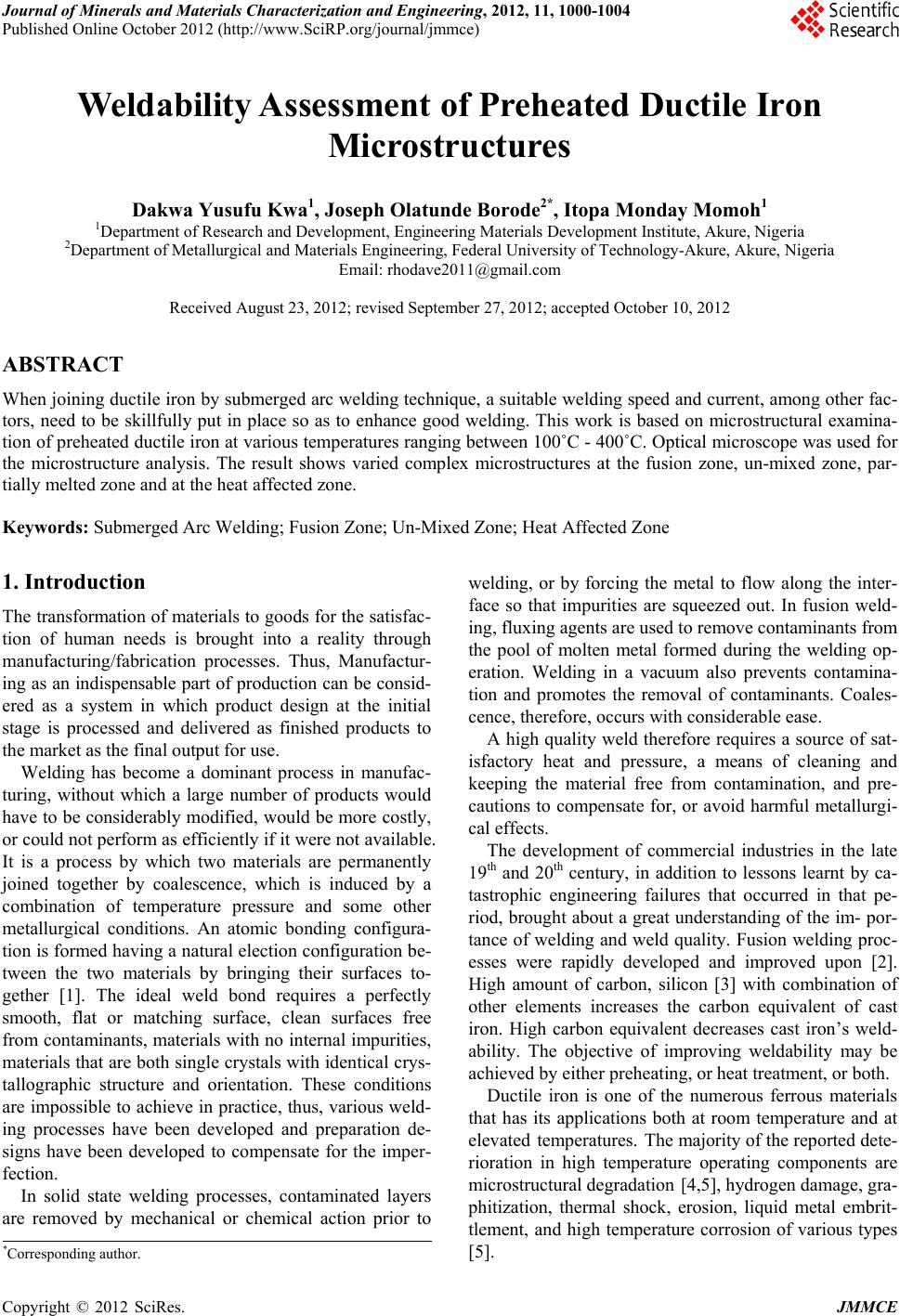
Journal of Minerals and Materials Characterization and Engineering, 2012, 11, 1000-1004
Published Online October 2012 (http://www.SciRP.org/journal/jmmce)
Weldability Assessment of Preheated Ductile Iron
Microstructures
Dakwa Yusufu Kwa1, Joseph Olatunde Borode2*, Itopa Monday Momoh1
1Department of Research and Development, Engineering Materials Development Institute, Akure, Nigeria
2Department of Metallurgical and Materials Engineering, Federal University of Technology-Akure, Akure, Nigeria
Email: rhodave2011@gmail.com
Received August 23, 2012; revised September 27, 2012; accepted October 10, 2012
ABSTRACT
When joining ductile iron by submerged arc welding technique, a suitable welding speed and current, among other fac-
tors, need to be skillfully put in place so as to enhance good welding. This work is based on microstructural examina-
tion of pr eheated ductile iron at v arious temperatures ran ging between 100˚C - 400 ˚C. Optical microscope was used for
the microstructure analysis. The result shows varied complex microstructures at the fusion zone, un-mixed zone, par-
tially melted zone and at the heat affected zone.
