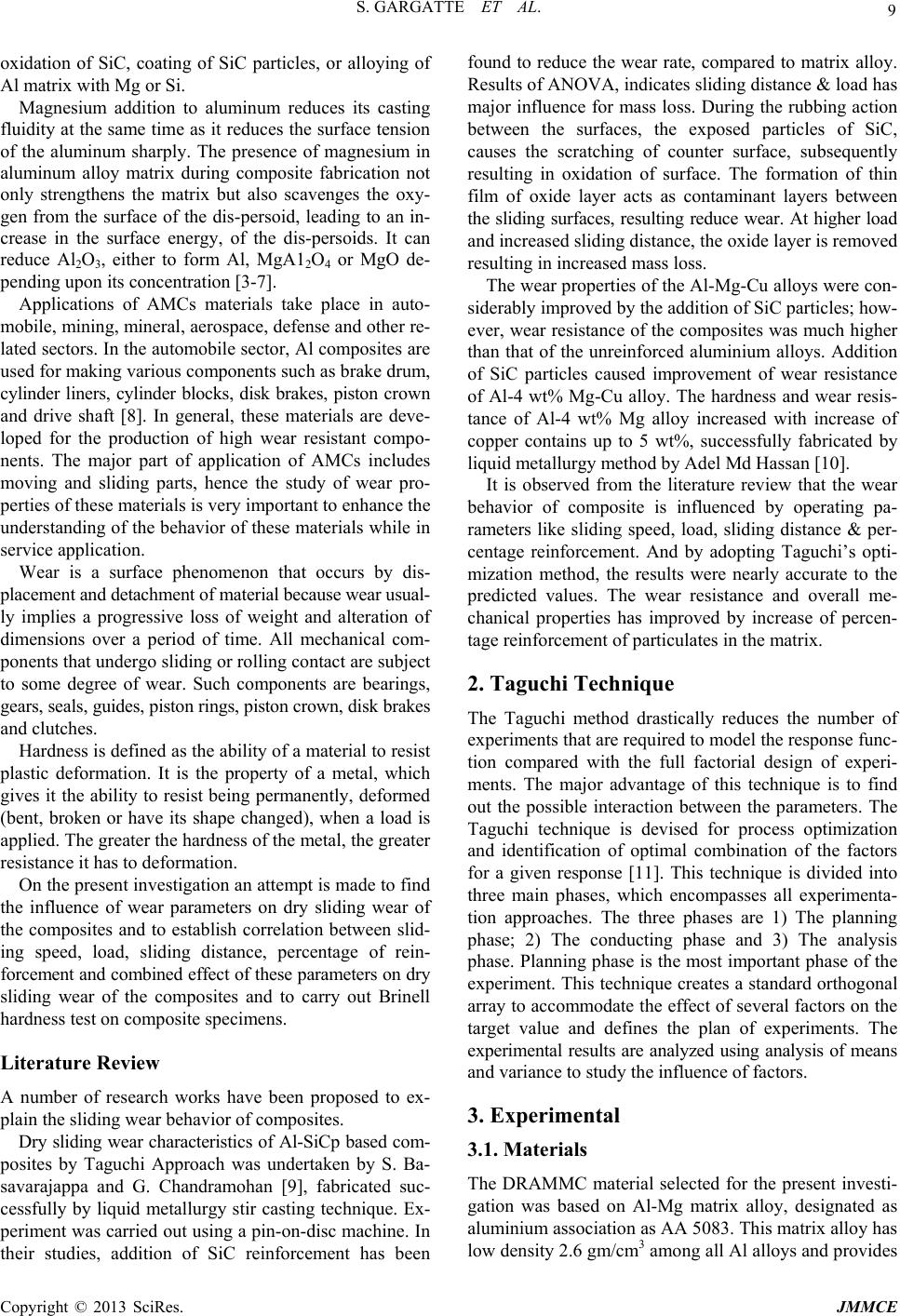
S. GARGATTE ET AL. 9
oxidation of SiC, coating of SiC particles, or alloying of
Al matrix with Mg or Si.
Magnesium addition to aluminum reduces its casting
fluidity at the same time as it reduces the surface tension
of the aluminum sharply. The presence of magnesium in
aluminum alloy matrix during composite fabrication not
only strengthens the matrix but also scavenges the oxy-
gen from the surface of the dis-persoid, leading to an in-
crease in the surface energy, of the dis-persoids. It can
reduce Al2O3, either to form Al, MgA12O4 or MgO de-
pending upon its concentration [3-7].
