 Open Journal of Geology, 2012, 2, 229-240 http://dx.doi.org/10.4236/ojg.2012.24023 Published Online October 2012 (http://www.SciRP.org/journal/ojg) U-Pb Zircon Age, Geochemical and Sr-Nd Isotopic Constraints on the Age and Origin of the Granodiorites in Guilong, Southeastern Yunnan Province, Southern China Shen Liu1, Ruizhong Hu1, Caixia Feng1, Shan Gao2, Guangying Feng1, Youqiang Qi1, Tao Wang3, Ian M. Coulson4, Yuhong Yang1, Chaogui Yang1 1State Key Laboratory of Ore Deposit Geochemistry, Institute of Geochemistry, Chinese Academy of Sciences, Guiyang, China 2State Key Laboratory of Geological Processes and Mineral Resources, China University of Geosciences, Wuhan, China 3Chengdu University of Technology, Chengdu, China 4Solid Earth Studies Laboratory, Department of Geology, University of Regina, Regina, Canada Email: liushen@vip.gyig.ac.cn Received July 9, 2012; revised August 6, 2012; accepted September 7, 2012 ABSTRACT Post-collision felsic rocks in Southeastern Yunnan province contain granodiorites. U-Pb zircon ages, geochemical data and Sr-Nd isotopic data for these rocks are reported in the present paper. Laser ablation inductively coupled plasma mass spectrometry U-Pb zircon analyses yielded consistent age 252.5 ± 1.0 Ma for one sample of the felsic rocks. The granodiorites were characterized by variational and high (87Sr/86Sr)i, ranging from 0.7223 to 0.7236 and very low εNd (t) values from –29.1 to –30.4. In addition, these rocks are characterized by slight Eu negative anomalies, Nb, Ta, Ti and Sr negative anomalies on primitive mantle normalization spider. Geochemical and isotopic characteristics suggest that these rocks were derived from an enriched crust source. The granodiorites resulted from the fractionation of potassium feldspar, plagioclase and ilmenite or rutile. However, the granodiorites were unaffected by visible crustal contamination during ascent. As a result, the granodiorites may have been formed due to partial melting of crust-derived sedimentary rocks beneath southeastern Yunnan province, southern China. Keywords: Granodiorites; Age; Origin; Southeastern Yunnan Province; Southern China 1. Introduction Felsic rocks (e.g., granite, granodiorite, etc.) are widely distributed in Honghe polymetallic deposits (super-large Sn, Cu, Pb, Zn, Sb, Ag, Mo, Au and Bi deposits) [1-6] and Bainiuchang super-large Ag-Pb-Zn polymetallic de- posits [7-14]. These rocks, especially granite and grano- diorite, can be used to study the mineralization and met- allogenesis of polymetallic deposits in southeastern Yun- nan province, Southern China. Although a number of studies about deposits have been carried out, recent analytical techniques and sys- tematic geochemical studies (e.g., ages, geochemical data and isotopic data) on granites and granodiorites are li- mited. Therefore, we provide systematic geochemical data and LA-ICP-MS zircon U-Pb and Sr-Nd data for the granodiorites to constrain age, source, fractionation and genetic model of the studied felsic rocks. 