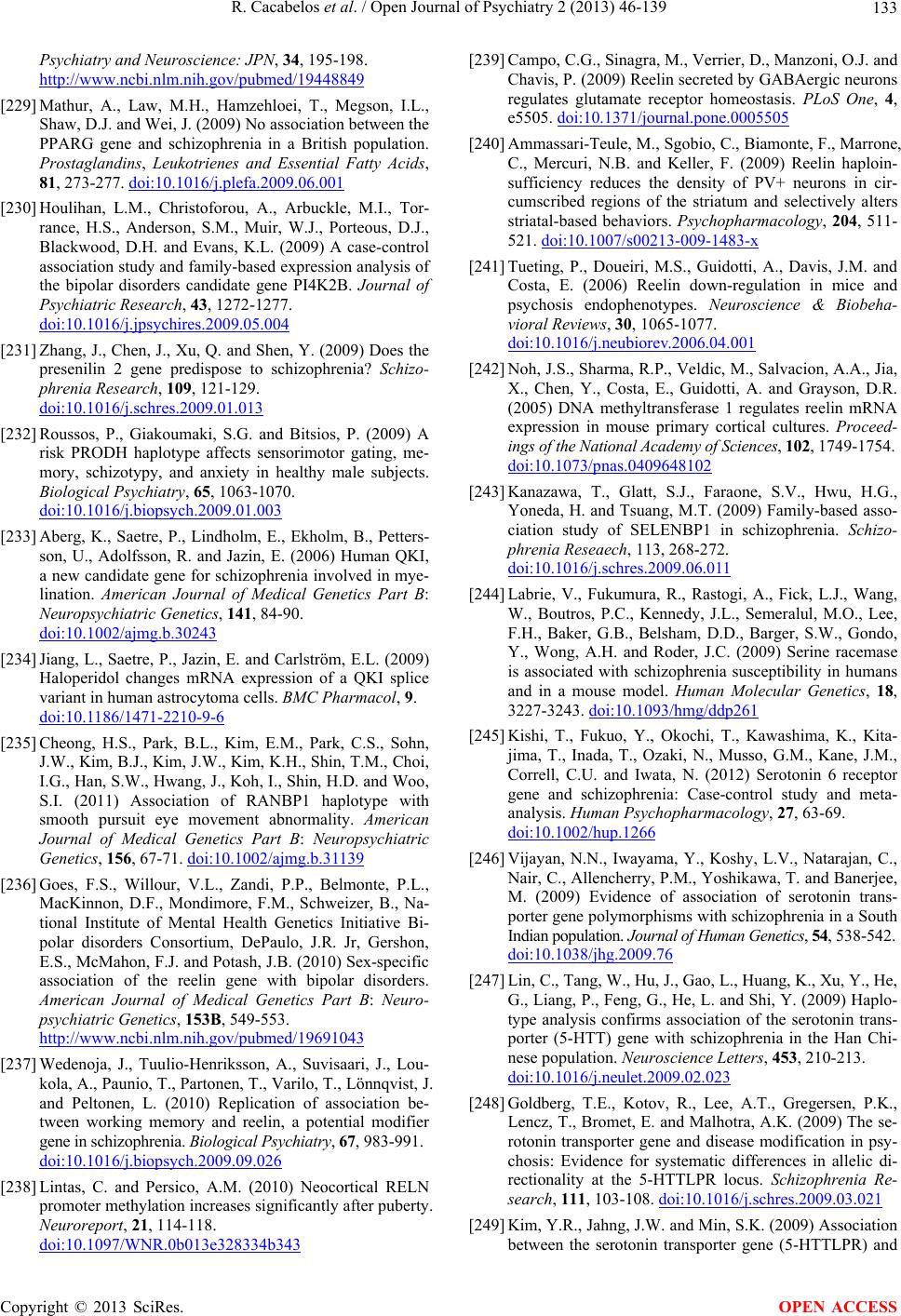 R. Cacabelos et al. / Open Journal of Psychiatry 2 (2013) 46-139 133 Psychiatry and Neuroscience: JPN, 34, 195-198. http://www.ncbi.nlm.nih.gov/pubmed/19448849 [229] Mathur, A., Law, M.H., Hamzehloei, T., Megson, I.L., Shaw, D.J. and Wei, J. (2009) No association between the PPARG gene and schizophrenia in a British population. Prostaglandins, Leukotrienes and Essential Fatty Acids, 81, 273-277. doi:10.1016/j.plefa.2009.06.001 [230] Houlihan, L.M., Christoforou, A., Arbuckle, M.I., Tor- rance, H.S., Anderson, S.M., Muir, W.J., Porteous, D.J., Blackwood, D.H. and Evans, K.L. (2009) A case-control association study and family-based expression analysis of the bipolar disorders candidate gene PI4K2B. Journal of Psychiatric Research, 43, 1272-1277. doi:10.1016/j.jpsychires.2009.05.004 [231] Zhang, J., Chen, J., Xu, Q. and Shen, Y. (2009) Does the presenilin 2 gene predispose to schizophrenia? Schizo- phrenia Research, 109, 121-129. doi:10.1016/j.schres.2009.01.013 [232] Roussos, P., Giakoumaki, S.G. and Bitsios, P. (2009) A risk PRODH haplotype affects sensorimotor gating, me- mory, schizotypy, and anxiety in healthy male subjects. Biological Psychiatry, 65, 1063-1070. doi:10.1016/j.biopsych.2009.01.003 [233] Aberg, K., Saetre, P., Lindholm, E., Ekholm, B., Petters- son, U., Adolfsson, R. and Jazin, E. (2006) Human QKI, a new candidate gene for schizophrenia involved in mye- lination. American Journal of Medical Genetics Part B: Neuropsychiatric Genetics, 141, 84-90. doi:10.1002/ajmg.b.30243 [234] Jiang, L., Saetre, P., Jazin, E. and Carlström, E.L. (2009) Haloperidol changes mRNA expression of a QKI splice variant in human astrocytoma cells. BMC Pharmacol, 9. doi:10.1186/1471-2210-9-6 [235] Cheong, H.S., Park, B.L., Kim, E.M., Park, C.S., Sohn, J.W., Kim, B.J., Kim, J.W., Kim, K.H., Shin, T.M., Choi, I.G., Han, S.W., Hwang, J., Koh, I., Shin, H.D. and Woo, S.I. (2011) Association of RANBP1 haplotype with smooth pursuit eye movement abnormality. American Journal of Medical Genetics Part B: Neuropsychiatric Genetics, 156, 67-71. doi:10.1002/ajmg.b.31139 [236] Goes, F.S., Willour, V.L., Zandi, P.P., Belmonte, P.L., MacKinnon, D.F., Mondimore, F.M., Schweizer, B., Na- tional Institute of Mental Health Genetics Initiative Bi- polar disorders Consortium, DePaulo, J.R. Jr, Gershon, E.S., McMahon, F.J. and Potash, J.B. (2010) Sex-specific association of the reelin gene with bipolar disorders. American Journal of Medical Genetics Part B: Neuro- psychiatric Genetics, 153B, 549-553. http://www.ncbi.nlm.nih.gov/pubmed/19691043 [237] Wedenoja, J., Tuulio-Henriksson, A., Suvisaari, J., Lou- kola, A., Paunio, T., Partonen, T., Varilo, T., Lönnqvist, J. and Peltonen, L. (2010) Replication of association be- tween working memory and reelin, a potential modifier gene in schizophrenia. Biological Psychiatry, 67, 983-991. doi:10.1016/j.biopsych.2009.09.026 [238] Lintas, C. and Persico, A.M. (2010) Neocortical RELN promoter methylation increases significantly after puberty. Neuroreport, 21, 114-118. doi:10.1097/WNR.0b013e328334b343 [239] Campo, C.G., Sinagra, M., Verrier, D., Manzoni, O.J. and Chavis, P. (2009) Reelin secreted by GABAergic neurons regulates glutamate receptor homeostasis. PLoS One, 4, e5505. doi:10.1371/journal.pone.0005505 [240] Ammassari-Teule, M., Sgobio, C., Biamonte, F., Marrone, C., Mercuri, N.B. and Keller, F. (2009) Reelin haploin- sufficiency reduces the density of PV+ neurons in cir- cumscribed regions of the striatum and selectively alters striatal-based behaviors. Psychopharmacology, 204, 511- 521. doi:10.1007/s00213-009-1483-x [241] Tueting, P., Doueiri, M.S., Guidotti, A., Davis, J.M. and Costa, E. (2006) Reelin down-regulation in mice and psychosis endophenotypes. Neuroscience & Biobeha- vioral Reviews, 30, 1065-1077. doi:10.1016/j.neubiorev.2006.04.001 [242] Noh, J.S., Sharma, R.P., Veldic, M., Salvacion, A.A., Jia, X., Chen, Y., Costa, E., Guidotti, A. and Grayson, D.R. (2005) DNA methyltransferase 1 regulates reelin mRNA expression in mouse primary cortical cultures. Proceed- ings of the National Academy of Sciences, 102, 1749-1754. doi:10.1073/pnas.0409648102 [243] Kanazawa, T., Glatt, S.J., Faraone, S.V., Hwu, H.G., Yoneda, H. and Tsuang, M.T. (2009) Family-based asso- ciation study of SELENBP1 in schizophrenia. Schizo- phrenia Reseaech, 113, 268-272. doi:10.1016/j.schres.2009.06.011 [244] Labrie, V., Fukumura, R., Rastogi, A., Fick, L.J., Wang, W., Boutros, P.C., Kennedy, J.L., Semeralul, M.O., Lee, F.H., Baker, G.B., Belsham, D.D., Barger, S.W., Gondo, Y., Wong, A.H. and Roder, J.C. (2009) Serine racemase is associated with schizophrenia susceptibility in humans and in a mouse model. Human Molecular Genetics, 18, 3227-3243. doi:10.1093/hmg/ddp261 [245] Kishi, T., Fukuo, Y., Okochi, T., Kawashima, K., Kita- jima, T., Inada, T., Ozaki, N., Musso, G.M., Kane, J.M., Correll, C.U. and Iwata, N. (2012) Serotonin 6 receptor gene and schizophrenia: Case-control study and meta- analysis. Human Psychopharmacology, 27, 63-69. doi:10.1002/hup.1266 [246] Vijayan, N.N., Iwayama, Y., Koshy, L.V., Natarajan, C., Nair, C., Allencherry, P.M., Yoshikawa, T. and Banerjee, M. (2009) Evidence of association of serotonin trans- porter gene polymorphisms with schizophrenia in a South Indian population. Journal of Human Genetics, 54, 538-542. doi:10.1038/jhg.2009.76 [247] Lin, C., Tang, W., Hu, J., Gao, L., Huang, K., Xu, Y., He, G., Liang, P., Feng, G., He, L. and Shi, Y. (2009) Haplo- type analysis confirms association of the serotonin trans- porter (5-HTT) gene with schizophrenia in the Han Chi- nese population. Neuroscience Letters, 453, 210-213. doi:10.1016/j.neulet.2009.02.023 [248] Goldberg, T.E., Kotov, R., Lee, A.T., Gregersen, P.K., Lencz, T., Bromet, E. and Malhotra, A.K. (2009) The se- rotonin transporter gene and disease modification in psy- chosis: Evidence for systematic differences in allelic di- rectionality at the 5-HTTLPR locus. Schizophrenia Re- search, 111, 103-108. doi:10.1016/j.schres.2009.03.021 [249] Kim, Y.R., Jahng, J.W. and Min, S.K. (2009) Association between the serotonin transporter gene (5-HTTLPR) and Copyright © 2013 SciRes. OPEN ACCESS
 R. Cacabelos et al. / Open Journal of Psychiatry 2 (2013) 46-139 134 anger-related traits in Korean schizophrenic patients. Neuropsychobiology, 59, 165-171. doi:10.1159/000218079 [250] Bachmann-Gagescu, R., Mefford, H.C., Cowan, C., Glew, G.M., Hing, A.V., Wallace, S., Bader, P.I., Hamati, A., Reitnauer, P.J., Smith, R., Stockton, D.W., Muhle, H., Helbig, I., Eichler, E.E., Ballif, B.C., Rosenfeld, J. and Tsuchiya, K.D. (2010) Recurrent 200-kb deletions of 16p11.2 that include the SH2B1 gene are associated with developmental delay and obesity. Genetics in Medicine, 12, 641-647. doi:10.1097/GIM.0b013e3181ef4286 [251] Maekawa, M., Ohnishi, T., Hashimoto, K., Watanabe, A., Iwayama, Y., Ohba, H., Hattori, E., Yamada, K. and Yo- shikawa, T. (2010) Analysis of strain-dependent prepulse inhibition points to a role for Shmt1 (SHMT1) in mice and in schizophrenia. Journal of Neurochemistry, 115, 1374-1385. doi :1 0. 1111/j.1471-4159.2010.07039.x [252] McAuley, E.Z., Scimone, A., Tiwari, Y., Agahi, G., Mowry, B.J., Holliday, E.G., Donald, J.A., Weickert, C.S., Mitchell, P.B., Schofield, P.R. and Fullerton, J.M. (2012) Identification of sialyltransferase 8B as a general- ized susceptibility gene for psychotic and mood disorders on chromosome 15q25-26. PLoS One, 7, e38172. doi:10.1371/journal.pone.0038172 [253] Takizawa, R., Hashimoto, K., Tochigi, M., Kawakubo, Y., Marumo, K., Sasaki, T., Fukuda, M. and Kasai, K. (2009) Association between sigma-1 receptor gene polymor- phism and prefrontal hemodynamic response induced by cognitive activation in schizophrenia. Progress in Neuro- Psychopharmacology and Biological Psychiatry, 33, 491-498. doi:10.1016/j.pnpbp.2009.01.014 [254] Cordeiro, Q., Siqueira-Roberto, J. and Vallada, H. (2010) Association between the SLC6A3 A1343G polymorphism and schizophrenia. Arquivos de Neuropsiquiatria, 68, 716- 719. doi:10.1590/S0004-282X2010000500008 [255] Koga, M., Ishiguro, H., Yazaki, S., Horiuchi, Y., Arai, M., Niizato, K., Iritani, S., Itokawa, M., Inada, T., Iwata, N., Ozaki, N., Ujike, H., Kunugi, H., Sasaki, T., Takahashi, M., Watanabe, Y., Someya, T., Kakita, A., Takahashi, H., Nawa, H., Muchardt, C., Yaniv, M. and Arinami, T. (2009) Involvement of SMARCA2/BRM in the SWI/SNF chromatin-remodeling complex in schizophrenia. Human Molecular Genetics, 18, 2483-2494. doi:10.1093/hmg/ddp166 [256] Yuan, A., Yi, Z., Sun, J., Du, Y., Yu, T., Zhang, C., Liu, Y., Zhou, Y., Liu, D., Li, H., Xu, Y., Cheng, Z., Li, W. and Yu, S. (2012) Effect of SOX10 gene polymorphism on early onset schizophrenia in Chinese Han population. Neuroscience Letters, 521, 93-97. doi:10.1016/j.neulet.2012.05.040 [257] Zhou, X., Tang, W., Greenwood, T.A., Guo, S., He, L., Geyer, M.A. and Kelsoe, J.R. (2009) Transcription factor SP4 is a susceptibility gene for bipolar disorders. PLoS One, 4, e5196. doi:10.1371/journal.pone.0005196 [258] Bermudo-Soriano, C.R., Vaquero-Lorenzo, C., Diaz-Hernandez, M., Perez-Rodriguez, M.M., Fernandez-Piqueras, J., Saiz-Ruiz, J. and Baca-Garcia E. (2009) SAT-1-1415T/C polymorphism and susceptibility to schizophrenia. Pro- gress in Neuro-Psychopharmacol Biological Psychiatry, 33, 345-348. doi:10.1016/j.pnpbp.2008.12.015 [259] Lewis, A.G. and Minchin, R.F. (2009) Lack of exonic sulfotransferase 4A1 mutations in controls and schizo- phrenia cases. Psychiatric Genetics, 19, 53-55. doi:10.1097/YPG.0b013e3283118776 [260] Chen, Q., Che, R., Wang, X., O’Neill, F.A., Walsh, D., Tang, W., Shi, Y., He, L., Kendler, K.S. and Chen, X. (2009) Association and expression study of synapsin III and schizophrenia. Neuroscience Letters, 465, 248-251. doi:10.1016/j.neulet.2009.09.032 [261] Murphy, B.C., O’Reilly, R.L. and Singh, S.M. (2008) DNA methylation and mRNA expression of SYN III, a candidate gene for schizophrenia. BMC Medical Genetics, 9, 115. doi:10.1186/1471-2350-9-115 [262] Iatropoulos, P., Gardella, R., Valsecchi, P., Magri, C., Ratti, C., Podavini, D., Rossi, G., Gennarelli, M., Sac- chetti, E. and Barlati, S. (2009) Association study and mutational screening of SYNGR1 as a candidate suscep- tibility gene for schizophrenia. Psychiatric Genetics, 19, 237-243. doi:10.1097/YPG.0b013e32832cebf7 [263] Wang, Y., Yu, L., Zhao, T., Xu, J., Liu, Z., Liu, Y., Feng, G., He, L. and Li, S. (2009) No association between bi- polar disorders and syngr1 or synapsin II polymorphisms in the Han Chinese population. Psychiatry Research, 169, 167-168. doi:10.1016/j.psychres.2008.12.005 [264] Corradini, I., Verderio, C., Sala, M., Wilson, M.C. and Matteoli, M. (2009) SNAP-25 in neuropsychiatric disor- ders. Annals of the New York Academy of Sciences, 1152, 93-99. d oi :1 0. 