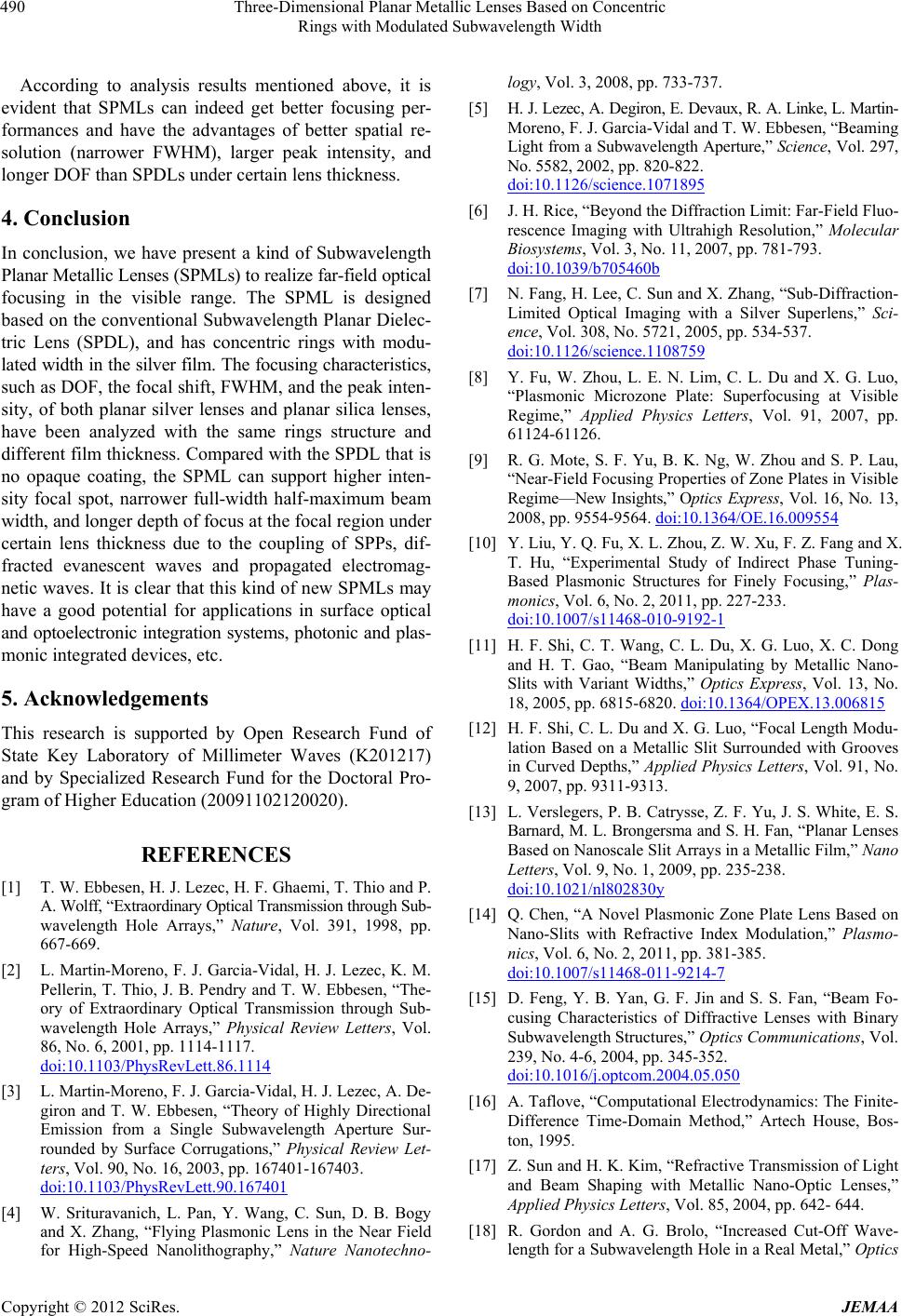
Three-Dimensional Planar Metallic Lenses Based on Concentric
Rings with Modulated Subwavelength Width
490
According to analysis results mentioned above, it is
evident that SPMLs can indeed get better focusing per-
formances and have the advantages of better spatial re-
solution (narrower FWHM), larger peak intensity, and
longer DOF than SPDLs under certain lens thickness.
4. Conclusion
In conclusion, we have present a kind of Subwavelength
Planar Metallic Lenses (SPMLs) to realize far-field optical
focusing in the visible range. The SPML is designed
based on the conventional Subwavelength Planar Dielec-
tric Lens (SPDL), and has concentric rings with modu-
lated width in the silver film. The focusing characteristics,
such as DOF, the focal shift, FWHM, and the peak inten-
sity, of both planar silver lenses and planar silica lenses,
have been analyzed with the same rings structure and
different film thickness. Compared with the SPDL that is
no opaque coating, the SPML can support higher inten-
sity focal spot, narrower full-width half-maximum beam
width, and longer depth of focus at the focal region under
certain lens thickness due to the coupling of SPPs, dif-
fracted evanescent waves and propagated electromag-
netic waves. It is clear that this kind of new SPMLs may
have a good potential for applications in surface optical
and optoelectronic integration systems, photonic and plas-
monic integrated devices, etc.
5. Acknowledgements
This research is supported by Open Research Fund of
State Key Laboratory of Millimeter Waves (K201217)
and by Specialized Research Fund for the Doctoral Pro-
gram of Higher Education (20091102120020).
