 Crystal Structure Theory and Applications, 2012, 1, 46-51 http://dx.doi.org/10.4236/csta.2012.13009 Published Online December 2012 (http://www.SciRP.org/journal/csta) Crystal and Molecular Structure, and Spectral Characteristics of Sodium 3,5-Bis(Hydroxyimino)-1-Methyl-2,4,6- Trioxocyclohexanide Olga Kovalchukova1, Nguyen Dinh Do1, Adam Stash2, Vitaly Bel’sky2, Paul Strashnov1, Andrew Alafinov3, Oleg Volyansky3, Svetlana Strashnova 1, Konstantin Kobrakov3 1Department of General Chemistry, Peoples’ Friendship University of Russia, Moscow, Russia 2Karpov Institute of Physical Chemistry, Moscow, Russia 3Department of Organic Chemistry and Chemistry of Dyes, Moscow State Textile University, Moscow, Russia Email: okovalchukova@mail.ru Received September 26, 2012; revised November 1, 2012; accepted November 12, 2012 ABSTRACT Sodium 3,5-bis(hydroxyimino)-1-methyl-2,4,6-trioxocyclohexanide C7H5N2NaO5 (I) has been isolated as the only product of the reaction of nitrosation of methylphloroglucinol. The structure of the titled compound has been deter- mined from single crystal X-ray diffraction data. The hydrated C7H5N2NaO5·2.5H2O crystallizes in the monoclinic space group C2/c, with a(Å) 16.408(3); b(Å) 12.446(3); c(Å) 13.716(3); (˚) 126.34(3). The planar organic anion exists in a triketo-dihydroxyimino form with the C–O and C–N distances from 1.220(2) to 1.271(2) Å and from 1.292(2) to 1.293 Å respectively. In the IR spectrum of I, the sharp absorption band occurred at 1681 cm−1 due to C=O stretching indicating the strong H-interactions. The correlations of theoretical (DFT-B3LYP/aug-cc-pVDZ) and experimental UV-vis absorption spectra in neutral and alkaline ethanolic solutions showed the existence of hydroxyimino-nitroso tautomerism while ionization of I. Keywords: Sodium 3,5-Bis(Hydroxyimino)-1-Methyl-2,4,6-Trioxocyclohexanide; Crystal Structure; IR Spectra; Electronic Absorption Spectra; Quantum Chemical Modeling 1. Introduction 2,4,6-Trihydroxytoluene (methylphloroglucinol, MPG) possesses strong and attractive synthetic potential be- cause of its specific structure. However, till recent years when the economically efficient method of synthesis of MPG on base of 2,4,6-trinitrotoluene [1,2] was devel- oped, it practically has not been realized. The reactions of electrophilic substitution of H-atoms in the benzene ring of methylphloroglucinol have been poorly studied till now. Except one paper concerning its bromination [3], a series of ancient publications on MPG acylation by HCN and nitriles [4-8], or ethanoil chloride [6] and anhydride [8] are known. And there is the only one example of MPG alkylation by formaldehyde in presence of sulfuric acid [9]. In frames of systematic investigations, some reactions of MPG electrophylic substitution (bromination, sul- fation, Hoesch reaction, azo-coupling) were recently re- ported [10-13]. As it was shown, in all cases except azo- coupling irrespectively of variations of reaction conditions mixtures of mono- and bis-substituted products were form- ed. In case of the reaction of MPG azo-coupling, non- standard conditions were found which allowed selective synthesis of mono- and bis azo-compounds based on MPG. In the present paper, we report the crystal structure, tautomerism, and some spectral characteristics of the pro- duct of the reaction of MPG nitrosation. 2. Experimental 2.1. Synthesis of the Title Compound The title compound was prepared by the reaction of methylphloroglucinol (MPG) with sodium nitrite. 5 g of MPG (0.0357 mole) were dissolved in 50 ml of water at 0˚C. The solution containing 6.41 g of NaNO2 (0.09 mole) in 25 ml of water was added with an intensive stir- ring. The solution was stored under 0˚C for 40 min, and then 0.7 ml of H2SO4 in 28 ml of water were added drop C opyright © 2012 SciRes. CSTA
 O. KOVALCHUKOVA ET AL. 47 wise. After 30 min the formed precipitate was filtered off and dried over P2O5. The product was finally purified by re-crystallization. Yield 82% M.p. 208˚C (with decom- position). The authenticity of the compound has been established by microanalyses, UV and IR spectra, and single crystal X-ray diffraction analysis. 2.2. Materials and Physical Measurements The melting point was determined on an Electrothermal Model 9200 apparatus and is uncorrected. The IR absor- ption spectrum was obtained in the region of 400 - 4000 cm–1 with a resolution of 4 cm–1 as KBr pellet using a Va- rian Excalibur HE 3100 IR spectrometer. Microanalysis was performed with a Carlo Erba Elemental Analyzer, model 1108. UV-vis spectrum was recorded in the range of 200 nm to 800 nm using a Varian Cary 50 Scan spec- trophotometer. Calculations of ionization constants were performed according to the procedure described in [14]. 