1111/j. 1749-6632.2008.03995.x [265] McKee, A.G., Loscher, J.S., O’Sullivan, N.C., Chadderton, N., Palfi, A., Batti, L., Sheridan, G.K., O’Shea, S., Moran, M., McCabe, O., Fernández, A.B., Pangalos, M.N., O’Con- nor, J.J., Regan, C.M., O’Connor, W.T., Humphries, P., Farrar, G.J. and Murphy, K.J. (2009) AAV-mediated chronic over-expression of SNAP-25 in adult rat dorsal hippocampus impairs memory-associated synaptic plas- ticity. Journal of Neurochemistry, 112, 991-1004. doi:10.1111/j.1 471 -4159.2009.06516.x [266] Fellerhoff, B. and Wank, R. (2009) Transporter associ- ated with antigen processing and the chaperone tapasin: Are non-classical HLA genes keys to the pathogenesis of schizophrenia? Medical Hypotheses, 72, 535-538. doi:10.1016/j.mehy.2008.12.036 [267] Ohi, K., Hashimoto, R., Yasuda, Y., Kiribayashi, M., Iike, N., Yoshida, T., Azechi, M., Ikezawa, K., Takahashi, H., Morihara, T., Ishii, R., Tagami, S., Iwase, M., Okochi, M., Kamino, K., Kazui, H., Tanaka, T., Kudo, T. and Takeda, M. (2009) TATA box-binding protein gene is associated with risk for schizophrenia, age at onset and prefrontal function. Genes, Brain and Behavior, 8, 473-480. doi:10.1111/j.1 601 -183X.2009.00497.x [268] Geser, F., Robinson, J.L., Malunda, J.A., Xie, S.X., Clark, C.M., Kwong, L.K., Moberg, P.J., Moore, E.M., Van Deerlin, V.M., Lee, V.M., Arnold, S.E. and Trojanowski, J.Q. (2010) Pathological 43-kDa transactivation response DNA-binding protein in older adults with and without severe mental illness. Archives of Neurology, 67, 1238-1250. doi:10.1001/archneurol.2010.254 [269] Wirgenes, K.V., Sønderby, I.E., Haukvik, U.K., Mat- Copyright © 2013 SciRes. OPEN ACCESS
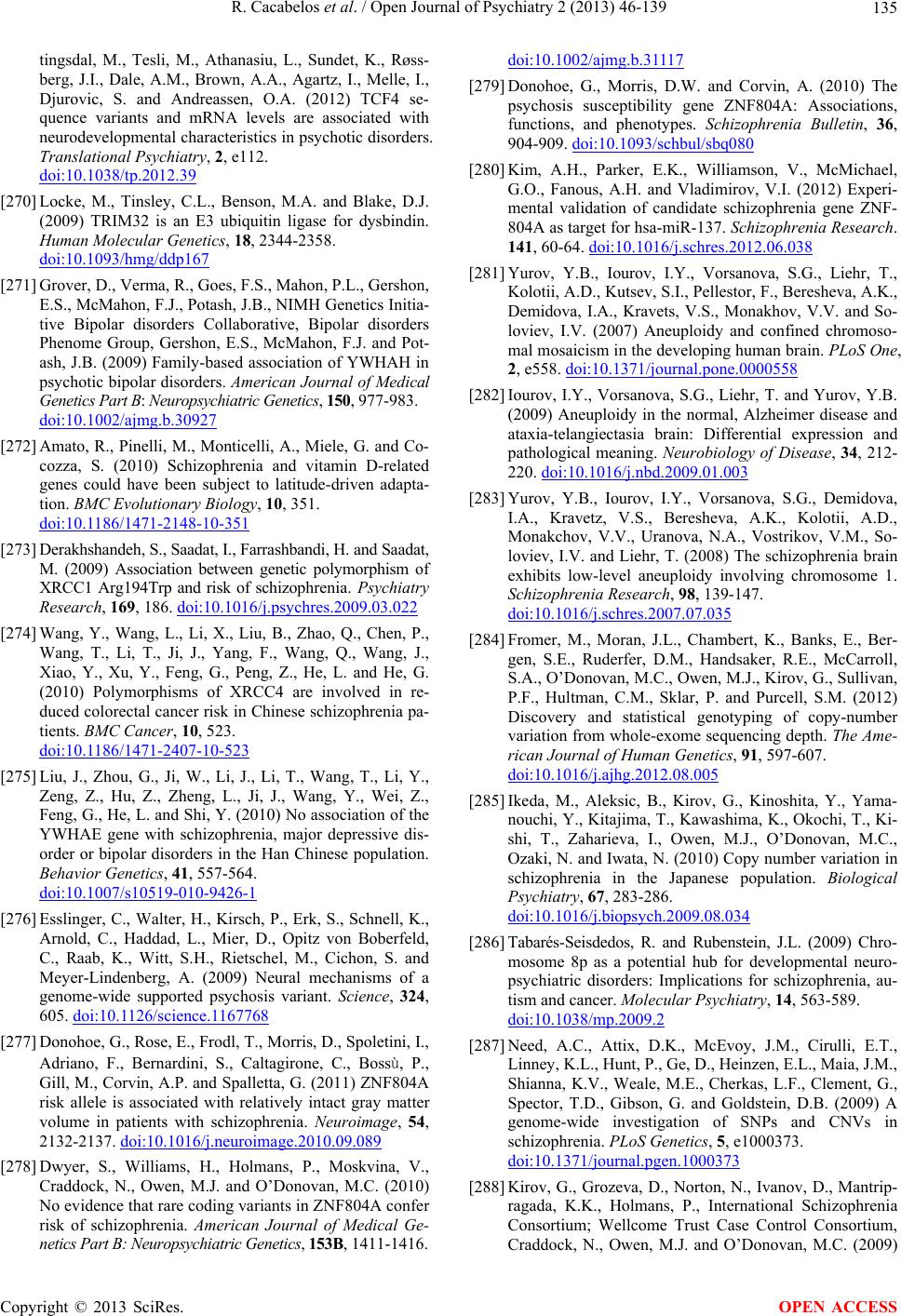 R. Cacabelos et al. / Open Journal of Psychiatry 2 (2013) 46-139 135 tingsdal, M., Tesli, M., Athanasiu, L., Sundet, K., Røss- berg, J.I., Dale, A.M., Brown, A.A., Agartz, I., Melle, I., Djurovic, S. and Andreassen, O.A. (2012) TCF4 se- quence variants and mRNA levels are associated with neurodevelopmental characteristics in psychotic disorders. Translational Psychiatry, 2, e112. doi:10.1038/tp.2012.39 [270] Locke, M., Tinsley, C.L., Benson, M.A. and Blake, D.J. (2009) TRIM32 is an E3 ubiquitin ligase for dysbindin. Human Molecular Genetics, 18, 2344-2358. doi:10.1093/hmg/ddp167 [271] Grover, D., Verma, R., Goes, F.S., Mahon, P.L., Gershon, E.S., McMahon, F.J., Potash, J.B., NIMH Genetics Initia- tive Bipolar disorders Collaborative, Bipolar disorders Phenome Group, Gershon, E.S., McMahon, F.J. and Pot- ash, J.B. (2009) Family-based association of YWHAH in psychotic bipolar disorders. American Journal of Medical Genetics Part B: Neuropsychiatric Genetics, 150, 977-983. doi:10.1002/ajmg.b.30927 [272] Amato, R., Pinelli, M., Monticelli, A., Miele, G. and Co- cozza, S. (2010) Schizophrenia and vitamin D-related genes could have been subject to latitude-driven adapta- tion. BMC Evolutionary Biology, 10, 351. doi:10.1186/1471-2148-10-351 [273] Derakhshandeh, S., Saadat, I., Farrashbandi, H. and Saadat, M. (2009) Association between genetic polymorphism of XRCC1 Arg194Trp and risk of schizophrenia. Psychiatry Research, 169, 186. doi:10.1016/j.psychres.2009.03.022 [274] Wang, Y., Wang, L., Li, X., Liu, B., Zhao, Q., Chen, P., Wang, T., Li, T., Ji, J., Yang, F., Wang, Q., Wang, J., Xiao, Y., Xu, Y., Feng, G., Peng, Z., He, L. and He, G. (2010) Polymorphisms of XRCC4 are involved in re- duced colorectal cancer risk in Chinese schizophrenia pa- tients. BMC Cancer, 10, 523. doi:10.1186/1471-2407-10-523 [275] Liu, J., Zhou, G., Ji, W., Li, J., Li, T., Wang, T., Li, Y., Zeng, Z., Hu, Z., Zheng, L., Ji, J., Wang, Y., Wei, Z., Feng, G., He, L. and Shi, Y. (2010) No association of the YWHAE gene with schizophrenia, major depressive dis- order or bipolar disorders in the Han Chinese population. Behavior Genetics, 41, 557-564. doi:10.1007/s10519-010-9426-1 [276] Esslinger, C., Walter, H., Kirsch, P., Erk, S., Schnell, K., Arnold, C., Haddad, L., Mier, D., Opitz von Boberfeld, C., Raab, K., Witt, S.H., Rietschel, M., Cichon, S. and Meyer-Lindenberg, A. (2009) Neural mechanisms of a genome-wide supported psychosis variant. Science, 324, 605. doi:10.1126/science.1167768 [277] Donohoe, G., Rose, E., Frodl, T., Morris, D., Spoletini, I., Adriano, F., Bernardini, S., Caltagirone, C., Bossù, P., Gill, M., Corvin, A.P. and Spalletta, G. (2011) ZNF804A risk allele is associated with relatively intact gray matter volume in patients