2. Geological Setting and Petrography Many types of Mesozoic-Cenozoic granites and acidic porphyries are present in southeastern Yunnan province. Each felsic rock may provide important insights into the tectonothermal evolution of the Mesozoic-Cenozoic litho- sphere of Yunnan province and the possible linkage(s) between Yunnan and other places (i.e., terrene, craton, etc.). Limited precise ages for the felsic rocks in Yunnan province have been published in recent papers. The study area is located within Guilong area, Luchun County, Yunnan province, southeastern China (Figure 1). Granodiorites in Guilong are emplaced into Trias sedi- mentary rocks (T3g) (e.g., sandstone and shale) and gran- ite without precise age. Some orthoclase and mafic dykes (x, lamprophyres) are present in the southern mar- gin of the granodiorites. The granodiorites are commonly ~0.9 km wide and ~1.7 km long. They are exposed for ca. 1.6 km2. The ages of these rocks remain unknown. Fig- ure 2 shows the representative photomicrographs of the granodiorites from Guilong. All granodiorites are por- phyry with typical porphyritic texture and massive struc- ture (Figure 2). The granodiorites mainly contain 40% to 45% plagioclase, 16% to 18% potash feldspar (K-feld- C opyright © 2012 SciRes. OJG
 S. LIU ET AL. 230 (b) Figure 1. (a) Simplified tectonic map of the study area, Yunnan Province, China; (b) Map of China and distributions of the fault. Figure 2. Repressive photos of granodior ites in Guilong, Southeastern Yunnan Provinc e. spar), 20% to 25% quartz, 5.0% to 8.0% biotite, 2.0% to 5.0% hornblende and minor (<2.0%) accessory mi- nerals, such as apatite, titanite, zircon, magnetite, alla- nite, etc. 3. Analytical Procedures 3.1. U-Pb Dating by LA-ICP-MS Method Zircon was separated from one sample (GL01) using conventional heavy liquid and magnetic techniques at the Langfang Regional Geological Survey, Hebei Province, China. Zircon separates were examined under transmitted and reflected light and by cathodoluminescence petrog- raphy at the State Key Laboratory of Continental Dy- namics, Northwest University, China, to observe their external and internal structures. Laser-ablation techniques were employed for zircon age determinations (Table 1; Figure 3) using an Agilent 7500a ICP-MS instrument equipped with a 193 nm ex- cimer laser at the State Key Laboratory of Geological Processes and Mineral Resources, China University of Geoscience, Wuhan, China. Zircon #91500 was used as standard and NIST 610 was used to optimize the results. A spot diameter of 24 μm was used. Prior to LA-ICP-MS Copyright © 2012 SciRes. OJG
