 Journal of Biomaterials and Nanobiotechnology, 2012, 3, 547-556 http://dx.doi.org/10.4236/jbnb.2012.324057 Published Online October 2012 (http://www.SciRP.org/journal/jbnb) 547 Study of the Adhesion of Clinical Strains of Staphylococcus aureus on an Abiotic Surface Using the Biofilm Ring Test® J. M. Liesse Iyamba1,2, N. B. Takaisi-Kikuni2, S. Dulanto1, J. P. Deh ay e 1 1Laboratoire de Chimie biologique et médicale et de Microbiologie pharmaceutique, Faculté de Pharmacie, Université libre de Bruxelles, Brussels, Belgium; 2Laboratoire de Microbiologie Expérimentale et Pharmaceutique, Faculté des Sciences Pharmaceu- tiques, Université de Kinshasa, Kinshasa, Democratic Republic of Congo. Email: jliessei@ulb.ac.be Received June 14th, 2012; revised July 25th, 2012; accepted August 14th, 2012 ABSTRACT Four methicillin-sensitive (MSSA) and 4 methicillin-resistant (MRSA) strains of Staphylococcus aureus were collected and isolated at the Laboratory of Bacteriology of the Provincial General Reference Hospital of Kinshasa in the Democ- ratic Republic of Congo. The microbial adhesion to solvents (MATS) test showed that the MRSA strains had a less hy- drophobic membrane than the MSSA strains. Using the Biofilm Ring Test® (BFRT®) to investigate on the adhesion of these bacterial strains to smooth surfaces, we observed that the MSSA strains adhered more rapidly than the MRSA strains. The biomass of the produced biofilm measured by the Crystal violet staining method (CVSM) was more impor- tant with MSSA than with MRSA strains. Ethylene glycol-bis(2-aminoethylether)-N,N,N',N'-tetraacetic acid (EGTA) inhibited the adhesion and the formation of a biofilm by MRSA strains; this inhibition was reversed by calcium, mag- nesium and manganese. The MRSA strains adhered less to silicon tubing and the adhesion was inhibited by EGTA in 2 of the 4 MRSA strains and none of the MSSA strains. In conclusion, the MSSA and MRSA strains adhered on an abiotic surface and formed a biofilm at distinct rates and with different sensitivities to ions. The results also confirm the utility as well as the limits of the BFRT® to study the adhesion of bacteria on a surface. Keywords: Biofilm Ring Test®; Crystal Violet; Cell Surface; Hydrophobicity; Adhesion; Catheter Tube 1. Introduction Staphylococcus aureus is a human pathogen that causes both chronic and nosocomial infections; many of which are mediated by their ability to adhere to medical devices and to form biofilms. This bacteria is responsible of a large variety of diseases, including endocarditis, osteo- myelitis, and foreign body infections [1]. A biofilm is de- fined as aggregated, microbial cells surrounded by a polymeric self-produced matrix, which may contain host components [2]. According to the Center for Disease Control and Prevention, 65% of human bacterial infec- tions are associated to a biofilm [3]. Furthermore, biofilms infections are a major problem in the clinic and cause many deaths and high health costs, e.g. 12% to 25% of patient mortality are attributable to catheter-related blood- stream infections [4]. Biofilm-associated bacteria are ge- nerally resistant to antibiotics [5], and to host immune responses [6]. Two main stages are involved in S. aureus biofilm formation [7]. In a first step, the cells adhere on an abiotic or a biotic surface. The adherence of S. aureus to foreign bodies depends on the cell surface characteristics of the micro-organisms and the nature of foreign body material and involves physicochemical forces such as polarity, London-van der Waals forces and hydrophobic interactions [8]. Divalent cationic ions (e.g. magnesium, calcium) may enhance the attachment of bacteria to sur- face by reducing electrostatic repulsion and stabilizing interaction between the negatively charged surface of bacteria and anionic substrates [9]. Cell surface hydro- phobicity and initial adherence of micro-organisms to a surface have been attributed to different bacterial surface- associated adhesins. The attachment of S. aureus on a biotic surface is likely to be mediated by cell-wall asso- ciated proteins such as the microbial surface components recognizing adhesive matrix molecules (MSCRAMM). The second stage of biofilm development includes cells multiplication and formation of a mature structure con- sisting of many cell layers. This stage is associated with the production of extracellular factors which may include exo-polysaccharides, proteins and extracellular DNA (eDNA) [10]. Excretion of polysaccharide intercellular adhesion (PIA) polymers in Staphylococcus species and the presence of divalent cations interact to form stronger Copyright © 2012 SciRes. JBNB
 Study of the Adhesion of Clinical Strains of Staphylococcus aureus on an Abiotic Surface Using the Biofilm Ring Test® 548 bonding between cells [11]. The detachment of cells from established biofilms allows the spreading and the colonization of new sites by planctonic bacteria [12]. Colorimetric microtiter plate systems are widely used to determine bacterial adhesion [13]. Among these tech- niques, the Crystal Violet staining method (CVSM) has been modified to increase its accuracy and to allow the quantification of the biomass of the biofilm in the entire well [14]. Despite the fact that this method is suitable for estimating the adherent cells stained after washing steps, it requires cultures of at least 24 to 48 h. The Biofilm Ring Test® (BFRT®), a newly described method, has been recently proposed as an alternative to the CVSM for studying bacterial adhesion [15]. This technique based on the immobilization of magnetic beads by adherent cells had not only been implemented to study the initial steps of biofilm formation [16,17], but also to explore the composition of Staphylococcus aureus and Leuconostoc mesenteroides biofilm matrix [15,18]. The aim of this study was to compare the adhesion of clinical methicil- lin-resistant Staphylococcus aureus (MRSA) and methi- cillin-sensitive Staphylococcus aureus (MSSA) strains and to determine the effect of divalent cations on the inhibition of bacterial adhesion by ethylene glycol-bis(2- aminoethylether)-N,N,N' ,N'- tetraacetic acid (EGTA). 