REFERENCES
[1] T. W. Ebbesen, H. J. Lezec, H. F. Ghaemi, T. Thio and P.
A. Wolff, “Extraordinary Optical Transmission through Sub-
wavelength Hole Arrays,” Nature, Vol. 391, 1998, pp.
667-669.
[2] L. Martin-Moreno, F. J. Garcia-Vidal, H. J. Lezec, K. M.
Pellerin, T. Thio, J. B. Pendry and T. W. Ebbesen, “The-
ory of Extraordinary Optical Transmission through Sub-
wavelength Hole Arrays,” Physical Review Letters, Vol.
86, No. 6, 2001, pp. 1114-1117.
doi:10.1103/PhysRevLett.86.1114
[3] L. Martin-Moreno, F. J. Garcia-Vidal, H. J. Lezec, A. De-
giron and T. W. Ebbesen, “Theory of Highly Directional
Emission from a Single Subwavelength Aperture Sur-
rounded by Surface Corrugations,” Physical Review Let-
ters, Vol. 90, No. 16, 2003, pp. 167401-167403.
doi:10.1103/PhysRevLett.90.167401
[4] W. Srituravanich, L. Pan, Y. Wang, C. Sun, D. B. Bogy
and X. Zhang, “Flying Plasmonic Lens in the Near Field
for High-Speed Nanolithography,” Nature Nanotechno-
logy, Vol. 3, 2008, pp. 733-737.
[5] H. J. Lezec, A. Degiron, E. Devaux, R. A. Linke, L. Martin-
Moreno, F. J. Garcia-Vidal and T. W. Ebbesen, “Beaming
Light from a Subwavelength Aperture,” Science, Vol. 297,
No. 5582, 2002, pp. 820-822.
doi:10.1126/science.1071895
[6] J. H. Rice, “Beyond the Diffraction Limit: Far-Field Fluo-
rescence Imaging with Ultrahigh Resolution,” Molecular
Biosystems, Vol. 3, No. 11, 2007, pp. 781-793.
doi:10.1039/b705460b
[7] N. Fang, H. Lee, C. Sun and X. Zhang, “Sub-Diffraction-
Limited Optical Imaging with a Silver Superlens,” Sci-
ence, Vol. 308, No. 5721, 2005, pp. 534-537.
doi:10.1126/science.1108759
[8] Y. Fu, W. Zhou, L. E. N. Lim, C. L. Du and X. G. Luo,
“Plasmonic Microzone Plate: Superfocusing at Visible
Regime,” Applied Physics Letters, Vol. 91, 2007, pp.
61124-61126.
[9] R. G. Mote, S. F. Yu, B. K. Ng, W. Zhou and S. P. Lau,
“Near-Field Focusing Properties of Zone Plates in Visible
Regime—New Insights,” Optics Express, Vol. 16, No. 13,
2008, pp. 9554-9564. doi:10.1364/OE.16.009554
[10] Y. Liu, Y. Q. Fu, X. L. Zhou, Z. W. Xu, F. Z. Fang and X.
T. Hu, “Experimental Study of Indirect Phase Tuning-
Based Plasmonic Structures for Finely Focusing,” Plas-
monics, Vol. 6, No. 2, 2011, pp. 227-233.
doi:10.1007/s11468-010-9192-1
[11] H. F. Shi, C. T. Wang, C. L. Du, X. G. Luo, X. C. Dong
and H. T. Gao, “Beam Manipulating by Metallic Nano-
Slits with Variant Widths,” Optics Express, Vol. 13, No.
18, 2005, pp. 6815-6820. doi:10.1364/OPEX.13.006815
[12] H. F. Shi, C. L. Du and X. G. Luo, “Focal Length Modu-
lation Based on a Metallic Slit Surrounded with Grooves
in Curved Depths,” Applied Physics Letters, Vol. 91, No.
9, 2007, pp. 9311-9313.
[13] L. Verslegers, P. B. Catrysse, Z. F. Yu, J. S. White, E. S.
Barnard, M. L. Brongersma and S. H. Fan, “Planar Lenses
Based on Nanoscale Slit Arrays in a Metallic Film,” Nano
Letters, Vol. 9, No. 1, 2009, pp. 235-238.
doi:10.1021/nl802830y
[14] Q. Chen, “A Novel Plasmonic Zone Plate Lens Based on
Nano-Slits with Refractive Index Modulation,” Plasmo-
nics, Vol. 6, No. 2, 2011, pp. 381-385.
doi:10.1007/s11468-011-9214-7
[15] D. Feng, Y. B. Yan, G. F. Jin and S. S. Fan, “Beam Fo-
cusing Characteristics of Diffractive Lenses with Binary
Subwavelength Structures,” Optics Communications, Vol.
239, No. 4-6, 2004, pp. 345-352.
doi:10.1016/j.optcom.2004.05.050
[16] A. Taflove, “Computational Electrodynamics: The Finite-
Difference Time-Domain Method,” Artech House, Bos-
ton, 1995.
[17] Z. Sun and H. K. Kim, “Refractive Transmission of Light
and Beam Shaping with Metallic Nano-Optic Lenses,”
Applied Physics Letters, Vol. 85, 2004, pp. 642- 644.
[18] R. Gordon and A. G. Brolo, “Increased Cut-Off Wave-
length for a Subwavelength Hole in a Real Metal,” Optics
Copyright © 2012 SciRes. JEMAA