with schizophrenia. Neuroimage, 54, 2132-2137. doi:10.1016/j.neuroimage.2010.09.089 [278] Dwyer, S., Williams, H., Holmans, P., Moskvina, V., Craddock, N., Owen, M.J. and O’Donovan, M.C. (2010) No evidence that rare coding variants in ZNF804A confer risk of schizophrenia. American Journal of Medical Ge- netics Part B: Neuropsychiatric Genetics, 153B, 1411-1416. doi:10.1002/ajmg.b.31117 [279] Donohoe, G., Morris, D.W. and Corvin, A. (2010) The psychosis susceptibility gene ZNF804A: Associations, functions, and phenotypes. Schizophrenia Bulletin, 36, 904-909. doi:10.1093/schbul/sbq080 [280] Kim, A.H., Parker, E.K., Williamson, V., McMichael, G.O., Fanous, A.H. and Vladimirov, V.I. (2012) Experi- mental validation of candidate schizophrenia gene ZNF- 804A as target for hsa-miR-137. Schizophrenia Research. 141, 60-64. doi:10.1016/j.schres.2012.06.038 [281] Yurov, Y.B., Iourov, I.Y., Vorsanova, S.G., Liehr, T., Kolotii, A.D., Kutsev, S.I., Pellestor, F., Beresheva, A.K., Demidova, I.A., Kravets, V.S., Monakhov, V.V. and So- loviev, I.V. (2007) Aneuploidy and confined chromoso- mal mosaicism in the developing human brain. PLoS One, 2, e558. doi:10.1371/journal.pone.0000558 [282] Iourov, I.Y., Vorsanova, S.G., Liehr, T. and Yurov, Y.B. (2009) Aneuploidy in the normal, Alzheimer disease and ataxia-telangiectasia brain: Differential expression and pathological meaning. Neurobiology of Disease, 34, 212- 220. doi:10.1016/j.nbd.2009.01.003 [283] Yurov, Y.B., Iourov, I.Y., Vorsanova, S.G., Demidova, I.A., Kravetz, V.S., Beresheva, A.K., Kolotii, A.D., Monakchov, V.V., Uranova, N.A., Vostrikov, V.M., So- loviev, I.V. and Liehr, T. (2008) The schizophrenia brain exhibits low-level aneuploidy involving chromosome 1. Schizophrenia Research, 98, 139-147. doi:10.1016/j.schres.2007.07.035 [284] Fromer, M., Moran, J.L., Chambert, K., Banks, E., Ber- gen, S.E., Ruderfer, D.M., Handsaker, R.E., McCarroll, S.A., O’Donovan, M.C., Owen, M.J., Kirov, G., Sullivan, P.F., Hultman, C.M., Sklar, P. and Purcell, S.M. (2012) Discovery and statistical genotyping of copy-number variation from whole-exome sequencing depth. The Ame- rican Journal of Human Genetics, 91, 597-607. doi:10.1016/j.ajhg.2012.08.005 [285] Ikeda, M., Aleksic, B., Kirov, G., Kinoshita, Y., Yama- nouchi, Y., Kitajima, T., Kawashima, K., Okochi, T., Ki- shi, T., Zaharieva, I., Owen, M.J., O’Donovan, M.C., Ozaki, N. and Iwata, N. (2010) Copy number variation in schizophrenia in the Japanese population. Biological Psychiatry, 67, 283-286. doi:10.1016/j.biopsych.2009.08.034 [286] Tabarés-Seisdedos, R. and Rubenstein, J.L. (2009) Chro- mosome 8p as a potential hub for developmental neuro- psychiatric disorders: Implications for schizophrenia, au- tism and cancer. Molecular Psychiatry, 14, 563-589. doi:10.1038/mp.2009.2 [287] Need, A.C., Attix, D.K., McEvoy, J.M., Cirulli, E.T., Linney, K.L., Hunt, P., Ge, D., Heinzen, E.L., Maia, J.M., Shianna, K.V., Weale, M.E., Cherkas, L.F., Clement, G., Spector, T.D., Gibson, G. and Goldstein, D.B. (2009) A genome-wide investigation of SNPs and CNVs in schizophrenia. PLoS Genetics, 5, e1000373. doi:10.1371/journal.pgen.1000373 [288] Kirov, G., Grozeva, D., Norton, N., Ivanov, D., Mantrip- ragada, K.K., Holmans, P., International Schizophrenia Consortium; Wellcome Trust Case Control Consortium, Craddock, N., Owen, M.J. and O’Donovan, M.C. (2009) Copyright © 2013 SciRes. OPEN ACCESS
 R. Cacabelos et al. / Open Journal of Psychiatry 2 (2013) 46-139 136 Support for the involvement of large copy number vari- ants in the pathogenesis of schizophrenia. Human Mo- lecular Genetics, 18, 1497-1503. doi:10.1093/hmg/ddp043 [289] Maziade, M., Chagnon, Y.C., Roy, M.A., Bureau, A., Fournier, A. and Mérette, C. (2009) Chromosome 13q13-q14 locus overlaps mood and psychotic disorders: The rele- vance for redefining phenotype. European Journal of Human Genetics, 17, 1034-1042. doi:10.1038/ejhg.2008.268 [290] de Kovel, C.G., Trucks, H., Helbig, I., Mefford, H.C., Baker, C., Leu, C., Kluck, C., Muhle, H., von Spiczak, S., Ostertag, P., Obermeier, T., Kleefuss-Lie, A.A., Hall- mann, K., Steffens, M., Gaus, V., Klein, K.M., Hamer, H.M., Rosenow, F., Brilstra, E.H., Trenité, D.K.-N., Swinkels, M.E., Weber, Y.G., Unterberger, I., Zimprich, F., Urak, L., Feucht, M., Fuchs, K., Møller, R.S., Hjal- grim, H., De Jonghe, P., Suls, A., Rückert, I.M., Wich- mann, H.E., Franke, A., Schreiber, S., Nürnberg, P., Elger, C.E., Lerche, H., Stephani, U., Koeleman, B.P., Lindhout, D., Eichler, E.E. and Sander, T. (2010) Recurrent mi- crodeletions at 15q11.2 and 16p13.11 predispose to idio- pathic generalized epilepsies. Brain, 133, 23-32. doi:10.1093/brain/awp262 [291] Dibbens, L.M., Mullen, S., Helbig, I., Mefford, H.C., Bayly, M.A., Bellows, S., Leu, C., Trucks, H., Obermeier, T., Wittig, M., Franke, A., Caglayan, H., Yapici, Z., EPICURE Consortium, Sander, T., Eichler, E.E., Schef- fer, I.E., Mulley, J.C. and Berkovic, S.F. (2009) Familial and sporadic 15q13.3 microdeletions in idiopathic gener- alized epilepsy: Precedent for disorders with complex in- heritance. Human Molecular