2. Materials and Methods 2.1. Origin of the Strains and Growth Conditions The 8 strains used in this study were collected for diag- nostic purposes in the Laboratory of Bacteriology of the Provincial General Reference Hospital of Kinshasa (HGPRK) in the Democratic Republic of Congo. Among the 4 MSSA strains, 2 strains (the 5668/B and 5741/B strains) were isolated from blood samples and the 2 other strains (the 1532/SW and 1620/SW) were from skin wounds. Among the 4 MRSA strains, 2 strains (the 007/FV and the 028/FV) were collected from a vaginal smear test, 1 strain (the 011/LP) from prostatic fluid and 1 strain (027/U) from urine. The bacteria were grown and iso- lated on Brain Heart Agar with 5% (v/v) sheep blood and on Mannitol Salt Agar (Difco, BD Franklin Lakes, NJ, USA). The identification of S. aureus was performed with the latex agglutination test (Pastorex Staph-Plus, Biorad, Marnes-la-Coquette, France). All MRSA strains were positive for mecA gene (data not shown). 2.2. Characterization of Cell Surface Properties of the Strains The microbial adhesion to solvents (MATS) test was performed to estimate, simultaneously, the hydrophobic- ity and the electron-acceptor and electron-donor charac- teristics of the bacterial membranes [19]. Briefly, the bacteria strains were cultivated on TSA medium and in- cubated for 24 h at 37˚C. TSB medium was inoculated with 2 or 3 colonies and incubated for 24 h at 37˚C. The bacteria were then harvested by centrifugation and washed twice with 150 mM potassium phosphate. The bacteria were suspended in the same buffer and adjusted to a concentration of ~108 CFU/mL. An aliquot of this suspension (2.4 mL) was mixed with 0.4 mL of one of 2 pairs of organic solvents and vigorously shaken by vor- texing for 2 min. Each pair of polar and non-polar sol- vents had similar Lifshitz-van der Waals surface tension properties. The two pairs of solvents tested were 1) chloroform (an acidic solvent) and hexadecane and 2) ethyl acetate (a strong basic solvent) and hexane, its n-alkane apolar control [19]. The mixture was allowed to stand for 15 min to ensure complete separation of the two phases. The OD of the aqueous phase was measured at 400 nm. The adhesion to each solvent was calculated by the equation: % adhesion = (1 − A/A0) × 100, where A0 was the absorbance of the bacterial suspension (aqueous phase) before mixing and A was the absorbance after mixing. The experiment was repeated at least 3 times for each strain. 2.3. Evaluation of Bacterial Adherence in a Smooth Surface Using the BFRT® The Biofilm Ring Test® (BFRT®) is based on the immo- bilization of the magnetic beads by adherent cells. Con- sequently, the more beads are entrapped by cells, the fewer they are detectable after magnetization and, sub- sequently, the more cells are attached to the surface [15]. Bacterial adhesion was assessed using an appropriate kit commercially available (Biofilm Control, Saint Beauzire, France). The toner solution (TON005) containing nega- tively charged magnetic beads of 1 - 3 µm was mixed with a calibrated Initial Bacterial Suspension (IBS) con- taining ~107 bacteria/mL. Two hundred µL of this mix- ture were placed in the wells of a 96-wells polystyrene plate formed by the assembly of 12 individual 8-wells strips (Strip Well MSW002B). After incubation time at 37˚C, wells of the strip were covered with a few drops of contrast liquid (inert opaque oil used for the reading step) and scanned with the plate reader to get Io image. Then, the plate was placed for 1 min on the magnet (Blok test) and scanned again to get I1 image. The adhesion capabil- ity of each strain was expressed as the Biofilm Index (BFI) calculated by the software and based on the com- parison of the two images. When BFI ≥ 7, a spot corre- sponding to the microbeads attracted by the magnet is visible and indicates the absence of adhesion or biofilm. When BFI ≤ 2, no spot can be observed after magnetiza- Copyright © 2012 SciRes. JBNB
 Study of the Adhesion of Clinical Strains of Staphylococcus aureus on an Abiotic Surface Using the Biofilm Ring Test® 549 tion indicating that microbeads are blocked by adherent cells. Biofilm in formation results in microbeads partially blocked 2 ≤ BFI ≤ 7. The experiment was repeated at least 3 times for each strain. 