2.3. The X-Ray Crystallography For the crystal structure determination, the single-crystal of the compound I was used for data collection on an Enraf-Nonius CAD-4 diffractometer. The β-filtered Mo Kα radiation (λ = 0.71073 Å) and an ω-2θ scan were used fordata collection. The lattice parameters were determined using reflections in the range 22 < 2θ < 26˚. The structure was solved with direct methods using SHELXS-93 [15]. The refinement of the structure was performed by the full-matrix least square method on F2 for all the data with anisotropic thermal parameters for non-hydrogen atoms. CCDC reference number 865,395. The supplemen- tary crystallographic data can be obtained free of charge from the Cambridge Crystallographic Data Centre via www.ccdc.cam.ac.uk/data_request/cif. Molecular struc- ture of the compound showing the atomic numbering scheme is shown in Figure 1. The crystallography details for the structures determination of the compound were presented in Table 1. Selected bond distances and bond angles are listed in Table 2. 2.4. Quantum-Chemical Modeling The geometry optimization of isomers was performed by means of DFT-B3LYP/aug-cc-pVDZ approach. Elec- tronic absorption spectra were predicted using TDDFT approach at the same theory level as geometry optimiza- tion. In TDDFT calculations, the bulk solvent effects were taken into account by means of polarizable contin- uum model (PCM). All calculations have been executed using the “SKIF-Chebyshev” supercomputer of the Mos- cow State University with FireFly package available free at http://classic.chem.msu.su/gran/firefly/index.html. Figure 1. Molecular structure of I (O7-H7 represents 0.5 H2O). Table 1. Crystallographic data and structure refinement parameters. Empirical formula C7H10N2NaO7.5 Formula weight 265.16 g/mol Crystal color Dark red Temperature 293(2) K Wavelength 0.71073 Å Crystal system Monoclinic Space group C2/c Unit cell dimensions a = 16.408(3) Å, b = 12.446(3) Å, c = 13.716(3) Å, β = 126.34(3)˚ Volume 2256.1(8) Å3 Z, calculated density 8, 1.561 Mg·m–3 Absorption coefficient 0.172 mm–1 F(000) 1096 Crystal size 0.50 × 0.30 × 0.20 mm Theta range for data collection2.25 to 25.47 deg. Limiting indices –19 ≤ h ≤15, 0 ≤ k ≤14, –16 ≤ l ≤ 15 Reflections collected/unique2191/2100 [R(int) = 0.0147] Completeness to theta = 25.47100% Refinement method Full-matrix least-squares on F2 Data/restraints/parameters 2100/0/200 Goodness-of-fit on F2 1.056 Final R indices [I > 2 sigma (I)]R1 = 0.0280, wR2 = 0.0868 R indices (all data) R1 = 0.1160, wR2 = 0.1005 Largest diff. peak and hole 0.281 e·Å–3 and –0.294 e·Å–3 Copyright © 2012 SciRes. CSTA