Genetics, 18, 3626-3631. doi:10.1093/hmg/ddp311 [292] Ben-Shachar, S., Lanpher, B., German, J.R., Qasaymeh, M., Potocki, L., Nagamani, S.C., Franco, L.M., Malphrus, A., Bottenfield, G.W., Spence, J.E., Amato, S., Rousseau, J.A., Moghaddam, B., Skinner, C., Skinner, S.A., Bernes, S., Armstrong, N., Shinawi, M., Stankiewicz, P., Patel, A., Cheung, S.W., Lupski, J.R., Beaudet, A.L. and Sahoo, T. (2009) Microdeletion 15q13.3: A locus with incomplete penetrance for autism, mental retardation, and psychiatric disorders. Journal of Medical Genetics, 46, 382-388. doi:10.1136/jmg.2008.064378 [293] Leonard, S. and Freedman, R. (2006) Genetics of chro- mosome 15q13-q14 in schizophrenia. Biological Psy- chiatry, 60, 115-122. doi:10.1016/j.biopsych.2006.03.054 [294] Pagnamenta, A.T., Wing, K., Sadighi Akha, E., Knight, S.J., Bölte, S., Schmötzer, G., Duketis, E., Poustka, F., Klauck, S.M., Poustka, A., Ragoussis, J., Bailey, A.J., Monaco, A.P. and International Molecular Genetic Study of Autism Consortium. (2009) A 15q13.3 microdeletion segregating with autism. European Journal of Human Genetics, 17, 687-692. doi:10.1038/ejhg.2008.228 [295] Ingason, A., Rujescu, D., Cichon, S., Sigurdsson, E., Sigmundsson, T., Pietiläinen, O.P., Buizer-Voskamp, J.E., Strengman, E., Francks, C., Muglia, P., Gylfason, A., Gustafsson, O., Olason, P.I., Steinberg, S., Hansen, T., Jakobsen, K.D., Rasmussen, H.B., Giegling, I., Möller, H.J., Hartmann, A., Crombie, C., Fraser, G., Walker, N., Lonnqvist, J., Suvisaari, J., Tuulio-Henriksson, A., Bramon, E., Kiemeney, L.A., Franke, B., Murray, R., Vassos, E., Toulopoulou, T., Mühleisen, T.W., Tosato, S., Ruggeri, M., Djurovic, S., Andreassen, O.A., Zhang, Z., Werge, T., Ophoff, R.A., Group Investigators, Rietschel, M., Nöthen, M.M., Petursson, H., Stefansson, H., Pelto- nen, L., Collier, D., Stefansson, K. and St Clair, D.M. (2011) Copy number variations of chromosome 16p13.1 region associated with schizophrenia. Molecular Psy- chiatry, 16, 17-25. doi:10.1038/mp.2009.101 [296] Jolin, E.M., Weller, R.A. and Weller, E.B. (2009) Psy- chosis in children with velocardiofacial syndrome (22q11.2 deletion syndrome). Current Psychiatry Reports, 11, 99-105. doi:10.1007/s11920-009-0016-y [297] Shashi, V., Kwapil, T.R., Kaczorowski, J., Berry, M.N., Santos, C.S., Howard, T.D., Goradia, D., Prasad, K., Vaibhav, D., Rajarethinam, R., Spence, E. and Keshavan, M.S. (2010) Evidence of gray matter reduction and dys- function in chromosome 22q11.2 deletion syndrome. Psychiatry Research, 181, 1-8. doi:10.1016/j.pscychresns.2009.07.003 [298] Guilmatre, A., Dubourg, C., Mosca, A.L., Legallic, S., Goldenberg, A., Drouin-Garraud, V., Layet, V., Rosier, A., Briault, S., Bonnet-Brilhault, F., Laumonnier, F., Odent, S., Le Vacon, G., Joly-Helas, G., David, V., Bendavid, C., Pi- noit, J.M., Henry, C., Impallomeni, C., Germano, E., Tor- torella, G., Di Rosa, G., Barthelemy, C., Andres, C., Faivre, L., Frébourg, T., Saugier Veber, P. and Campion, D. (2009) Recurrent rearrangements in synaptic and neurodevelop- mental genes and shared biologic pathways in schizophre- nia, autism, and mental retardation. Archives of General Psychiatry, 66, 947-956. doi:10.1001/archgenpsychiatry.2009.80 [299] Meechan, D.W., Tucker, E.S., Maynard, T.M. and LaMan- tia, A.S. (2009) Diminished dosage of 22q11 genes dis- rupts neurogenesis and cortical development in a mouse model of 22q11 deletion/DiGeorge syndrome. Proceedings of the National Academy of Sciences, 106, 16434-16445. doi:10.1073/pnas.0905696106 [300] Prasad, S.E., Howley, S. and Murphy, K.C. (2008) Can- didate genes and the behavioral phenotype in 22q11.2 deletion syndrome. Developmental Disabilities Research Reviews, 14, 26-34. doi:10.1002/ddrr.5 [301] Vincent, J.B., Paterson, A.D., Strong, E., Petronis, A. and Kennedy, J.L. (2000) The unstable trinucleotide repeat story of major psychosis. American Journal of Medical Genetics, 97, 77-97. doi:10.1002/(SICI)1096-8628(200021)97:1<77::AID-AJ MG11>3.0.CO;2-3 [302] Bruce, H.A., Sachs, N., Rudnicki, D.D., Lin, S.G., Willour, V.L., Cowell, J.K., Conroy, J., McQuaid, D.E., Rossi, M., Gaile, D.P., Nowak, N.J., Holmes, S.E., Sklar, P., Ross, C.A., Delisi, L.E. and Margolis, R.L. (2009) Long tandem repeats as a form of genomic copy number variation: Structure and length polymorphism of a chromosome 5p repeat in control and schizophrenia populations. Psychiat- ric Genetics, 19, 64-71. doi:10.1097/YPG.0b013e3283207ff6 [303] Hamshere, M.L., Schulze, T.G., Schumacher, J., Corvin, A., Owen, M.J., Jamra, R.A., Propping, P., Maier, W., Copyright © 2013 SciRes. OPEN ACCESS