2.4. Evaluation of the Formation of a Biofilm with the CVSM Polystyrene sterile strips were inoculated with 200 µL of IBS and incubated for various times at 35˚C in a humid atmosphere. A control well was inoculated with sterile medium. Each strain was evaluated in triplicate. Medium was removed from the wells which were washed 3 times with 200 µL sterile distilled water. The strips were air- dried for 45 min and the adherent cells were stained with 200 µL of 0.1% Crystal violet solution. After 45 min, the dye was eliminated and the wells were washed 5 times with 300 µL of sterile distilled water to remove excess stain. The dye incorporated by the cells forming a biofilm was dissolved with 200 µL of 33% (v/v) glacial acetic acid and the absorbance of each well was read at 540 nm in the microplate reader. The results were expressed as variation of OD540 nm (OD540 nm sample - OD540 nm control). The experiment was repeated at least 3 times, for each strain and incubation time. 2.5. Study of the Adhesion of the Bacteria on Catheter Tubing The bacteria were grown overnight and the OD600 nm ad- justed to 1.00 ± 0.05. They were then diluted 250-fold. A silicon tubing (2 cm long, 3 mm inner diameter) was in- cubated with the bacteria at 37˚C for 18 h. At the end of the incubation, the tubes were rinsed twice with water before adding 1 mL phosphate-buffer solution (pH 7.2). The cells were detached from the tubing by incubation in an ultrasound bath at 25˚C for 5 min. After serial dilu- tions of the bacterial suspension with PBS, the bacteria were plated on Petri dishes containing 15 mL TSA me- dium and the Petri dishes were incubated at 35˚C for 48 h before counting the colonies. Dishes with less than 50 colonies or with more than 500 colonies were not count- ed. Results are expressed as colonies forming units per mL (CFU/mL). 2.6. Statistical Analysis Results were analyzed with the Mann-Whitney non-pa- rametric test. ***P < 0.005; **P < 0.01; *P < 0.05. 3. Results 3.1. Measure of the Adhesion of the Strains to Solvents The hydrophobicity and the electron-donor and electron- acceptor properties of the cell walls of the various strains were tested according to Bellon-Fontaine et al. [19]. The 4 MSSA strains migrated nearly totally (from 90% to 97%) from the aqueous to the hexadecane phase establishing that their membrane was very hydrophobic. The 4 MRSA strains migrated slightly less (from 80% to 83%). The dif- ference between MSSA (93.1% ± 1.4%) and MRSA strains (82.1% ± 1.2%) was significant (P < 0.001, n = 12) suggesting that MRSA strains were slightly less hydro- phobic than MSSA strains (Figures 1(a) and (b)). The bacteria were also extracted with chloroform. The extrac- tion of MSSA strains (95% ± 0.6%) was better than the extraction of MRSA strains (82.1% ± 1.5 %) (P < 0.001, n = 12) showing that the cell walls of MSSA strains were more basic (electron-donor capacity) than cell walls from MSSA strains A TCC 259235668/S 5741/S1532/P 1620 /P 0 25 50 75 100 125 Chloroform Hexadecane Ethyl acetate He xane (a) Strains % bacteria extracted by the solvent (a) MRSA strains A T CC 33 5 910011/LP027/U 028/FV007/FV 0 25 50 75 100 125 Chloroform Hexadecane Hexane Ethyl acetate (b ) Strains % bacteria extracted by the solvent (b) Figure 1. Study of the interaction of S. aureus with organic solvents. The affinity of 4 MSSA strains (a) or 4 MRSA strains (b) for chloroform, hexadecane, ethyl acetate or hexane was measured. Results are expressed as percent- ages of bacteria transferred from the aqueous phase to the organic solvent. Values are the means ± s.e.m. of 3 ex- periments. Copyright © 2012 SciRes. JBNB
 Study of the Adhesion of Clinical Strains of Staphylococcus aureus on an Abiotic Surface Using the Biofilm Ring Test® 550 MRSA strains. The comparison of the extraction of the strains by ethyl acetate and hexane gave an estimate of the electron-accepting property of the strains. The 2 sol- vents extracted comparable percentages of the 4 MSSA strains showing that the cell walls of these bacteria had no electron-accepting propensity; ethyl acetate extracted the 4 MRSA strains 3-times less than hexane and it could be concluded that their walls were rather acidic. 3.2. Comparison between the BFRT® and the CVSM to Evaluate the Adhesion of the Bacteria In a next experiment, the adhesion of the 8 strains on an abiotic surface (the bottom of the wells of a multi-well plate) was estimated using the BFRT® and the CVSM. As shown in Figure 2(a), the 4 MSSA strains immobi- lized the magnetic beads of the BFRT®. Two MSSA strains (1620/SW and 1538/SW) fully blocked the beads within 2 h (drop of the BFRT® from 17.0 ± 0.1 to 2.4 ± 0.3, n = 18). The drop of the BFI was slightly slower for the 5668 and the 5741 strains (from 17.6 ± 0.2 to 9.3 ± 1.0 after 2 h). The BFI further dropped for the next 2 hours and reached 1.4 ± 0.1 after 4 h. The 4 MRSA stains immobilized the beads at a slower rate (Figure 2b). After 2 hours, 3 strains (011/LP, 027/U and 028/FV) decreased the BFI from 15.5 ± 0.9 to 9.1 ± 0.6, n =27). These strains fully blocked the beads after 3 h (BFI: 2.1 ± 0.1, n = 27). One strain (007/FV) was much less effective and significantly blocked the beads only after 4 h (7.3 ± 1.6, n = 7). Similar experiments were performed in the wells of a multi-well plate and the biomass adhering at the bottom of the wells was assayed after staining with Crystal violet. As shown in Figures 3(a) and (b), the formation of a biomass became significant after 3 h and differed among the strains. Some MSSA strains produced a very significant biomass after 4 h (5668/B > 1620/SW > 1532/SW > 5741/B). Among the MRSA strains, the 007/FV strain did not produce any significant biofilm after 4 h. From these results it could be concluded that the results obtained with the BFRT® and the CVSM were consistent but that the BFRT® was more efficient for the study of short-term effects. 