Keywords: Submerged Arc Welding; Fusion Zone; Un-Mixed Zone; Heat Affected Zone
1. Introduction
The transformation of materials to goods for the satisfac-
tion of human needs is brought into a reality through
manufacturing/fabrication processes. Thus, Manufactur-
ing as an indisp ensable part of production can be consid -
ered as a system in which product design at the initial
stage is processed and delivered as finished products to
the market as the final output fo r u se .
Welding has become a dominant process in manufac-
turing, without which a large number of products would
have to be considerably modified, would be more costly,
or could not perform as efficiently if it were not available.
It is a process by which two materials are permanently
joined together by coalescence, which is induced by a
combination of temperature pressure and some other
metallurgical conditions. An atomic bonding configura-
tion is formed having a natural election configuration be-
tween the two materials by bringing their surfaces to-
gether [1]. The ideal weld bond requires a perfectly
smooth, flat or matching surface, clean surfaces free
from contaminants, materials with no internal impurities,
materials that are both single crystals with identical crys-
tallographic structure and orientation. These conditions
are impossible to achieve in practice, thus, various weld-
ing processes have been developed and preparation de-
signs have been developed to compensate for the imper-
fection.
In solid state welding processes, contaminated layers
are removed by mechanical or chemical action prior to
welding, or by forcing the metal to flow along the inter-
face so that impurities are squeezed out. In fusion weld-
ing, fluxing agents are used to remove contaminants from
the pool of molten metal formed during the welding op-
eration. Welding in a vacuum also prevents contamina-
tion and promotes the removal of contaminants. Coales-
cence, therefore, occurs with considerable ease.
A high quality weld therefore requires a source of sat-
isfactory heat and pressure, a means of cleaning and
keeping the material free from contamination, and pre-
cautions to compensate for, or avoid harmful metallurgi-
cal effects.
The development of commercial industries in the late
19th and 20th century, in addition to lessons learnt by ca-
tastrophic engineering failures that occurred in that pe-
riod, brought abou t a great understanding of the im- por-
tance of welding and weld quality. Fusion welding proc-
esses were rapidly developed and improved upon [2].
High amount of carbon, silicon [3] with combination of
other elements increases the carbon equivalent of cast
iron. High carbon equivalent decreases cast iron’s weld-
ability. The objective of improving weldability may be
achieved by either preheating, or heat treatment, or both.
Ductile iron is one of the numerous ferrous materials
that has its applications both at room temperature and at
elevated temperatures. The majority of the reported dete-
rioration in high temperature operating components are
microstructural degradation [4,5], hydrogen damage, gra-
phitization, thermal shock, erosion, liquid metal embrit-
tlement, and high temperature corrosion of various types
[5].
*Corresponding author.
Copyright © 2012 SciRes. JMMCE