 R. Cacabelos et al. / Open Journal of Psychiatry 2 (2013) 46-139 137 Orozco y Diaz, G., Mayoral, F., Rivas, F., Jones, I., Jones, L., Kirov, G., Gill, M., Holmans, P.A., Nöthen, M.M., Cichon, S., Rietschel, M. and Craddock, N. (2009) Mood- incongruent psychosis in bipolar disorders: Conditional linkage analysis shows genome-wide suggestive linkage at 1q32.3, 7p13 and 20q13.31. Bipolar disorders, 11, 610-620. doi:10 .1111 /j.1399-5618.2009.00736.x [304] Shaffer, L.G., Theisen, A., Bejjani, B.A., Ballif, B.C., Aylsworth, A.S., Lim, C., McDonald, M., Ellison, J.W., Kostiner, D., Saitta, S. and Shaikh, T. (2007) The discov- ery of microdeletion syndromes in the post-genomic era: Review of the methodology and characterization of a new 1q41q42 microdeletion syndrome. Genetics in Medicine, 9, 607-616. doi:10.1097/GIM.0b013e3181484b49 [305] Slavotinek, A.M. (2008) Novel microdeletion syndromes detected by chromosome microarrays. Human Genetics, 124, 1-17. doi:10.1007/s00439-008-0513-9 [306] Glessner, J.T., Wang, K., Cai, G., Korvatska, O., Kim, C.E., Wood, S., Zhang, H., Estes, A., Brune, C.W., Bradfield, J.P., Imielinski, M., Frackelton, E.C., Reichert, J., Crawford, E.L., Munson, J., Sleiman, P.M., Chiavacci, R., Annaiah, K., Thomas, K., Hou, C., Glaberson, W., Flory, J., Otieno, F., Garris, M., Soorya, L., Klei, L., Piven, J., Meyer, K.J., Anagnostou, E., Sakurai, T., Game, R.M., Rudd, D.S., Zurawiecki, D., McDougle, C.J., Davis, L.K., Miller, J., Posey, D.J., Michaels, S., Kolevzon, A., Silverman, J.M., Bernier, R., Levy, S.E., Schultz, R.T., Dawson, G., Owley, T., McMahon, W.M., Wassink, T.H., Sweeney, J.A., Nurn- berger, J.I., Coon, H., Sutcliffe, J.S., Minshew, N.J., Grant, S.F., Bucan, M., Cook, E.H., Buxbaum, J.D., Devlin, B., Schellenberg, G.D. and Hakonarson, H. (2009) Autism ge- nome-wide copy number variation reveals ubiquitin and neuronal genes. Nature, 459, 569-573. doi:10.1038/nature07953 [307] Bucan, M., Abrahams, B.S., Wang, K., Glessner, J.T., Herman, E.I., Sonnenblick, L.I., Alvarez Retuerto, A.I., Imielinski, M., Hadley, D., Bradfield, J.P., Kim, C., Gidaya, N.B., Lindquist, I., Hutman, T., Sigman, M., Kustanovich, V., Lajonchere, C.M., Singleton, A., Kim, J., Wassink, T.H., McMahon, W.M., Owley, T., Sweeney, J.A., Coon, H., Nurnberger, J.I., Li, M., Cantor, R.M., Minshew, N.J., Sutcliffe, J.S., Cook, E.H., Dawson, G., Buxbaum, J.D., Grant, S.F., Schellenberg, G.D., Geschwind, D.H. and Ha- konarson, H. (2009) Genome-wide analyses of exonic copy number variants in a family-based study point to novel au- tism susceptibility genes. PLoS Genetics, 5, e1000536. doi:10.1371/journal.pgen.1000536 [308] Kumar, R.A. and Christian, S.L. (2009) Genetics of au- tism spectrum disorders. Current Neurology and Neuro- science Reports, 9, 188-197. doi:10.1007/s11910-009-0029-2 [309] Yan, J., Zhang, F., Brundage, E., Scheuerle, A., Lanpher, B., Erickson, R.P., Powis, Z., Robinson, H.B., Trapane, P.L., Stachiw-Hietpas, D., Keppler-Noreuil, K.M., Lalani, S.R., Sahoo, T., Chinault, A.C., Patel, A., Cheung, S.W. and Lupski, J.R. (2009) Genomic duplication resulting in increased copy number of genes encoding the sister chro- matid cohesion complex conveys clinical consequences distinct from Cornelia de Lange. Journal of Medical Ge- netics, 46, 626-634. doi:10.1136/jmg.2008.062471 [310] Kong, A., Frigge, M.L., Masson, G., Besenbacher, S., Sulem, P., Magnusson, G., Gudjonsson, S.A., Sigurdsson, A., Jonasdottir, A., Jonasdottir, A., Wong, W.S., Sigurds- son, G., Walters, G.B., Steinberg, S., Helgason, H., Thorleifsson, G., Gudbjartsson, D.F., Helgason, A., Magnusson, O.T., Thorsteinsdottir, U. and Stefansson, K. (2012) Rate of de novo mutations and the importance of father’s age to disease risk. Nature, 488, 471-475. doi:10.1038/nature11396 [311] Sun, G., Yan, J., Noltner, K., Feng, J., Li, H., Sarkis, D.A., Sommer, S.S. and Rossi, J.J. (2009) SNPs in hu- man miRNA genes affect biogenesis and function. RNA, 15, 1640-1651. doi:10.1261/rna.1560209 [312] Feng, J., Sun, G., Yan, J., Noltner, K., Li, W., Buzin, C.H., Longmate, J., Heston, L.L., Rossi, J. and Sommer, S.S. (2009) Evidence for X-chromosomal schizophrenia associated with microRNA alterations. PLoS One, 4, e6121. doi:10.1371/journal.pone.00061 21 [313] Burmistrova, O.A., Goltsov, A.Y., Abramova, L.I., Kaleda, V.G., Orlova, V.A. and Rogaev, E.I. (2007) Mi- croRNA in schizophrenia: Genetic and expression analy- sis of miR-130b (22q11). Biochemistry (Moscow), 72, 578-582. doi:10.1134/S0006297907050161 [314] Gregory, R.I., Chendrimada, T.P. and Shiekhattar, R. (2006) MicroRNA biogenesis: Isolation and characteriza- tion of the microprocessor complex. Methods in Molecu- lar Biology, 342, 33-47. http://www.ncbi.nlm.nih.gov/pubmed/16957365 [315] Zhu, Y., Kalbfleisch, T., Brennan, M.D. and Li, Y. (2009) MicroRNA gene is hosted in an intron of a schizophre- nia-susceptibility gene. Schizophrenia Research, 109, 86-89. doi:10.1016/j.schres.2009.01.022 [316] Cummings, E., Donohoe, G., Hargreaves, A., Moore, S., Fahey, C., Dinan, T.G., McDonald, C., O’Callaghan, E., O’Neill, F.A., Waddington, J.L., Murphy, K.C., Morris, D.W., Gill, M. and Corvin, A. (2012) Mood congruent psychotic symptoms and specific cognitive deficits in carriers of the novel schizophrenia risk variant at MIR- 137. Neuroscience Letters (in press). doi:10.1016/j.neulet.2012.08.065 [317] Pinsonneault, J.K., Papp, A.C. and Sadée, W. (2006) Alle- lic mRNA expression of X-linked monoamine oxidase a (MAOA) in human brain: Dissection of epigenetic and ge- netic factors. Human Molecular Genetics, 15, 