3.3. Effect of Ions on the Formation of a Biofilm Divalent cations contribute to the interaction among bac- teria or their interaction with components of the ex- tracellular matrix [20]. The purpose of these experiments was to test the effect of EGTA, a chelator of divalent cations, on the adhesion of bacteria and the interaction between EGTA and divalent cations. Preliminary ex- periments were performed to test whether these ionic conditions affected by themselves the mobility of the MSSA strains 0 1 2 3 4 0 4 8 12 16 20 5668/B 5741/B 1532/SW 1620/SW (a) Ti me (hours ) BFI (arbitr a ry u n its ) (a) MRS A stra i n s 0 12 3 4 0 4 8 12 16 20 007/FV 027/U 028/FV 011/LP (b) Time ( h ours) BFI (arbi t rary units) (b) Figure 2. Time-course of the immobilization of magnetic beads by clinical strains of S. aureus. MSSA strains (a) and MRSA strains (b) of S. aureus were incubated at 35˚C in the presence of magnetic beads. The immobilization of the beads was measured after 1, 2, 3 or 4 h using the Biofilm Ring Test®. Results are expressed as the Biofilm Index (BFI). Data are the means ± s.e.m. of 3 experiments. beads. As shown in Figure 4(a), EGTA (from 100 µM to 1 mM) had no effect on the BFI. One mM calcium, magnesium and manganese decreased the BFI below 2. Only 1 mM EGTA was tested on the adhesion of the bacteria using the BFRT®. At this concentration, EGTA has no effect on the viability and the doubling time of the tested strains (data not shown). The bacteria were ex- posed to the chelator for 6 h. As shown in Figure 4(b), the metal-chelator had no effect on the drop of the BFI provoked by the 4 MSSA strains. EGTA increased the BFI measured in the presence of 3 MRSA strains (the 011/LP, 027/U and 028/FV strains). The 007/FV was not affected by EGTA (Figure 4(c)). Considering the inter- action of the ions with the BFRT®, the reversibility of the inhibition by EGTA was tested using the CVSM. The 4 MRSA strains were cultured for 24 h in control condi- tions or in the presence of 1 mM EGTA, in the absence Copyright © 2012 SciRes. JBNB
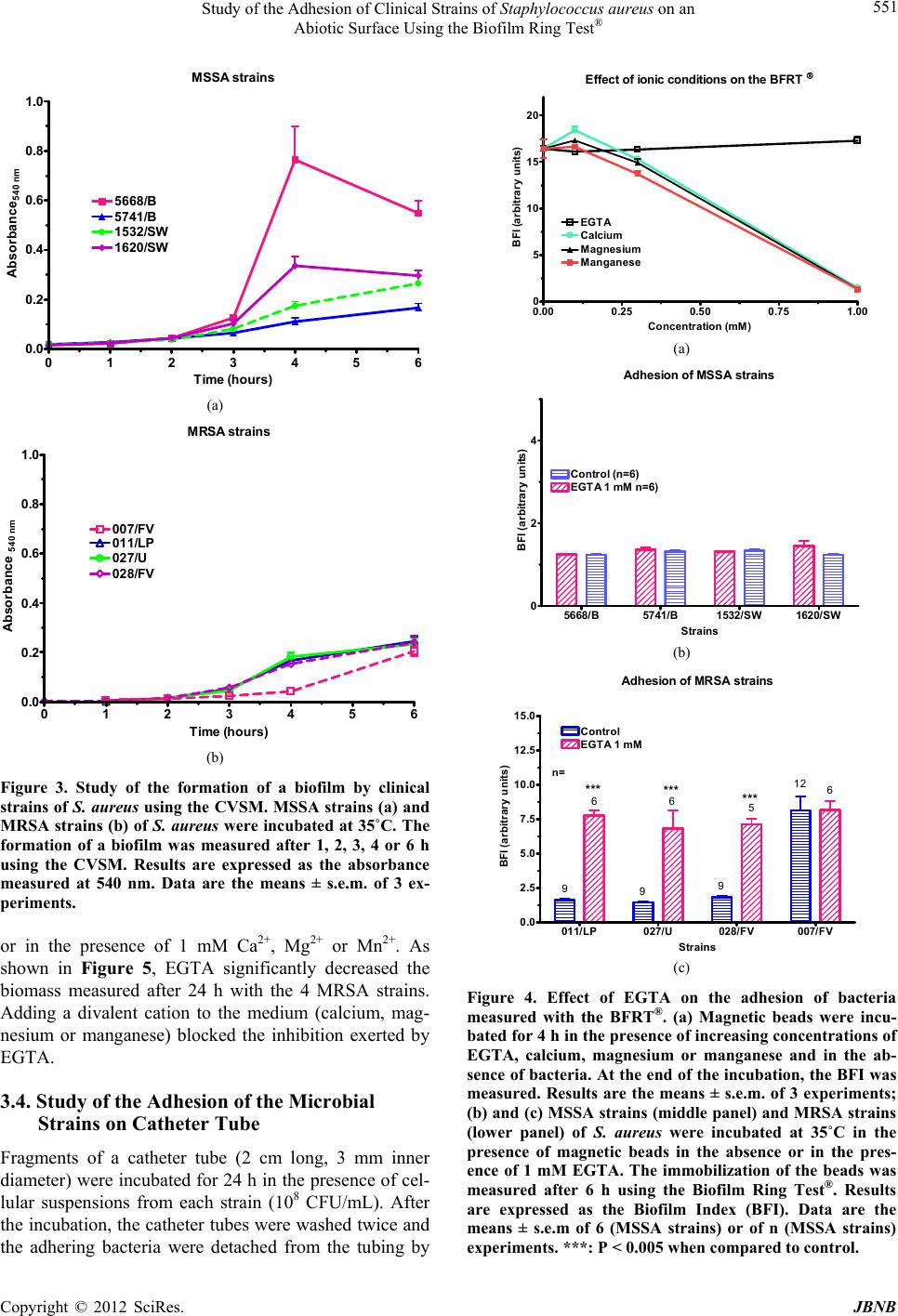 Study of the Adhesion of Clinical Strains of Staphylococcus aureus on an Abiotic Surface Using the Biofilm Ring Test® 551 MSSA strains 0 1 2 3 4 5 6 0.0 0.2 0.4 0.6 0.8 1.0 1620/SW 5741/B 5668/B 1532/SW (a) Ti me (ho u r s ) Absorbance 540 nm (a) MRSA strains 0 1 2 3 4 5 6 0.0 0.2 0.4 0.6 0.8 1.0 007/FV 027/U 011/LP 028/FV (b) Ti me (ho u r s ) Absorbance 540 nm (b) Figure 3. Study of the formation of a biofilm by clinical strains of S. aureus using the CVSM. MSSA strains (a) and MRSA strains (b) of S. aureus were incubated at 35˚C. The formation of a biofilm was measured after 1, 2, 3, 4 or 6 h using the CVSM. Results are expressed as the absorbance measured at 540 nm. Data are the means ± s.e.m. of 3 ex- periments. or in the presence of 1 mM Ca2+, Mg2+ or Mn2+. As shown in Figure 5, EGTA significantly decreased the biomass measured after 24 h with the 4 MRSA strains. Adding a divalent cation to the medium (calcium, mag- nesium or manganese) blocked the inhibition exerted by EGTA. 3.4. Study of the Adhesion of the Microbial Strains on Catheter Tube Fragments of a catheter tube (2 cm long, 3 mm inner diameter) were incubated for 24 h in the presence of cel- lular suspensions from each strain (108 CFU/mL). After the incubation, the catheter tubes were washed twice and the adhering bacteria were detached from the tubing by Effe ct of i onic con dit io n s on th e BFRT 0.00 0.25 0.50 0.751.00 0 5 10 15 20 C alc iu m Magnesium Mang anes e EGTA (a) Concentration (mM) BFI (arbitrary units) (a) Adhesion of MSSA strains 5668/B5741/B1532/SW 1620/SW 0 2 4 EGTA 1 m M n=6) Control (n=6) (b) Strains BF I (arb i trary unit s) (b) Adhesion of MRS A strains 011/LP027/U028/FV 007/FV 0.0 2.5 5.0 7.5 10.0 12.5 15.0 EGTA 1 mM Control 999 12 666 5 n= *** *** *** (c) Strains BFI (arbitrary units) (c) Figure 4. Effect of EGTA on the adhesion of bacteria measured with the BFRT®. (a) Magnetic beads were incu- bated for 4 h in the presence of increasing concentrations of EGTA, calcium, magnesium or manganese and in the ab- sence of bacteria. At the end of the incubation, the BFI was measured. Results are the means ± s.e.m. of 3 experiments; (b) and (c) MSSA strains (middle panel) and MRSA strains (lower panel) of S. aureus were incubated at 35˚C in the presence of magnetic beads in the absence or in the pres- ence of 1 mM EGTA. The immobilization of the beads was measured after 6 h using the Biofilm Ring Test®. Results are expressed as the Biofilm Index (BFI). Data are the means ± s.e.m of 6 (MSSA strains) or of n (MSSA strains) xperiments. ***: P < 0.005 when compared to control. e Copyright © 2012 SciRes. JBNB
 Study of the Adhesion of Clinical Strains of Staphylococcus aureus on an Abiotic Surface Using the Biofilm Ring Test® Copyright © 2012 SciRes. JBNB 552 011 /LP CONT Ca2+ Mg2+ Mn2+ 0.00 0.05 0.10 0.15 0.20 Control (n=3) EGTA 1 mM (n=6) * (a ) Absorbance 540 nm 027/U CONT Ca2+ Mg2+ Mn2+ 0.00 0.05 0.10 0.15 0.20 Control (n= 3) EGTA 1 mM (n=6) * (b ) Absorbance540 nm (a) (b) 028/FV CONT Ca2+ Mg2+ Mn2+ 0.00 0.05 0.10 0.15 0.20 Con tr ol (n=3) EGTA 1 mM (n=6) * (c) Absorbance540 nm 007/FV CONT Ca2+ Mg2+ Mn2+ 0.00 0.05 0.10 0.15 0.20 Control EGTA 1 mM * (d ) Absorbance540 nm Control (n = 3) EGTA 1 mM (n = 6) (a) (d) Figure 5. Reversibility of the inhibition by EGTA of the formation of a biofilm by MRSA strains. The 4 MRSA strains of S. aureus were incubated at 35˚C in the control conditions or in the presence of 1 mM EGTA, in the absence or in the presence of 1 mM calcium, magnesium or manganese. The formation of a biofilm was measured after 24 h using the CVSM. Results are expressed as the absorbance measured at 540 nm. Data are the means ± s.e.m. of n experiments. *: P < 0.05. sonication for 5 minutes in an ultrasound bath. The MSSA strains adhered