 S. LIU ET AL. 231 Table 1. LA-ICPMS U-Pb isotopic data for zircons in the felsic rocks in Guilong, Yunnan Provinc e , China. Copyright © 2012 SciRes. OJG
 S. LIU ET AL. 232 Figure 3. Selected zircon CL images and the LA-ICP-MS zircon U-Pb concordia diagram for the granodiorite (GL01) in Guilong, Southeastern Yunnan Provinc e. zircon U-Pb dating, the surfaces of the grain mounts were washed in dilute HNO3 and pure alcohol to remove any potential lead contamination. The analytical method- ology has been described in detail by Yuan et al. (2004) [15]. Correction for common Pb was performed follow- ing Andersen (2002) [16]. Data were processed using the GLITTER and ISOPLOT programs [17] (Table 1; Fig- ure 3). Errors for individual analyses by LA-ICP-MS were quoted at the 95% (1σ) confidence level. 3.2. Major Elemental, Trace Elemental and Isotopic Analyses Twenty-seven samples were collected to carry out major and trace element determinations and Sr-Nd isotopic ana- lyses. Whole-rock samples were trimmed to remove al- tered surfaces, cleaned with deionized water and then crushed and powdered using an agate mill. Major elements were analyzed using PANalytical Ax- ios-advance (Axios PW4400) X-Ray Fluorescence spec- trometer (XRF) at the State Key Laboratory of Ore De- posit Geochemistry, Institute of Geochemistry, Chinese Academy of Sciences. Fused glass disks were used. Based on the Chinese National standards GSR-1 and GSR-3 (Table 2), analytical precision was better than 5%. Loss on Ignition (LOI) was obtained using 1 g of powder heated to 1100˚C for 1 h. Trace elements were analyzed by plasma optical emis- sion MS ICP-MS at the National Research Center of Geoanalysis, Chinese Academy of Geosciences follow- ing the procedures described by Qi et al. (2000) [18]. The discrepancy among triplicates was less than 5% for all elements. Analysis results of the international stan- dards OU-6 and GBPG-1 were consistent with the re- commended values (Table 3). For the analyses of Rb-Sr and Sm-Nd isotopes, sample powders were spiked with mixed isotope tracers, dis- solved in Teflon capsules with HF + HNO3 acids and separated by conventional cation-exchange techniques. Isotopic measurements were performed using a Finnigan Triton Ti thermal ionization mass spectrometer at the State Key Laboratory of Geological Processes and Mi- neral Resources, China