2636-2649. doi:10.1093/hmg/ddl192 [318] Crews, D. (2008) Epigenetics and its implications for behavioural neuroendocrinology. Neuroendocrinology, 29, 344-357. doi:10.1016/j.yfrne.2008.01.003 [319] Feng, J. and Fan, G. (2009) The role of DNA methylation in the central nervous system and neuropsychiatric disor- ders. International Review of Neurobiology, 89, 67-84. doi:10.1016/S0074-7742(09)89004-1 [320] Reichenberg, A., Mill, J. and MacCabe, J.H. (2009) Epi- genetics, genomic mutations and cognitive function. Cog- nitive Neuropsychiatry, 14, 377-390. doi:10.1080/13546800902978417 [321] MacDonald, J.L. and Roskams, A.J. (2009) Epigenetic regulation of nervous system development by DNA me- thylation and histone deacetylation. Progress in Neurobi- ology, 88, 170-183. doi:10.1016/j.pneurobio.2009.04.002 Copyright © 2013 SciRes. OPEN ACCESS
 R. Cacabelos et al. / Open Journal of Psychiatry 2 (2013) 46-139 138 [322] Urdinguio, R.G., Sanchez-Mut, J.V. and Esteller, M. (2009) Epigenetic mechanisms in neurological diseases: Genes, syndromes, and therapies. The Lancet Neurology, 8, 1056-1072. doi:10.1016/S1474-4422(09)70262-5 [323] Bronner, C., Chataigneau, T., Schini-Kerth, V.B. and Landry, Y. (2007) The “Epigenetic Code Replication Machinery”, ECREM: A promising drugable target of the epigenetic cell memory. Current Medicinal Chemistry, 14, 2629-2641. doi:10.2174/092986707782023244 [324] Chavez-Blanco, A., Perez-Plasencia, C., Perez-Cardenas, E., Carrasco-Legleu, C., Rangel-Lopez, E., Segura-Pacheco, B., Taja-Chayeb, L., Trejo-Becerril, C., Gonzalez-Fierro, A., Candelaria, M., Cabrera, G. and Duenas-Gonzalez, A. (2006) Antineoplastic effects of the DNA methylation in- hibitor hydralazine and the histone deacetylase inhibitor valproic acid in cancer cell lines. Cancer Cell Interna- tional, 6, 2. doi:10.1186/1475-2867-6-2 [325] Abdolmaleky, H.M., Smith, C.L., Zhou, J.R. and Thiagalingam, S. (2008) Epigenetic alterations of the dopaminergic system in major psychiatric disorders. Methods in Molecular Biology, 448, 187-212. doi:10.1007/978-1-59745-205-2_9 [326] Kazantsev, A.G. and Thompson, L.M. (2008) Therapeu- tic application of histone deacetylase inhibitors for central nervous system disorders. Nature Reviews Drug Discovery, 7, 854-868. doi:10.1038/nrd2681 [327] Dong, E., Nelson, M., Grayson, D.R., Costa, E. and Gui- dotti, A. (2008) Clozapine and sulpiride but not haloperi- dol or olanzapine activate brain DNA demethylation. Pro- ceedings of the National Academy of Sciences, 105, 13614-13619. doi:10.1073/pnas.0805493105 [328] Guidotti, A., Dong, E., Kundakovic, M., Satta, R., Gray- son, D.R. and Costa, E. (2009) Characterization of the ac- tion of antipsychotic subtypes on valproate-induced chro- matin remodeling. Trends in Pharmacological Sciences, 30, 55-60. doi:10.1016/j.tips.2008.10.010 [329] Costa, E., Chen, Y., Dong, E., Grayson, D.R., Kundakovic, M., Maloku, E., Ruzicka, W., Satta, R., Veldic, M., Zhubi, A. and Guidotti, A. (2009) GABAergic promoter hyper- methylation as a model to study the neurochemistry of schizophrenia vulnerability. Expert Review of Neurothe- rapeutics, 9, 87-98. doi:10.1586/14737175.9.1.87 [330] Dong, E., Agis-Balboa, R.C., Simonini, M.V., Grayson, D.R., Costa, E. and Guidotti, A. (2005) Reelin and glu- tamic acid decarboxylase67 promoter remodeling in an epigenetic methionine-induced mouse model of schizo- phrenia. Proceedings of the National Academy of Sci- ences of USA, 102, 12578-12583. doi:10.1073/pnas.0505394102 [331] Grayson, D.R., Chen, Y., Dong, E., Kundakovic, M. and Guidotti, A. (2009) From trans-methylation to cytosine methylation: Evolution of the methylation hypothesis of schizophrenia. Epigenetics, 4, 144-149. doi:10.4161/epi.4.3.8534 [332] Gavin, D.P., Kartan, S., Chase, K., Jayaraman, S. and Sharma, R.P. (2009) Histone deacetylase inhibitors and candidate gene expression: An in vivo and in vitro ap- proach to studying chromatin remodeling in a clinical population. Journal of Psychiatric Research, 43, 870-876. doi:10.1016/j.jpsychires.2008.12.006 [333] Li, J., Harris, R.A., Cheung, S.W., Coarfa, C., Jeong, M., Goodell, M.A., White, L.D., Patel, A., Kang, S.H., Shaw, C., Chinault, A.C., Gambin, T., Gambin, A., Lupski, J.R. and Milosavljevic, A. (2012) Genomic hypomethylation in the human germline associates with selective structural mutability in the human genome. PLoS Genetics. 