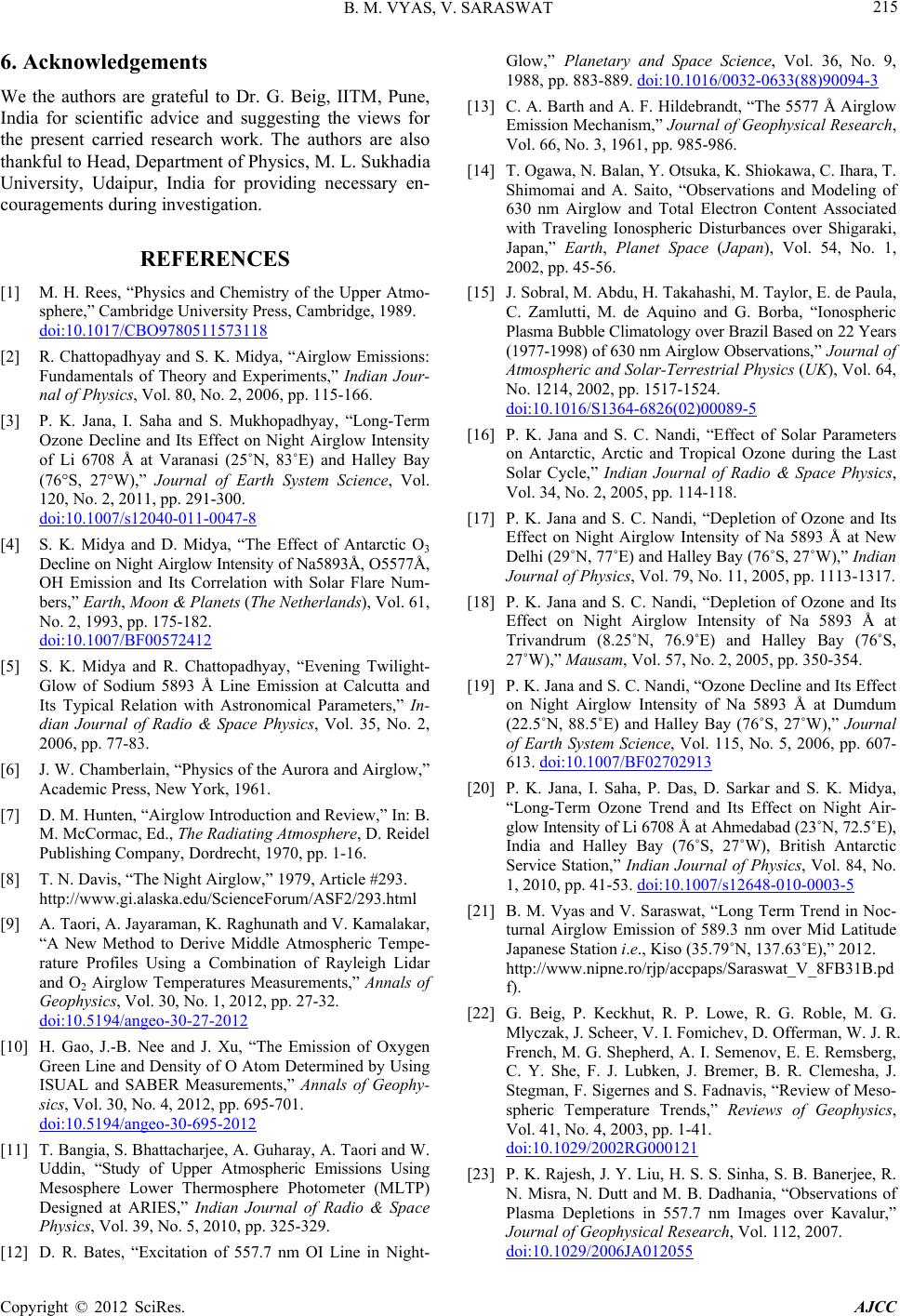
B. M. VYAS, V. SARASWAT 215
6. Acknowledgements
We the authors are grateful to Dr. G. Beig, IITM, Pune,
India for scientific advice and suggesting the views for
the present carried research work. The authors are also
thankful to Head, Department of Physics, M. L. Sukhadia
University, Udaipur, India for providing necessary en-
couragements during investigation.