Applications of AMCs materials take place in auto-
mobile, mining, mineral, aerospace, defense and other re-
lated sectors. In the automobile sector, Al composites are
used for making various components such as brake drum,
cylinder liners, cylinder blocks, disk brakes, piston crown
and drive shaft [8]. In general, these materials are deve-
loped for the production of high wear resistant compo-
nents. The major part of application of AMCs includes
moving and sliding parts, hence the study of wear pro-
perties of these materials is very important to enhance the
understanding of the behavior of these materials while in
service application.
Wear is a surface phenomenon that occurs by dis-
placement and detachment of material because wear usual-
ly implies a progressive loss of weight and alteration of
dimensions over a period of time. All mechanical com-
ponents that undergo sliding or rolling contact are subject
to some degree of wear. Such components are bearings,
gears, seals, guides, piston rings, piston crown, disk brakes
and clutches.
Hardness is defined as the ability of a material to resist
plastic deformation. It is the property of a metal, which
gives it the ability to resist being permanently, deformed
(bent, broken or have its shape changed), when a load is
applied. The greater the hardness of the metal, the greater
resistance it has to deformation.
On the present investigation an attempt is made to find
the influence of wear parameters on dry sliding wear of
the composites and to establish correlation between slid-
ing speed, load, sliding distance, percentage of rein-
forcement and combined effect of these parameters on dry
sliding wear of the composites and to carry out Brinell
hardness test on composite specimens.
Literature Review
A number of research works have been proposed to ex-
plain the sliding wear behavior of composites.
Dry sliding wear characteristics of Al-SiCp based com-
posites by Taguchi Approach was undertaken by S. Ba-
savarajappa and G. Chandramohan [9], fabricated suc-
cessfully by liquid metallurgy stir casting technique. Ex-
periment was carried out using a pin-on-disc machine. In
their studies, addition of SiC reinforcement has been
found to reduce the wear rate, compared to matrix alloy.
Results of ANOVA, indicates sliding distance & load has
major influence for mass loss. During the rubbing action
between the surfaces, the exposed particles of SiC,
causes the scratching of counter surface, subsequently
resulting in oxidation of surface. The formation of thin
film of oxide layer acts as contaminant layers between
the sliding surfaces, resulting reduce wear. At higher load
and increased sliding distance, the oxide layer is removed
resulting in increased mass loss.
The wear properties of the Al-Mg-Cu alloys were con-
siderably improved by the addition of SiC particles; how-
ever, wear resistance of the composites was much higher
than that of the unreinforced aluminium alloys. Addition
of SiC particles caused improvement of wear resistance
of Al-4 wt% Mg-Cu alloy. The hardness and wear resis-
tance of Al-4 wt% Mg alloy increased with increase of
copper contains up to 5 wt%, successfully fabricated by
liquid metallurgy method by Adel Md Hassan [10].
It is observed from the literature review that the wear
behavior of composite is influenced by operating pa-
rameters like sliding speed, load, sliding distance & per-
centage reinforcement. And by adopting Taguchi’s opti-
mization method, the results were nearly accurate to the
predicted values. The wear resistance and overall me-
chanical properties has improved by increase of percen-
tage reinforcement of particulates in the matrix.
2. Taguchi Technique
The Taguchi method drastically reduces the number of
experiments that are required to model the response func-
tion compared with the full factorial design of experi-
ments. The major advantage of this technique is to find
out the possible interaction between the parameters. The
Taguchi technique is devised for process optimization
and identification of optimal combination of the factors
for a given response [11]. This technique is divided into
three main phases, which encompasses all experimenta-
tion approaches. The three phases are 1) The planning
phase; 2) The conducting phase and 3) The analysis
phase. Planning phase is the most important phase of the
experiment. This technique creates a standard orthogonal
array to accommodate the effect of several factors on the
target value and defines the plan of experiments. The
experimental results are analyzed using analysis of means
and variance to study the influence of factors.
3. Experimental
3.1. Materials
The DRAMMC material selected for the present investi-
gation was based on Al-Mg matrix alloy, designated as
aluminium association as AA 5083. This matrix alloy has
low density 2.6 gm/cm3 among all Al alloys and provides
Copyright © 2013 SciRes. JMMCE