more on the tubing (1.095 × 107 ± 5.37 × 106) than the MRSA strains (2.4 × 105 ± 6.75 × 104). Adding EGTA to the culture medium in- creased the adhesion of the MSSA strains (1.176 × 108 ± 1.59 × 107) and decreased the adhesion of MRSA strains (7.67 × 104 ± 2.24 × 104). The ANOVA analysis of the results followed by the Bonferroni post test confirmed that the adhesion of the MSSA strains was significantly more important than the adhesion of MRSA strains. EGTA had no significant effect on either group. Consid- ering the large variations of the measurements, the results for each strain were analyzed. As shown in Figures 6(a)-(d), the 4 MSSA strains were comparable and there was no effect of EGTA on either strain. Two MRSA strains (007/U and 028/FV) were not affected by EGTA. The chelator inhibited the adhesion of the 2 other MRSA strains (011/LP and 027/FV). 4. Discussion In this work, we studied the interaction of clinical strains of S. aureus with an abiotic surface. We show that the BFRT® was a more rapid method than the CVSM to de- tect the adhesion of the bacteria to the surface. MSSA strains adhered more rapidly and the interaction of MRSA strains with an abiotic surface was inhibited by EGTA. Calcium, magnesium and manganese reversed the inhibition exerted by EGTA. Different methods have been used to evaluate the ad- hesion of bacterial strains. Bacterial adhesion and the early stage of biofilm formation can be studied using reactors developed for the cultivation of biofilms like flow cells [21,22], annular biofilm reactors [23], fowler cells [24], modified Robbins devices [25], CDC biofilm reactors [26,27]. Disadvantages of such flow cell devices include limited sample area, and if operated on once- through flow basis, the potential exists for gradients in biofilm amount and fluid phase concentrations to develop in longitudinal direction. The examination and charac- terization of biofilms in medical devices, and human in- fections can also be performed using microscopy tech- niques (transmission electron, scanning electron, confocal
 Study of the Adhesion of Clinical Strains of Staphylococcus aureus on an Abiotic Surface Using the Biofilm Ring Test® 553 MS S A s t ra in s 5668/S 1620/P 1532/P 5741/S 0 2 4 6 8 10 Control EGTA 1 mM (a) Str a ins Log CF U/ mL (a) MRSA stra i n s 011/LP007/U027/FV 028/FV 0 2 4 6 8 10 Control EGTA 1 mM ** (b) Str a in s Log CFU/ mL (b) Figure 6. Adhesion of the clinical strains of S. aureus to a catheter. The 4 MSSA strains (a) and the 4 MRSA strains (b) were incubated for 18 h in the presence of a piece of catheter. After washing, the bacteria were detached by sonication and the number of colony forming units was estimated. Results are the means ± s.e.m. of 4 measurements. *: P < 0.05. laser-scanning, epifluorescence, atomic force microscopy) [28-31]. These methods are mostly qualitative and de- scriptive, but they do not directly measure the adhesion of bacterial populations [32]. The enumeration of bacte- ria on plate counts after the removal of biofilms or biofilm-associated bacteria by mechanical forces such as vortexing or sonication is also widely used [33,34]. This method gives indication only on the number of bacteria inside the biofilm, and not on the number of bacteria ad- hering on a surface. This slow and fastidious method is also highly dependent on cell recovery which is difficult to estimate and is not fitted to large scale screening ex- periments. Microtiter plate methods have been also de- veloped. Culture of the bacteria in the wells of the mi- croplate followed by the estimation of the biomass re- maining in the well after washing using the CVSM [35] is a very convenient and widespread method to evaluate biofilm adhesion. It has been modified to increase its accuracy and to improve its reproducibility [13,14]. As shown in this study, this method has a low sensitivity and requires rather long incubation times to get significant increase of the absorbance. The washing steps, used to remove non-adherent cells, are also critical. The lack of standardization of these washing steps makes difficult the comparison of results obtained by different laboratories [15]. The BFRT® is a newly described method for study- ing biofilm formation. This technique is based on the immobilization of magnetic beads by adherent cells [15, 17]. In this paper, we presented evidence that this method could detect adhesion of S. aureus to the bottom of the wells within a few hours, before any significant biofilm could be detected with the CVSM. These observations confirmed our previous results on S. aureus [18] or P. aeruginosa [17]. The BFRT® could also demonstrate that MSSA strains interacted more rapidly with the surface than MRSA strains. The adhesion of the MRSA strains was partly reverted by the presence in the culture me- dium of EGTA, while the adhesion of the MSSA strains was not affected by the metal-chelator. These results were confirmed with the CVSM which illustrated that, at later times, the MSSA strains formed a more important biofilm than MRSA strains. Our results were in agree- ment with those of O’Neill et al. [36]. These authors re- ported that MRSA strains were less likely to form a biofilm than MSSA strains and that their sensitivity to salts differed. They concluded that the mechanism in- volved in the formation of the biofilm was different among MSSA and MRSA strains. Another explanation for this difference between MSSA and MRSA strains could be their distinct membrane properties. The MATS test demonstrated that the two populations diverged: the membranes of the MSSA strains were more hydrophobic and had a higher propensity to donate electrons. These properties should affect the adherence of the bacteria [8]. Cations (calcium, magnesium, manganese) interfered with the behavior of the beads in the BFRT® and de- creased the BFI. Similar artefactual interactions have also been described with pronase [37] or with culture media with high ionic strength [38]. Such interaction has also been observed with antimicrobial cationic peptides derived from cathelicidin and which, at neutral pH, have many positive charges (C. Nagant, personal communica- tion). These interactions might thus be a consequence of some electrostatic interactions between the negatively charged beads and the cations or between some compo- nents of the medium. These results also illustrate the fact that the mobility of the beads can be affected not only by adhering bacteria but also by modifications of rheologi- cal properties of the medium. The interaction of the cations with EGTA was thus tested with the CVSM. The Copyright © 2012 SciRes. JBNB
 Study of the Adhesion of Clinical Strains of Staphylococcus aureus on an Abiotic Surface Using the Biofilm Ring Test® 554 ions had no effect on the formation of the biofilm by the MRSA strains but the 3 cations reversed the inhibition by EGTA. This lack of specificity suggested that the effect of the ions was indirect. Considering the dissociation constant of EGTA for the 3 cations [39], the concentra- tion of free EGTA should be very low (in the micromolar range) in solutions containing 1 mm EGTA and 1 mM calcium, magnesium or manganese. The most likely ex- planation is thus that, in the absence of any added diva- lent cation, EGTA probably binds another endogenous cation (iron [40] or zinc [41]) contributing to the forma- tion of the biofilm. It has been recently reported that zinc contributes to the rod-like structure of SasG, a protein involved in the formation of a biofilm by S. aureus [42]. The results obtained in the wells of a microplate were, at least, partly confirmed by measuring the adhesion of the bacteria on catheter tubing. In agreement with the results of the BFRT and the CVSM, the MRSA strains adhered less than the MSSA strains. The 2 MRSA strains with less adherence on the tubing in the presence of EGTA were also the 2 strains mostly affected by EGTA in the BFRT®. The conclusions of the studies comparing the adhesion of MSSA and MRSA strains were not con- sistent. Amaral et al. [43] reported that the adhesion of clinical MRSA strains on bronchial epithelial cells was higher than the adhesion of clinical MSSA strains. More recently, Pozzi et al. [44] showed that the expression of a gene responsible with resistance to methicillin was asso- ciated with increased adhesion on a catheter [44]. At the opposite, Aathitan et al. [45] reported that MRSA and MSSA strains similarly interacted with liver epithelial cells [45], whereas Karauzum et al. [46] observed that MRSA strains were less adherent on human airway epithelial cells than MSSA strains [46]. There is thus a large panel of opinions on the ability of the two popula- tions of strains to adhere on a surface. In conclusion, the formation of a biofilm by MSSA and MRSA strains proceeds at a different rate and is dif- ferently affected by cations. This should help in the for- mulation of washing solutions to clean material con- taminated by MRSA-carriers. The BFRT® could contrib.- ute to the search for these new cleaning solutions: it is a very easy and efficient technique to study the initial steps of the formation of a biofilm by S. aureus, at a time when the growth of the bacterial population only marginally affects the results. However, the results obtained with the BFRT are also consistent with the investigation on catheters. Further studies should also look for a correla- tion between the BFRT® and the adhesion of S. aureus on biotic surfaces like wounds. It should also be kept in mind that the results of an assay might be indirectly af- fected by modifications of the properties of the medium provoked by the tested drugs. 5. Acknowledgements This work was supported by grant No 3.4577.10 of the Fonds National de la Recherche Scientifique of Belgium and by a grant from the Coopération Technique Belge (CTB). J.-M. Liesse Iyamba is a recipient of a Ph.D. Re- search Fellowship from the CTB. REFERENCES [1] N. K. Archer, M. J. Mazaitis, J. W. Costerton, J. G. Leid, M. E. Powers and M. E. Shirtliff, “Staphylococcus aureus Biofilms: Properties, Regulation, and Roles in Human Disease,” Virulence, Vol. 2, No. 5, 2011, pp. 445-459. doi:10.4161/viru.2.5.17724 [2] L. Hall-Stoodley, P. Stoodley, S. Kathju, N. Høiby, C. Moser, J. W. Costerton, A. Moter and T. Bjarnsholt, “To- wards Diagnostic Guidelines for Biofilm-Associated In- fections,” FEMS Immunology and Medical Microbiology, Vol. 65, No. 2, 2012, pp. 127-145. doi:10.1111/j.1574-695X. 2012.00968 [3] D. G. Cvitkovitch, Y. H. Li and R. P. Ellen, “Quorum Sensing and Biofilm Formation in Streptococcal Infec- tions,” Journal of Clinical Investigation, Vol. 112, No. 11, 2003, pp. 1626-1632. doi:10.1172/JCI200320430 [4] M. Leone and L. R. Dillon, “Catheter Outcomes in Home Infusion,” Journal of Infusion Nursing, Vol. 31, No. 2, 2008, pp. 84-91. doi:10.1097/01.NAN.0000313655. 65410.4e [5] P. S. Stewart and J. W. Costerton, “Antibiotic Resistance of Bacteria in Biofilms,” The Lancet, Vol. 358, No. 9276, 2001, pp. 135-138. doi:10.1016/S0140-6736(01)05321-1 [6] L. Hall-Stoodley and P. Stoodley, “Evolving Concepts in Biofilm Infections,” Cellular Microbiology, Vol. 11, No. 7, 2009, pp.1034-1043. doi:10.1111/j.1462-5822.2009. 