 O. KOVALCHUKOVA ET AL. 48 Table 2. Selected bond lengths (Å) and angle s (˚). O(1)-C(1) 1.220(2) C(2)-N(1)-O(4) 117.52(14) O(2)-C(3) 1.271(2) C(6)-N(2)-O(5) 118.20(15) O(3)-C(5) 1.265(2) O(1)-C(1)-C(6) 122.54(15) O(4)-N(1) 1.3583(19) O(1)-C(1)-C(2) 121.51(15) O(5)-N(2) 1.348(2) C(6)-C(1)-C(2) 115.95(13) N(1)-C(2) 1.293(2) N(1)-C(2)-C(3) 125.73(15) N(2)-C(6) 1.292(2) N(1)-C(2)-C(1) 113.70(14) C(1)-C(6) 1.471(2) C(3)-C(2)-C(1) 120.43(14) C(1)-C(2) 1.483(2) O(2)-C(3)-C(4) 123.67(15) C(2)-C(3) 1.481(2) O(2)-C(3)-C(2) 115.34(15) C(3)-C(4) 1.395(3) C(4)-C(3)-C(2) 120.98(15) C(4)-C(5) 1.398(3) C(3)-C(4)-C(5) 120.86(15) C(4)-C(7) 1.503(2) C(3)-C(4)-C(7) 119.62(18) C(5)-C(6) 1.482(2) C(5)-C(4)-C(7) 119.52(18) O(3)-C(5)-C(4) 123.19(16) O(3)-C(5)-C(6) 115.84(16) C(4)-C(5)-C(6) 120.97(15) N(2)-C(6)-C(1) 113.49(14) N(2)-C(6)-C(5) 125.84(15) C(1)-C(6)-C(5) 120.62(15) 3. Results and Discussion Dark-red plates of single crystals of the only product of MPG nitrosation were obtained by re-crystallization from ethanolic aqueous solution, and the crystal structure was determined by X-ray diffraction studies. The title com- pound crystallizes in a hydrated form of a sodium salt (C7H5N2NaO5·2.5H2O) in the monoclinic space group C2/c with Z = 1. As it is evident (Figure 1, Table 2), the isolated prod- uct of transformation of MPG exists in the anionic form of a triketo-dihydroxyimino tautomer. The negative charge of the anion is neutralized by Na+ cations. The C–O distances in the planar organic anion (1.220(2); 1.271(2) and 1.265(2) Å for C1–O1, C3–O2 and C5–O3 respectively) as well as N1–C2 (1.293(2) Å) and N2–C6 (1.292(2) Å) are close to the corresponding double bonds. The C–C bonds of the ring are not equivalent: C1–C6, C1–C2, C2–C3, and C5–C6 bond lengths (1.471(2) - 1.483(2) Å) indicate a small degree of conjunction as well as C3–C4 (1.395(3) Å) and C4–C5 (1.398(3) Å) bonds are much shorter. Similar types of structures of polyketo-compounds of carbocyclic and heterocyclic series were reviewed in [16]. H4 and H5 atoms are located at O4 and O5 atoms of two hydroxyimino-groups of the anion and involved in the intramolecular H-bonds with the neighboring car- bonyl O-atoms (Table 3). The organic anion chelates the Na+ cations by the oxygen atoms of С=О groups and N-atoms of neighbor- ing oxime fragments (r O 1Na −25,647(15) Å; r N 1Na −26,625(16) Å). The О(1), N(2) atoms as well as the O-atoms of both oxime groups are also involved in coor- dination with the Na+ cations of the neighboring mole- cules. The coordination sphere of Na+ also includes two water molecules, one of which is bridging. One of H2O molecules is of the lattice nature. Thus, a 3D lattice is formed (Figure 2) which is linked