REFERENCES
[1] M. H. Rees, “Physics and Chemistry of the Upper Atmo-
sphere,” Cambridge University Press, Cambridge, 1989.
doi:10.1017/CBO9780511573118
[2] R. Chattopadhyay and S. K. Midya, “Airglow Emissions:
Fundamentals of Theory and Experiments,” Indian Jour-
nal of Physics, Vol. 80, No. 2, 2006, pp. 115-166.
[3] P. K. Jana, I. Saha and S. Mukhopadhyay, “Long-Term
Ozone Decline and Its Effect on Night Airglow Intensity
of Li 6708 Å at Varanasi (25˚N, 83˚E) and Halley Bay
(76S, 27W),” Journal of Earth System Science, Vol.
120, No. 2, 2011, pp. 291-300.
doi:10.1007/s12040-011-0047-8
[4] S. K. Midya and D. Midya, “The Effect of Antarctic O3
Decline on Night Airglow Inten sity of Na5893Å, O55 77Å,
OH Emission and Its Correlation with Solar Flare Num-
bers,” Earth, Moon & Planets (The Netherlands), Vol. 61,
No. 2, 1993, pp. 175-182.
doi:10.1007/BF00572412
[5] S. K. Midya and R. Chattopadhyay, “Evening Twilight-
Glow of Sodium 5893 Å Line Emission at Calcutta and
Its Typical Relation with Astronomical Parameters,” In-
dian Journal of Radio & Space Physics, Vol. 35, No. 2,
2006, pp. 77-83.
[6] J. W. Chamberlain, “Physics of the Aurora and Airglow,”
Academic Press, New York, 1961.
[7] D. M. Hunten, “Airglow Introduction and Review,” In: B.
M. McCormac, Ed., The Radiating Atmosphere, D. Reidel
Publishing Company, Dordrecht, 1970, pp. 1-16.
[8] T. N. Davis, “The Night Airglow,” 1979, Article #293.
http://www.gi.alaska.edu/ScienceForum/ASF2/293.html
[9] A. Taori, A. Jayaraman, K. Raghunath and V. Kamalakar,
“A New Method to Derive Middle Atmospheric Tempe-
rature Profiles Using a Combination of Rayleigh Lidar
and O2 Airglow Temperatures Measurements,” Annals of
Geophysics, Vol. 30, No. 1, 2012, pp. 27-32.
doi:10.5194/angeo-30-27-2012
[10] H. Gao, J.-B. Nee and J. Xu, “The Emission of Oxygen
Green Line and Density of O Atom Determined by Using
ISUAL and SABER Measurements,” Annals of Geophy-
sics, Vol. 30, No. 4, 2012, pp. 695-701.
doi:10.5194/angeo-30-695-2012
[11] T. Bangia, S. Bhattacharjee, A. Guharay, A. Taori and W.
Uddin, “Study of Upper Atmospheric Emissions Using
Mesosphere Lower Thermosphere Photometer (MLTP)
Designed at ARIES,” Indian Journal of Radio & Space
Physics, Vol. 39, No. 5, 2010, pp. 325-329.
[12] D. R. Bates, “Excitation of 557.7 nm OI Line in Night-
Glow,” Planetary and Space Science, Vol. 36, No. 9,
1988, pp. 883-889. doi:10.1016/0032-0633(88)90094-3
[13] C. A. Barth and A. F. Hildebrandt, “The 5577 Å Airglow
Emission Mechanism,” Journal of Geophysical Research,
Vol. 66, No. 3, 1961, pp. 985-986.
[14] T. Ogawa, N. Balan, Y. Otsuka, K. Shiokawa, C. Ihara, T.