01323 [7] M. Otto, “Staphylococcal Biofilms,” Current Topics in Microbiology and Immunology, Vol. 322, 2008, pp. 207- 228. doi:10.1007/978-3-540-75418-3_10 [8] C. von Eiff, B. Jansen, W. Kohnen and K. Becker, “Infec- tions Associated with Medical Devices: Pathogenesis, Management and Prophylaxis,” Drugs, Vol. 65, No. 2, 2005, pp. 179-214. doi:10.2165/00003495- 200565020-00003 [9] L. D. Renner and D. B. Weibel, “Physicochemical Re- gulation of Biofilm Formation,” MRS Bulletin, Vol. 36, No. 5, 2011, pp. 347-355. doi:10.1557/mrs.2011.65 [10] D. López, H. Vlamakis and R. Kolter, “Biofilms,” Cold Spring Harbor Perspectives in Biology, Vol. 2, No. 7, 2010, Article ID: a000398. [11] T. R. Garrett, M. Bhakoo and Z. Zhang, “Bacterial Ad- hesion and Biofilms on Surfaces,” Progress in Natural Sciencs, Vol. 18, No. 9, 2008, pp.1049-1056. [12] B. R. Boles and A. R. Horswill, “Staphylococcal Biofilm Disassembly,” Trends in Microbiology, Vol. 19, No. 9, 2011, pp. 449-455. doi:10.1016/j.tim.2011.06.004 [13] E. Peeters, H. J. Nelis and T. Coenye “Comparison of Copyright © 2012 SciRes. JBNB
 Study of the Adhesion of Clinical Strains of Staphylococcus aureus on an Abiotic Surface Using the Biofilm Ring Test® 555 Multiple Methods for Quantification of Biofilms Grown in Microtiter Plates,” Journal of Microbiological Methods, Vol. 72, No. 2, 2008, pp. 157-165. doi.10.1016/j.mimet. 2007.11.010 [14] S. Stepanovic, D. Vukovic, I. Dakic, B. Savic and M. Svabic-Vlahovic, “A Modified Microtiter-Plate Test for Quantification of Staphylococcal Biofilm Formation,” Journal of Microbiological Methods, Vol. 40, No. 2, 2000, pp. 175-179. [15] P. Chavant, B. Gaillard-Martinie, R. Talon, M. Hébraud and T. Bernardi, “A New Device for Rapid Evaluation of Biofilm Formation Potential by Bacteria,” Journal of Microbiological Methods, Vol. 68, No. 3, 2007, pp. 605- 612. doi:10.1016/j.mimet.2006.11.010 [16] S. Sulaeman, G. Le Bihan, A. Rossero, M. Federighi, E. Dé and O. Tresse, “Comparison between the Biofilm Ini- tiation of Campylobacter Jejuni and Campylobacter Coli Strains to an Inert Surface Using BioFilm Ring Test,” Journal of Applied Microbiology, Vol. 108, No. 4, 2010, pp. 1303-1312. doi :10.1111/j.1365-2672. 2009.04534l [17] C. Nagant, M. Tré-Hardy, M. Devleeschouwer and J. P. Dehaye, “Study of the Initial Phase of Biofilm Formation Using a Biofomic Approach,” Journal of Microbiological Methods, Vol. 82, No. 3, 2010, 243-248. doi:10.1016/j. mimet.2010.06.011 [18] J. M. Liesse Iyamba, M. Seil, M. Devleeschouwer, N. B. Takaisi Kikuni and J. P. Dehaye, “Study of the Formation of a Biofilm by Clinical Strains of Staphylococcus aureus,” Biofouling, Vol. 27, No. 8, 2011, pp. 811-821. doi:10.1080/08927014.2011.604776 [19] M.-N. Bellon-Fontaine, J. Rault and C. J. Van Oss, “Mi- crobial Adhesion to Solvents: A Novel Method to Deter- mine the Electron-Donor/Electron-Acceptor or Lewis Acid-Base Properties of Microbial Cells,” Colloids and Surfaces B: Biointerfaces, Vol. 71, No. 1-2, 1996, pp. 147- 153. [20] N. Ozerdem Akpolat, S. Elçi, S. Atmaca, H. Akbayin and K. Gül, “The Effects of Magnesium, Calcium and EDTA on Slime Production by Staphylococcus Epidermidis Strains,” Folia Microbiologica (Praha), Vol. 48, No. 5, 2003, pp. 649-653. doi:10.1007/BF02993473 [21] D. E. Caldwell and J. R. Lawrence, “Study of Attached Cells in Continuous-Flow Slide Culture,” In: J. W. T. Wimpenny, Ed., Handbook of Laboratory Model Systems for Microbial Ecosystems, CRC Press, Boca Raton, 1988, pp. 117-138. [22] J. R. Lawrence, D. R. Korber, B. D. Hoyle, J. W. Cos- terton and D. E. Caldwell, “Optical Sectioning of Micro- bial Biofilms,” Journal of Bacteriology, Vol. 173, No. 20, 1991, pp. 6558-6567. [23] A. Escher and W. G. Characklis, “Modeling the Initial Events in Biofilm Accumulation,” In: W. G. Characklis and K. C. Marshall, Eds., Biofilms, John Wiley and Sons, New York, 1990, pp. 445-486. [24] H. W. Fowler, “Microbial Adhesion to Surfaces,” In: J. V. T. Wimpenny, Ed., Handbook of Laboratory Model Sys- tems for Microbial Ecosystems, CRC Press, Boca Raton, 1988, pp. 139-153. [25] M. Vorachit, K. Lam, P. Jayanetra and J. W. Costerton, “Resistance of Pseudomonas Pseudomallei Growing as a Biofilm on Silastic Disks to Ceftazidime and Cotrimox- azole,” Antimicrobial Agents and Chemotherapy, Vol. 37, No. 9, 1993, pp. 2000-2002. doi:/10.1128/AAC.37.9.2000 [26] R. Murga, J. M. Miller and R. M. Donlan, “Biofilm For- mation by Gram-Negative Bacteria on Central Venous Catheter Connectors: Effect of Conditioning Films in a Laboratory Model,” Journal of Clinical Microbiology, Vol. 39, No. 6, 2001, pp. 2294-2297. doi:10.1128/JCM. 39.6.2294-2297.2001 [27] C. Nagant, B. Pitts, N. Kamran, M. Vandenbranden, J. G. Bolscher, P. S. Stewart and J. P. Dehaye, “Identification of the Domains of the Human Antimicrobial Peptide LL-37 Active against the Biofilms Formed by Pseudo- monas Aeruginosa Using a Library of Truncated Frag- ments,” Unpublished. [28] S. B. Surman, J. T. Walker, D. T. Goddard, L. H. G. Morton, C. W. Keevil, W. Weaver, A. Skinner, A. Han- son, D. Caldwell and J. Kurtz, “Comparison of Micro- scope Techniques Examination of Biofilms,” Journal of Microbiological Methods, Vol. 25, No. 1, 1996, pp. 57-70. doi:10.1016/0167-7012(95)00085-2 [29] A. Sénéchal, S. D. Carrigan and M. Tabrizian, “Probing Surface Adhesion Forces of Enterococcus Faecalis to Medical-Grade Polymers Using Atomic Force Micro- scopy,” Langmuir, Vol. 20, No. 10, 2004, pp. 