by intermolecular H-bonds involving coordinated and lattice water mole- cules (Table 3). The organic anion chelates the Na+ cations by the oxygen atoms of С=О groups and N-atoms of neighbor- ing oxime fragments (r O 1Na −25,647(15) Å; r N 1Na −26,625(16) Å). The О(1), N(2) atoms as well as the O-atoms of both oxime groups are also involved in coor- dination with the Na+ cations of the neighboring mole- cules. The coordination sphere of Na+ also includes two water molecules, one of which is bridging. One of H2O molecules is of the lattice nature. Thus, a 3D lattice is formed (Figure 2) which is linked by intermolecular H-bonds involving coordinated and lattice water mole- cules (Table 3). Table 3. Hydrogen bonds in I (Å and deg. ). D-H...A d(D-H)d(H...A) d(D...A)<(DHA) O(4)-H(4)...O (2)#1 1.06(3)1.46(3) 2.442(2)151(3) O(5)-H(5)...O (3)#1 0.81(3)1.69(3) 2.470(2)161(3) O(7)-H(7)...O (8)#1 0.83(3)1.98(3) 2.797(2)165(3) O(6)-H(61).. .O(2)#40.76(3)2.03(3) 2.748(2)158(3) O(6)-H(62).. .O(8)#50.88(5)1.86(5) 2.732(2)169(4) O(8)-H(81).. .O(3)#60.77(4)2.01(4) 2.763(2)170(3) O(8)-H(82).. .O(6)#10.82(4)2.01(4) 2.737(2)147(4) Symmetry transformations used to generate equivalent atoms: #1 x, y, z #2 –x + 1, –y + 1, –z #3 – x + 1,y, –z + 1/2 #4 x, –y + 1, z – 1/2 #5 –x + 1/2, –y + 1/2, –z #6 x, y – 1, z. Figure 2. Fragment of the crystal structure of I. Copyright © 2012 SciRes. CSTA
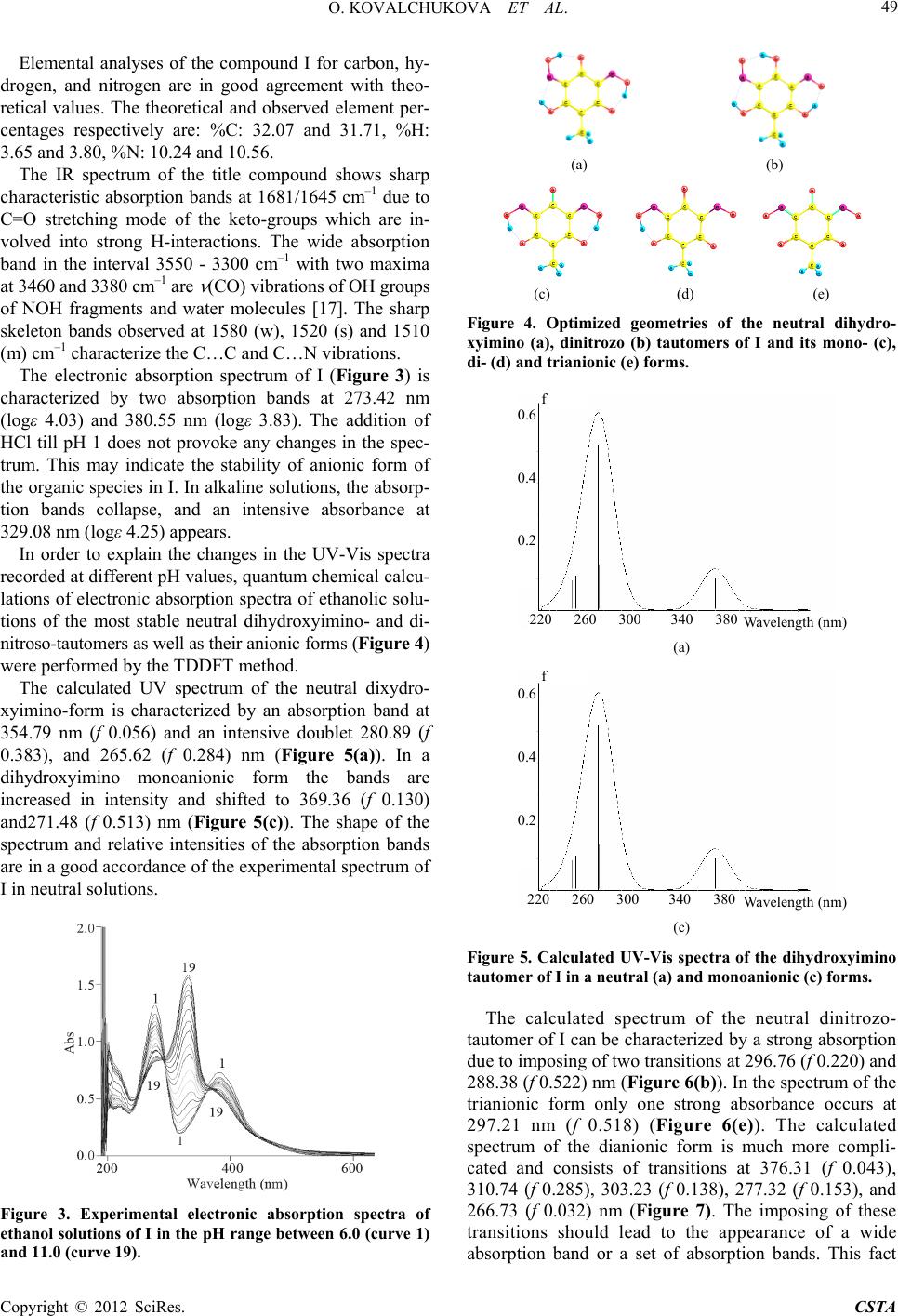 O. KOVALCHUKOVA ET AL. 49 Elemental analyses of the compound I for carbon, hy- drogen, and nitrogen are in good agreement with theo- retical values. The theoretical and observed element per- centages respectively are: %C: 32.07 and 31.71, %H: 3.65 and 3.80, %N: 10.24 and 10.56. The IR spectrum of the title compound shows sharp characteristic absorption bands at 1681/1645 cm–1 due to C=O stretching mode of the keto-groups which are in- volved into strong H-interactions. The wide absorption band in the interval 3550 - 3300 cm–1 with two maxima at 3460 and 3380 cm–1 are (CO) vibrations of OH groups of NOH fragments and water molecules [17]. The sharp skeleton bands observed at 1580 (w), 1520 (s) and 1510 (m) cm–1 characterize the C…C and C…N vibrations. The electronic absorption spectrum of I (Figure 3) is characterized by two absorption bands at 273.42 nm (logε 4.03) and 380.55 nm (logε 3.83). The addition of HCl till pH 1 does not provoke any changes in the spec- trum. This may indicate the stability of anionic form of the organic species in I. In alkaline solutions, the absorp- tion