University of Geosciences, Wu- han, China. Procedural blanks were <200 pg for Sm and Nd and <500 pg for Rb and Sr. Mass fractionation cor- rections for Sr and Nd isotopic ratios were based on 86Sr/88Sr = 0.1194 and 146Nd/144Nd = 0.7219, respectively. Analyses of standards yielded the following results: NBS987 gave 87Sr/86Sr = 0.710246 ± 16 (2σ) and La Jolla gave 143Nd/144Nd = 0.511863 ± 8 (2σ). The analytic- cal results for Sr-Nd isotopes are presented in Table 4. 4. Results 4.1. Zircon U-Pb Age Euhedral zircon grains in samples GL01 are clean and prismatic, with magmatic oscillatory zoning. A total of 18 grains have a weighted mean 206Pb/238U age of 252.5 ± 1.0 Ma (1σ) (95% confidence interval) for GL01 (Ta- Copyright © 2012 SciRes. OJG
 S. LIU ET AL. 233 ble 1; Figure 3). These determinations are the best esti- mates of the crystallization ages of the granodiorites. Some inherited zircons (1421 and 8 80 Ma; Table 1 ) are present in the rock. 4.2. Major and Trace Elements Geochemical data on the granodiorites in the study area are listed in Tables 2 and 3. The granodiorites have a relatively wide range of chemical compositions, with SiO2 = 65.73 wt% to 69.94 wt%, Al2O3 = 13.04 wt% to 14.11wt%, MgO = 1.41 wt% to 1.90 wt% (Mg# = 40 to 46), Fe2O3 = 4.67 wt% to 5.59 wt%, CaO = 0.72 wt% to 2.72 wt%, K2O = 3.71 wt% to 4.98 wt% and Na2O = 2.45 wt% to 4.03 wt%. They have consistent TiO2 = 0.67 wt% to 0.81 wt%, MnO = 0.06 wt% to 0.08 wt% and P2O5 = 0.14 wt% to 0.16 wt%. Table 2. Major oxides (w t%) for the felsic rocks in Guilong, Yunnan Province, China. Sample Rock type SiO2TiO2 Al2O3 Fe2O3MnO CaO MgOK2ONa 2OP 2O5 LOI Total Mg#TZr (˚C) GL-1 granodiorite 66.91 0.71 13.04 4.750.082.721.594.442.570.15 2.36 99.32 42 840 GL-2 granodiorite 67.470.77 14.06 5.210.070.741.724.124.030.16 1.76 100.11 42 844 GL-3 granodiorite 68.940.71 13.34 4.670.071.001.514.483.500.15 1.81 100.17 42 846 GL-4 granodiorite 66.82 0.67 13.57 4.700.071.811.564.182.840.14 2.75 99.11 42 857 GL-5 granodiorite 66.85 0.74 13.63 5.070.081.431.714.662.540.15 2.37 99.22 43 842 GL-6 granodiorite 67.29 0.74 13.72 5.100.070.931.664.672.530.16 2.38 99.25 42 873 GL-7 granodiorite 67.82 0.81 14.07 4.960.070.991.684.812.650.16 1.86 99.87 43 886 GL-8 granodiorite 67.53 0.75 13.56 4.960.061.041.414.792.570.16 2.36 99.18 38 853 GL-9 granodiorite 68.00 0.76 13.78 5.000.080.911.694.832.520.16 2.14 99.87 43 867 GL-10 granodiorite 68.960.74 13.79 5.080.071.071.674.762.560.15 1.35 100.20 42 838 GL-11 granodiorite 65.73 0.77 14.03 5.590.080.731.904.812.580.16 2.76 99.13 43 875 GL-12 granodiorite 69.110.75 13.95 5.050.060.721.524.412.990.16 1.42 100.13 40 839 GL-13 granodiorite 68.72 