8, e1002692. doi:10.1371/journal.pgen.1002692 [334] Rezin, G.T., Amboni, G., Zugno, A.I., Quevedo, J. and Streck, E.L. (2009) Mitochondrial dysfunction and psychi- atric disorders. Neurochemical Research, 34, 1021-1029. doi:10.1007/s11064-008-9865-8 [335] Shao, L., Martin, M.V., Watson, S.J., Schatzberg, A., Akil, H., Myers, R.M., Jones, E.G., Bunney, W.E. and Vawter, M.P. (2008) Mitochondrial involvement in psy- chiatric disorders. Annals of Medicine, 40, 281-295. doi:10.1080/07853890801923753 [336] Rollins, B., Martin, M.V., Sequeira, P.A., Moon, E.A., Morgan, L.Z., Watson, S.J., Schatzberg, A., Akil, H., Myers, R.M., Jones, E.G., Wallace, D.C., Bunney WE and Vawter MP, (2009) Mitochondrial variants in schi- zophrenia, bipolar disorders, and major depressive disor- der. PLoS One, 4, e4913. doi:10.1371/journal.pone.0004913 [337] Munakata, K., Iwamoto, K., Bundo, M. and Kato, T. (2005) Mitochondrial DNA 3243A > G mutation and in- creased expression of LARS2 gene in the brains of pa- tients with bipolar disorders and schizophrenia. Biologi- cal Psychiatry, 57, 525-532. doi:10.1016/j.biopsych.2004.11.041 [338] Washizuka S, Iwamoto K, Kakiuchi C, Bundo M and Kato T. (2009) Expression of mitochondrial complex I subunit gene NDUFV2 in the lymphoblastoid cells de- rived from patients with bipolar disorders and schizo- phrenia. Neuroscience Reseasrch, 63, 199-204. doi:10.1016/j.neures.2008.12.004 [339] Park, Y.U., Jeong, J., Lee, H., Mun, J.Y., Kim, J.H., Lee, J.S., Nguyen, M.D., Han, S.S., Suh, P.G. and Park, S.K. (2010) Disrupted-in-schizophrenia 1 (DISC1) plays es- sential roles in mitochondria in collaboration with Mito- filin. Proceedings of the National Academy of Sciences, 107, 17785-17790. doi:10.1073/pnas.1004361107 [340] Bilder, R.M., Sabb, F.W., Parker, D.S., Kalar, D., Chu, W.W., Fox, J., Freimer, N.B. and Poldrack, R.A. (2009) Cognitive ontologies for neuropsychiatric phenomics re- search. Cognitive Neuropsychiatry, 14, 419-450. doi:10.1080/13546800902787180 [341] Meda, S.A., Jagannathan, K., Gelernter, J., Calhoun, V.D., Liu, J., Stevens, M.C. and Pearlson, G.D. (2010) A pilot multivariate parallel ICA study to investigate differential linkage between neural networks and genetic profiles in schizophrenia. Neuroimage, 53, 1007-1015. doi:10.1016/j.neuroimage.2009.11.052 [342] Bergen, S.E., Fanous, A.H., Walsh, D., O’Neill, F.A. and Kendler, K.S. (2009) Polymorphisms in SLC6A4, PAH, GABRB3, and MAOB and modification of psychotic disorder features. Schizophrenia Research, 109, 94-97. doi:10.1016/j.schres.2009.02.009 [343] Chu TT, Liu Y and Kemether E. (2009) Thalamic tran- Copyright © 2013 SciRes. OPEN ACCESS
 R. Cacabelos et al. / Open Journal of Psychiatry 2 (2013) 46-139 Copyright © 2013 SciRes. 139 OPEN ACCESS scriptome screening in three psychiatric states. Journal of Human Genetics, 54, 665-675. doi:10.1038/jhg.2009.93 [344] Dean, B., Boer, S., Gibbons, A., Money, T. and Scarr. E. (2009) Recent advances in post-mortem pathology and neurochemistry in schizophrenia. Current Opinion in Psychiatry, 22, 154-160. doi:10.1097/YCO.0b013e328323d52e [345] Martins-de-Souza, D., Gattaz, W.F., Schmitt, A., Novello, J.C., Marangoni, S., Turck, C.W. and Dias-Neto, E. (2009) Proteome analysis of schizophrenia patients Wernicke’s area reveals an energy metabolism dysregulation. BMC Psychiatry, 9, 17. doi:10.1186/1471-244X-9-17 [346] Kuzman, M.R., Medved, V., Terzic, J. and Krainc, D. (2009) Genome-wide expression analysis of peripheral blood identifies candidate biomarkers for schizophrenia. Journal of Psychiatric Research, 43, 1073-1077. doi:10.1016/j.jpsychires.2009.03.005 [347] Quinones, M.P. and Kaddurah-Daouk, R. (2009) Me- tabolomics tools for identifying biomarkers for neuro- psychiatric diseases. Neurobiology of Disease, 35, 165-76. doi:10.1016/j.nbd.2009.02.019 [348] Need, A.C., Motulsky, A.G. and Goldstein, D.B. (2005) Priorities and standards in pharmacogenetic research. Nature Genetics, 37, 671-681. doi:10.1038/ng1593 [349] Johnson, A.D., Wang, S. and Sadee, W. (2005) Poly- morphisms affecting gene regulation and mRNA proc- essing: Broad implications for pharmacogenetics. Phar- macology & Therapeutics, 106, 19-38. doi:10.1016/j.pharmthera.2004.11.001 [350] Ishikawa, T., Onishi, Y., Hirano, H., Oosumi, K., Naga- kura, M. and Tarui, S. (2004) Pharmacogenomics of drug transporters: A new approach to functional analysis of the genetic polymorphisms of ABCB1 (P-glycoprotein/MDR1). Biological & Pharmaceutical Bulletin, 27, 939-948. doi:10.1248/bpb.27.939 [351] Nishimura, M. and Naito, S. (2008) Tissue-specific mRNA expression profiles of human solute carrier trans- porter superfamilies. Drug Metabolism and Pharmacoki- netics, 23, 22-44. doi:10.2133/dmpk.23.22 [352] Agrawal, N., Dasaradhi, P.V., Mohmmed, A., Malhotra, P., Bhatnagar, R.K. and Mukherjee, S.K. (2003) RNA in- terference: biology, mechanism, and applications. Micro- biology and Molecular Biology Reviews, 67, 657-685. doi:10.1128/MMBR.67.4.657-685.2003 [353] Leung, R.K. and Whittaker, P.A. (2005) RNA interfer- ence: From gene silencing to gene-specific therapeutics. Pharmacology & Therapeutics, 107, 222-239. doi:10.1016/j.pharmthera.2005.03.004 [354] Gonzalez-Alegre, P. (2007) Therapeutic RNA interfer- ence for neurodegenerative diseases: From promise to progress. Pharmacology & Therapeutics, 114, 34-55. doi:10.1016/j.pharmthera.2007.01.003 [355] Ying, S.Y. and Lin, S.L. (2009) Intron-mediated RNA interference and microRNA biogenesis. Methods in Mo- lecular Biology, 487, 387-413. doi:10.1007/978-1-60327-547-7_19 [356] Boudreau, R.L., Spengler, R.M., and Davidson, B.L. (2011) Rational design of therapeutic siRNAs: minimiz- ing off-targeting potential to improve the safety of RNAi therapy for Huntington’s disease. Molecular Therapy, 19, 2169-2177. doi:10.1038/mt.2011.185
|