bands collapse, and an intensive absorbance at 329.08 nm (logε 4.25) appears. In order to explain the changes in the UV-Vis spectra recorded at different pH values, quantum chemical calcu- lations of electronic absorption spectra of ethanolic solu- tions of the most stable neutral dihydroxyimino- and di- nitroso-tautomers as well as their anionic forms (Figure 4) were performed by the TDDFT method. The calculated UV spectrum of the neutral dixydro- xyimino-form is characterized by an absorption band at 354.79 nm (f 0.056) and an intensive doublet 280.89 (f 0.383), and 265.62 (f 0.284) nm (Figure 5(a)). In a dihydroxyimino monoanionic form the bands are increased in intensity and shifted to 369.36 (f 0.130) and271.48 (f 0.513) nm (Figure 5(c)). The shape of the spectrum and relative intensities of the absorption bands are in a good accordance of the experimental spectrum of I in neutral solutions. Figure 3. Experimental electronic absorption spectra of ethanol solutions of I in the pH range between 6.0 (cur ve 1) and 11.0 (curve 19). (a) (b) (c) (d) (e) Figure 4. Optimized geometries of the neutral dihydro- xyimino (a), dinitrozo (b) tautomers of I and its mono- (c), di- (d) and trianionic (e) forms. f 220 260 300 340 380 Wavelength (nm) 0.6 0.4 0.2 (a) f 220 260 300 340 380 Wavelength (nm) 0.6 0.4 0.2 (c) Figure 5. Calculated UV-Vis spectra of the dihydroxyimino tautomer of I in a neutral (a) and monoanionic (c ) for ms. The calculated spectrum of the neutral dinitrozo- tautomer of I can be characterized by a strong absorption due to imposing of two transitions at 296.76 (f 0.220) and 288.38 (f 0.522) nm (Figure 6(b)). In the spectrum of the trianionic form only one strong absorbance occurs at 297.21 nm (f 0.518) (Figure 6(e)). The calculated spectrum of the dianionic form is much more compli- cated and consists of transitions at 376.31 (f 0.043), 310.74 (f 0.285), 303.23 (f 0.138), 277.32 (f 0.153), and 266.73 (f 0.032) nm (Figure 7). The imposing of these transitions should lead to the appearance of a wide absorption band or a set of absorption bands. This fact Copyright © 2012 SciRes. CSTA
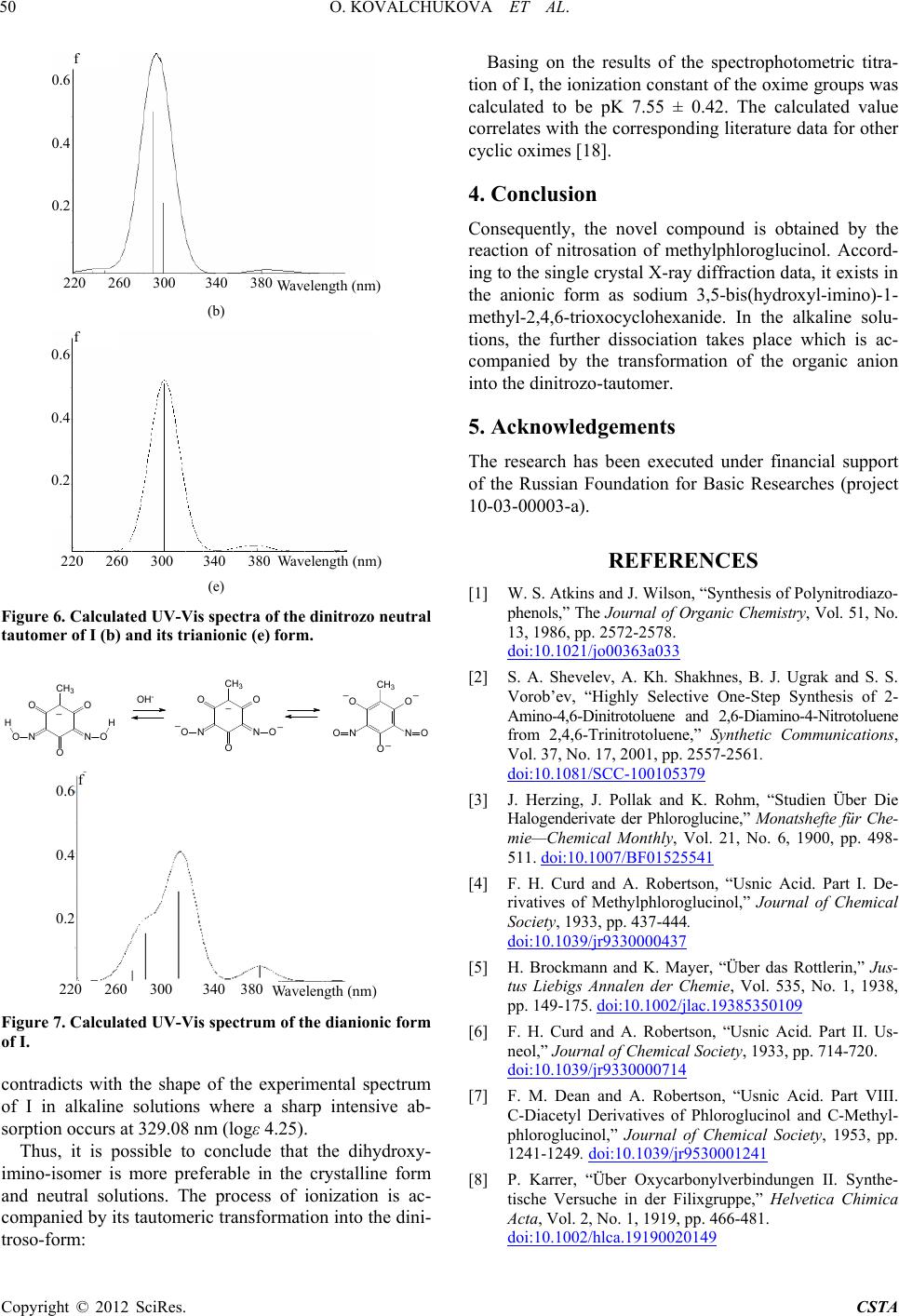 O. KOVALCHUKOVA ET AL. 50 f 220 260 300 340 380 Wavelength (nm) 0.6 0.4 0.2 (b) f 220 260 300 340 380 Wavelength (nm) 0.6 0.4 0.2 (e) Figure 6. Calculated UV-Visctra of the dinitrozo n speeutral tautomer of I (b) and its trianionic (e) form. CH3 OO NNO H O H O _OH- CH3 OO N O NOO _ _ _ O NO CH3 OO NO __ _ 220 260 300 340 380 Wavelength (nm) 0.6 0.4 0.2 Figure 