Shimomai and A. Saito, “Observations and Modeling of
630 nm Airglow and Total Electron Content Associated
with Traveling Ionospheric Disturbances over Shigaraki,
Japan,” Earth, Planet Space (Japan), Vol. 54, No. 1,
2002, pp. 45-56.
[15] J. Sobral, M. Abdu, H. Takahashi, M. Taylor, E. de Paula,
C. Zamlutti, M. de Aquino and G. Borba, “Ionospheric
Plasma Bubble Climatology over Brazil Base d on 22 Year s
(1977-1998) of 630 nm Airglow Observatio ns,” Journal of
Atmospheric and Solar-Terrestrial Physics (UK), Vol. 64,
No. 1214, 2002, pp. 1517-1524.
doi:10.1016/S1364-6826(02)00089-5
[16] P. K. Jana and S. C. Nandi, “Effect of Solar Parameters
on Antarctic, Arctic and Tropical Ozone during the Last
Solar Cycle,” Indian Journal of Radio & Space Physics,
Vol. 34, No. 2, 2005, pp. 114-118.
[17] P. K. Jana and S. C. Nandi, “Depletion of Ozone and Its
Effect on Night Airglow Intensity of Na 5893 Å at New
Delhi (29˚N, 77˚E) and Halley Bay (76˚S, 27 ˚W),” Indian
Journal of Physics, Vol. 79, No. 11, 2005, pp. 1113-1317.
[18] P. K. Jana and S. C. Nandi, “Depletion of Ozone and Its
Effect on Night Airglow Intensity of Na 5893 Å at
Trivandrum (8.25˚N, 76.9˚E) and Halley Bay (76˚S,
27˚W),” Mausam, Vol. 57, No. 2, 2005, pp. 350-354.
[19] P. K. Jana and S. C. Nandi, “Ozone Decline and Its Effect
on Night Airglow Intensity of Na 5893 Å at Dumdum
(22.5˚N, 88.5˚E) and Halley Bay (76˚S, 27˚W),” Journal
of Earth System Science, Vol. 115, No. 5, 2006, pp. 607-
613. doi:10.1007/BF02702913
[20] P. K. Jana, I. Saha, P. Das, D. Sarkar and S. K. Midya,
“Long-Term Ozone Trend and Its Effect on Night Air-
glow Intensity of Li 6708 Å at Ahmedaba d (23˚N, 72.5˚E),
India and Halley Bay (76˚S, 27˚W), British Antarctic
Service Station,” Indian Journal of Physics, Vol. 84, No.
1, 2010, pp. 41-53. doi:10.1007/s12648-010-0003-5
[21] B. M. Vyas and V. Saraswat, “Long Term Trend in Noc-
turnal Airglow Emission of 589.3 nm over Mid Latitude
Japanese Station i.e., Kiso (35.79˚N, 137.63˚E),” 2012.
http://www.nipne.ro/rjp/accpaps/Saraswat_V_8FB31B.pd
f).
[22] G. Beig, P. Keckhut, R. P. Lowe, R. G. Roble, M. G.
Mlyczak, J. Scheer, V. I. Fomichev, D. Offerman, W. J. R.
French, M. G. Shepherd, A. I. Semenov, E. E. Remsberg,
C. Y. She, F. J. Lubken, J. Bremer, B. R. Clemesha, J.
Stegman, F. Sigerne s and S. Fadnavis, “Revie w of Meso-
spheric Temperature Trends,” Reviews of Geophysics,
Vol. 41, No. 4, 2003, pp. 1-41.
doi:10.1029/2002RG000121
[23] P. K. Rajesh, J. Y. Li u, H. S. S. Sinha, S. B. Banerjee , R.
N. Misra, N. Dutt and M. B. Dadhania, “Observations of
Plasma Depletions in 557.7 nm Images over Kavalur,”
Journal of Geophysical Research, Vol. 112, 2007.
doi:10.1029/2006JA012055
Copyright © 2012 SciRes. AJCC