4172-4177. doi:10.1021/la035847y [30] S. L. Burnett, J. Chen and L. R. Beuchat, “Attachment of Escherichia coli O157:H7 to the Surfaces and Internal Structures of Apples as Detected by Confocal Scanning Laser Microscopy,” Applied and Environmental Micro- biology, Vol. 66, No. 11, 2000, pp. 4679-4687. doi:/10.1128/AEM.66.11.4679-4687.2000 [31] L. Kodjikian, C. Burillon, G. Lina, C. Roques, G. Pellon, J. Freney and F. N. Renaud, “Biofilm Formation on In- traocular Lenses by a Clinical Strain Encoding the Ica Locus: A Scanning Electron Microscopy Study,” Inves- tigative Ophthalmology and Visual Science, Vol. 44, No. 10, 2003, pp. 4382-4387. doi:10.1167/iovs.03-0185 [32] J. B. Xavier, D. C. White and J. S. Almeida, “Automated Biofilm Morphology Quantification from Confocal Laser Scanning Microscopy Imaging,” Water Scie nc e an d Te c h- nology, Vol. 47, No. 5, 2003, pp. 31-37. [33] H. Anwar, J. L. Strap and J. W. Costerton, “Eradication of Biofilm Cells of Staphylococcus aureus with Tobra- mycin and Cephalexin,” Canadian Journal of Microbiol- ogy, Vol. 38, No. 7, 1992, pp. 618-625. doi: 10.1139/m92-102 [34] N. Oulahal, A. Martial-Gros, M. Bonneau and L. J. Blum, “Combined Effect of Chelating Agents and Ultrasound on Biofilm Removal from Stainless Steel Surfaces. Applica- tion to Escherichia coli Milk and Staphylococcus aureus Milk Biofilms,” Biofilms, Vol. 1, No. 1, 2004, pp. 65-73. doi:10.1017/S1479050504001140 [35] G. D. Christensen, W. A. Simpson, J. J. Younger, L. M. Baddour, F. F. Barrett, D. M. Melton and E. H. Beachey, Copyright © 2012 SciRes. JBNB
 Study of the Adhesion of Clinical Strains of Staphylococcus aureus on an Abiotic Surface Using the Biofilm Ring Test® Copyright © 2012 SciRes. JBNB 556 “Adherence of Coagulase-Negative Staphylococci to Plas- tic Tissue Culture Plates: A Quantitative Model for the Adherence of Staphylococci to Medical Devices,” Jour- nal of Clinical Microbiology, Vol. 22, No. 6, 1985, pp. 996-1006. [36] E. O’Neill, C. Pozzi, P. Houston, D. Smyth, H. Hum- phreys, D. A. Robinson and J. P. O’Gara, “Association between Methicillin Susceptibility and Biofilm Regula- tion in Staphylococcus aureus Isolates from Device-Re- lated Infections,” Journal of Clinical Microbiology, Vol. 45, No. 5, 2007, pp. 1379-1388. doi: 10.1128/JCM.02280-06 [37] S. Badel, C. Laroche, C. Gardarin, T. Bernardi and P. Michaud, “New Method Showing the Influence of Matrix Components in Leuconostoc Mesenteroides Biofilm For- mation,” Applied Biochemistry Biotechnology, Vol. 151, No. 2-3, 2008, pp. 364-370. doi:10.1007/ s12010-008-8199-y [38] S. Badel, F. Callet, C. Laroche, C. Gardarin, E. Petit, H. El Alaoui, T. Bernardi and P. Michaud, “A New Tool to Detect High Viscous Exopolymers from Microalgae,” Journal of Industrial Microbiology and Biotechnology Vol. 32, No. 2, 2011, pp. 319-326. doi:10.1007/ s10295-010-0775-9 [39] G. L. Smith and D. J. Miller, “Potentiometric Measure- ments of Stoichiometric and Apparent Affinity Constants of EGTA for Protons and Divalent Ions Including Cal- cium,” Biochimica et Biophysica Acta, Vol. 839, No. 3, 1985, pp. 287-299. doi:10.1016/ 0304-4165(85)90011-X [40] M.-H. Lin, J.-C. Shu, H.-Y. Huang and Y.-C. Cheng, “Involvement of Iron in Biofilm Formation by Staphylo - coccus aureus,” PLOS One, Vol. 7, No. 3, 2012, Article ID: e34388. doi:10.1371/journal.pone.0034388 [41] D. G. Conrady, C. C. Brescia, K. Horii, A. A. Weiss, D. J. Hassett and A. B. Herr, “A Zinc-Dependent Adhesion Module Is Responsible for Intercellular Adhesion in Sta- phylococcal Biofilms,” Proceedings of the National Aca- demy of Sciences USA, Vol. 105, No. 49, 2008, pp. 19456-19461. doi:10.1073/pnas.0807717105 [42] D. T. Gruszka, J. A. Wojdyla, R. J. Bingham, J. P. Turk- enburg, I. W. Manfield, A. Steward, A. P. Leech, J. A. Geoghegan, T. J. Foster, J. Clarke and J. R. Potts, “Staphy- lococcal Biofilm-Forming Protein Has a Contiguous Rod-Like Structure,” Proceedings of the National Acad- emy of Sciences USA, Vol. 109, No. 17, 2012, pp. E1011- E1018. doi:10.1073/pnas.1119456109 [43] M. M. Amaral, L. R. Coelho, R. P. Flores, R. R. Souza, M. C. Silva-Carvalho, L. A. Teixeira, B. T. Ferreira-Car- valho and A. M. S. Figueiredo, “The Predominant Variant of the Brazilian Epidemic Clonal Complex of Methicil- lin-Resistant Staphylococcus aureus Has an Enhanced Ability to Produce Biofilm and to Adhere to and Invade Airway Epithelial Cells,” Journal of Infectious Diseases, Vol. 192, No. 5, 2005, pp. 801-810. doi:10.1086/432515 [44] C. Pozzi, E. M. Waters, J. K. Rudkin, C. R. Schaeffer, A. J. Lohan, P. Tong, B. J. Loftus, G. B. Pier, P. D. Fey, R. C. Massey and J. P. O’Gara, “Methicillin-Resistance Al- ters the Biofilm Phenotype and Attenuates Virulence in Staphylococcus aureus Device-Associated Infections,” PLOS Pathogy, Vol. 8, No. 4, 2012, Article ID: e1002626. [45] S. Aathithan, R. Dybowski and G. L. French, “Highly Epidemic Strains of Methicillin-Resistant Staphylococcus aureus Not Distinguished by Capsule Formation, Protein a Content or Adherence to HEP-2 Cells,” European Jour- nal of Clinical Microbiology & Infectious Diseases, Vol. 20, No. 1, 2001, pp. 27-32. doi:10.1007/PL00011233 [46] H. Karauzum, T. Ferry, S. de Bentzmann, G. Lina, M. Bes, F. Vandenesch, M. Schmaler, B. Berger-Bächi, J. Etienne and R. Landmann, “Comparison of Adhesion and Virulence of Two Predominant Hospital-Acquired Methi- cillin-Resistant Staphylococcus aureus Clones and Clonal Methicillin-Susceptible Staphylococcus aureus Isolates,” Infection and Immunity, Vol. 76, No. 11, 2008, pp. 5133- 5138. doi:10.1128/IAI.01697-07.
|