7. Calculated UV-Vis spectrum of the dianion form ontradicts with the shape of the experimental spectrum the dihydroxy- im the results of the spectrophotometric titra- tio 4. Conclusion novel compound is obtained by the 5. Acknowledgements nancial support REFERENCES [1] W. S. Atkins a of Polynitrodiazo- ic of I. c of I in alkaline solutions where a sharp intensive ab- sorption occurs at 329.08 nm (logε 4.25). Thus, it is possible to conclude that ino-isomer is more preferable in the crystalline form and neutral solutions. The process of ionization is ac- companied by its tautomeric transformation into the dini- troso-form: Basing on n of I, the ionization constant of the oxime groups was calculated to be pK 7.55 ± 0.42. The calculated value correlates with the corresponding literature data for other cyclic oximes [18]. Consequently, the reaction of nitrosation of methylphloroglucinol. Accord- ing to the single crystal X-ray diffraction data, it exists in the anionic form as sodium 3,5-bis(hydroxyl-imino)-1- methyl-2,4,6-trioxocyclohexanide. In the alkaline solu- tions, the further dissociation takes place which is ac- companied by the transformation of the organic anion into the dinitrozo-tautomer. The research has been executed under fi of the Russian Foundation for Basic Researches (project 10-03-00003- а). nd J. Wilson, “Synthesis phenols,” The Journal of Organic Chemistry, Vol. 51, No. 13, 1986, pp. 2572-2578. doi:10.1021/jo00363a033 [2] S. A. Shevelev, A. Kh. Shakhnes, B. J. Ugrak and S. S. Vorob’ev, “Highly Selective One-Step Synthesis of 2- Amino-4,6-Dinitrotoluene and 2,6-Diamino-4-Nitrotoluene from 2,4,6-Trinitrotoluene,” Synthetic Communications, Vol. 37, No. 17, 2001, pp. 2557-2561. doi:10.1081/SCC-100105379 [3] J. Herzing, J. Pollak and K. Rohm, “Studien Über Die Halogenderivate der Phloroglucine,” Monatshefte für Che- mie—Chemical Monthly, Vol. 21, No. 6, 1900, pp. 498- 511. doi:10.1007/BF01525541 [4] F. H. Curd and A. Robertson, “Usnic Acid. Part I. De- rivatives of Methylphloroglucinol,” Journal of Chemical Society, 1933, pp. 437-444. doi:10.1039/jr9330000437 [5] H. Brockmann and K. Mayer, “Über das Rottlerin,” Jus- tus Liebigs Annalen der Chemie, Vol. 535, No. 1, 1938, pp. 149-175. doi:10.1002/jlac.19385350109 [6] F. H. Curd and A. Robertson, “Usnic Acid. Part II. Us- neol,” Journal of Chemical Society, 1933, pp. 714-720. doi:10.1039/jr9330000714 [7] F. M. Dean and A. Robertson, “Usnic Acid. Part VIII. C-Diacetyl Derivatives of Phloroglucinol and C-Methyl- phloroglucinol,” Journal of Chemical Society, 1953, pp. 1241-1249. doi:10.1039/jr9530001241 [8] P. Karrer, “Über Oxycarbonylverbindungen II. Synthe- tische Versuche in der Filixgruppe,” Helvetica Chimica Acta, Vol. 2, No. 1, 1919, pp. 466-481. doi:10.1002/hlca.19190020149 Copyright © 2012 SciRes. CSTA
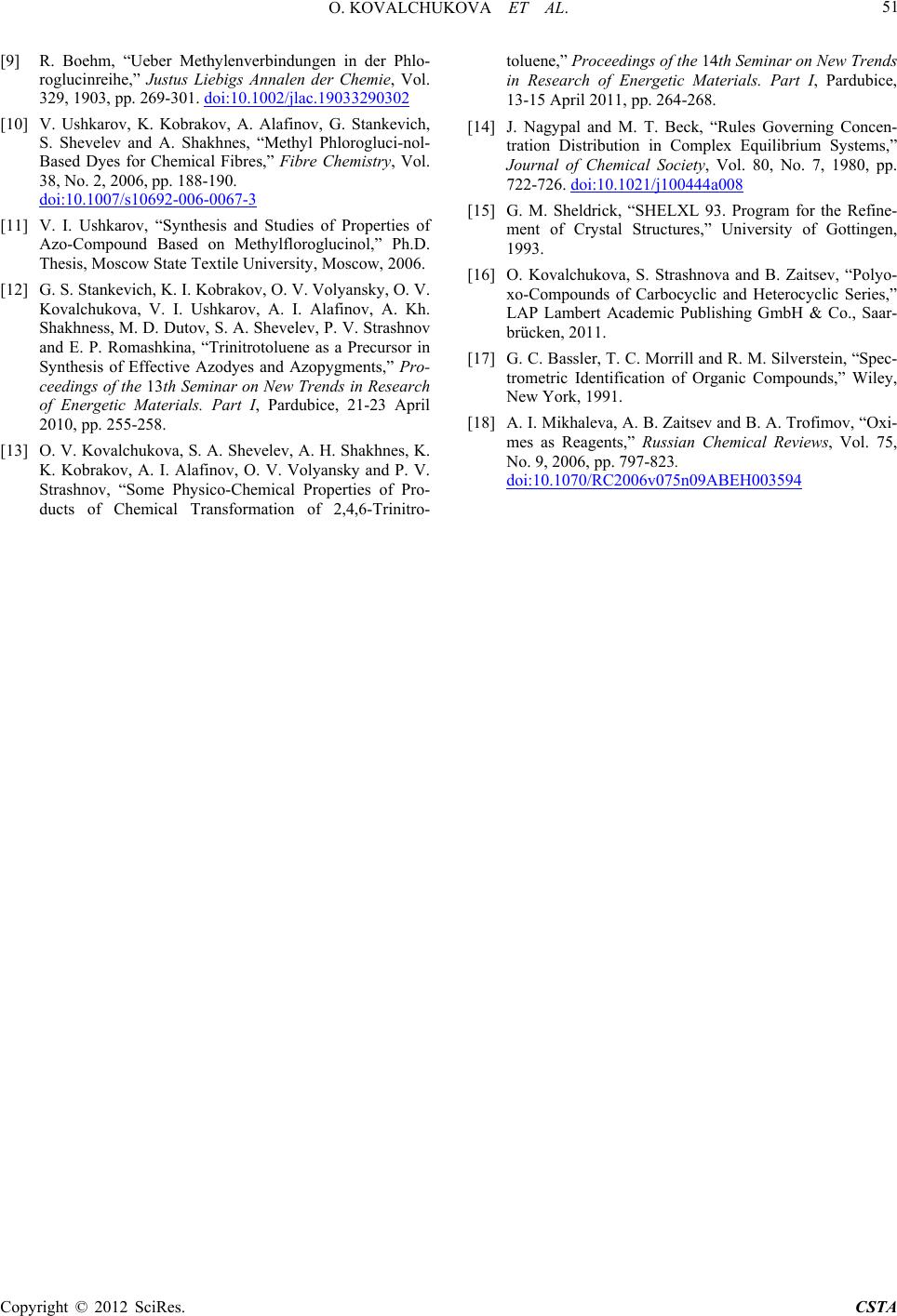 O. KOVALCHUKOVA ET AL. Copyright © 2012 SciRes. CSTA 51 rbindungen in der Phlo- [9] R. Boehm, “Ueber Methylenve roglucinreihe,” Justus Liebigs Annalen der Chemie, Vol. 329, 1903, pp. 269-301. doi:10.1002/jlac.19033290302 [10] V. Ushkarov, K. Kobrakov, A. Alafinov, G. Stankevich, S. Shevelev and A. Shakhnes, “Methyl Phlorogluci-nol- Based Dyes for Chemical Fibres,” Fibre Chemistry, Vol. 38, No. 2, 2006, pp. 188-190. doi:10.1007/s10692-006-0067-3 [11] V. I. Ushkarov, “Synthesis and Studies of Properties of Azo-Compound Based on Methylfloroglucinol,” Ph.D. Thesis, Moscow State Textile University, Moscow, 2006. [12] G. S. Stankevich, K. I. Kobrakov, O. V. Volyansky, O. V. Kovalchukova, V. I. Ushkarov, A. I. Alafinov, A. Kh. Shakhness, M. D. Dutov, S. A. Shevelev, P. V. Strashnov and E. P. Romashkina, “Trinitrotoluene as a Precursor in Synthesis of Effective Azodyes and Azopygments,” Pro- ceedings of the 13th Seminar on New Trends in Research of Energetic Materials. Part I, Pardubice, 21-23 April 2010, pp. 255-258. [13] O. V. Kovalchukova, S. A. Shevelev, A. H. Shakhnes, K. ules Governing Concen- K. Kobrakov, A. I. Alafinov, O. V. Volyansky and P. V. Strashnov, “Some Physico-Chemical Properties of Pro- ducts of Chemical Transformation of 2,4,6-Trinitro- toluene,” Proceedings of the 14th Seminar on New Trends in Research of Energetic Materials. Part I, Pardubice, 13-15 April 2011, pp. 264-268. [14] J. Nagypal and M. T. Beck, “R tration Distribution in Complex Equilibrium Systems,” Journal of Chemical Society, Vol. 80, No. 7, 1980, pp. 722-726. doi:10.1021/j100444a008 [15] G. M. Sheldrick, “SHELXL 93. Program for the Refine- alchukova, S. Strashnova and B. Zaitsev, “Polyo- . C. Morrill and R. M. Silverstein, “Spec- Zaitsev and B. A. Trofimov, “Oxi- ABEH003594 ment of Crystal Structures,” University of Gottingen, 1993. [16] O. Kov xo-Compounds of Carbocyclic and Heterocyclic Series,” LAP Lambert Academic Publishing GmbH & Co., Saar- brücken, 2011. [17] G. C. Bassler, T trometric Identification of Organic Compounds,” Wiley, New York, 1991. [18] A. I. Mikhaleva, A. B. mes as Reagents,” Russian Chemical Reviews, Vol. 75, No. 9, 2006, pp. 797-823. doi:10.1070/RC2006v075n09
|