0.69 13.46 4.650.071.451.564.722.590.14 1.85 99.91 42 825 GL-14 granodiorite 68.130.75 13.79 4.880.070.931.674.872.520.16 2.33 100.10 43 846 GL-15 granodiorite 67.94 0.75 13.63 4.860.081.751.584.372.830.15 1.98 99.92 42 825 GL-16 granodiorite 68.46 0.72 13.35 5.350.080.971.653.713.480.15 1.99 99.91 40 840 GL-17 granodiorite 67.54 0.74 13.64 5.070.061.051.624.792.600.16 2.31 99.58 41 845 GL-18 granodiorite 66.21 0.72 13.63 5.170.071.431.544.772.860.15 2.63 99.18 42 839 GL-19 granodiorite 68.040.76 13.94 5.2 0.07 1.19 1.62 4.90 2.45 0.16 1.75 100.07 41 851 GL-20 granodiorite 69.130.74 13.61 5.110.081.191.664.442.670.15 1.45 100.22 42 835 GL-21 granodiorite 66.88 0.73 13.86 4.780.060.931.554.972.990.16 2.37 99.28 42 845 GL-22 granodiorite 67.750.75 13.68 5.140.081.381.714.362.770.15 2.24 100.01 42 841 GL-23 granodiorite 69.940.77 13.79 5.150.070.851.604.842.700.16 0.56 100.43 41 852 GL-24 granodiorite 67.65 0.72 13.84 4.840.060.901.584.962.580.15 2.12 99.39 43 839 GL-25 granodiorite 69.110.72 13.94 4.710.071.161.524.882.690.15 1.21 100.16 46 847 GL-26 granodiorite 69.130.75 14.11 5.000.071.301.634.982.620.15 0.38 100.13 45 848 GL-27 granodiorite 68.920.75 13.92 5.540.101.161.564.712.740.16 0.57 100.13 41 857 GSR-3 RV* 44.642.37 13.83 13.40.178.817.772.323.380.95 2.24 99.88 - - GSR-3 MV* 44.752.36 14.14 13.350.168.827.742.3 3.180.97 2.12 99.89 - - GSR-1 RV* 72.830.29 13.4 2.140.061.550.425.013.130.09 0.7 99.62 - - GSR-1 MV* 72.650.29 13.52 2.180.061.560.465.033.150.11 0.69 99.70 - - Note: LOI, loss on ignition. Mg# = 100 × Mg/(Mg + Fe) atomic ratio. “-”, not caculated. TZr (˚C) is calculated from zircon saturation thermometry [33]. RV, recommended values; MV, measured values. The values for GSR-1 and GSR-3 are from Wang et al. (2003) [38]. Copyright © 2012 SciRes. OJG
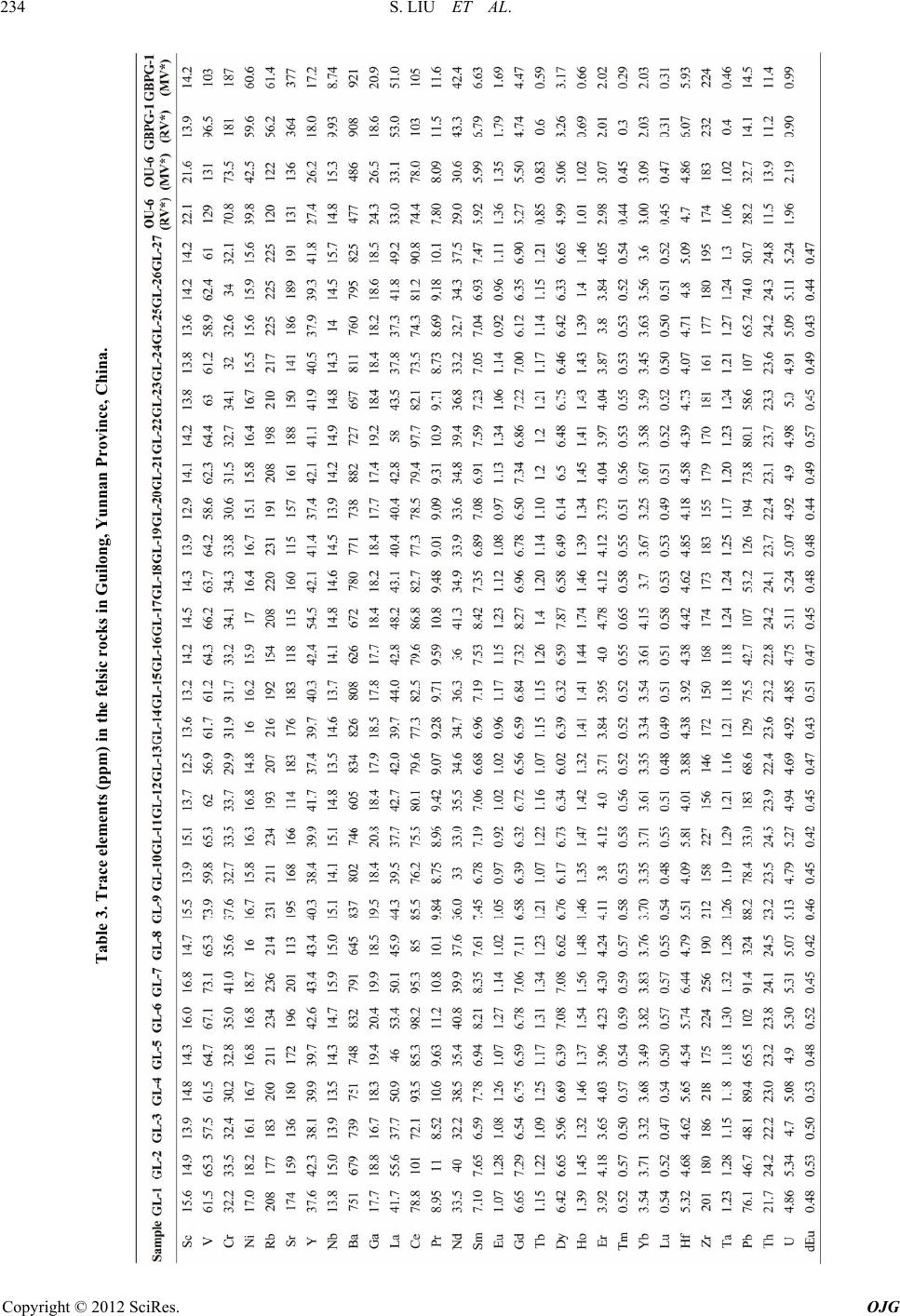 S. LIU ET AL. 234 Table 3. Trace elements (ppm) in the felsic rocks in Guilong, Yunnan Province, China. Copyright © 2012 SciRes. OJG
 S. LIU ET AL. Copyright © 2012 SciRes. OJG 235 The granodiorites are relatively high in total alkalis, with K2O + Na2O ranging from 7.02 wt% to 8.15 wt%. All granodiorites in the calc-alkaline field are plotted on the Total Alkali-Silica (TAS) diagram (Figure 4(a)). All sam- ples also straddle the shoshonitic series in the Na2O vs K2O plot (Figure 4(b)). In the plot of the molar ratios of Al2O3/(Na2O + K2O) and Al2O3/(CaO + Na2O + K2O), the rocks are mostly peraluminous, except for one sample falling the metaluminous field (Figure 4(c)). The grano- diorites display almost unchanged TiO2, Al2O3, Fe2O3, MgO, CaO, Na2O + K2O, MnO, P2O5, Rb, Cr and Ni, relatively decreasing Zr and increasing SiO2. They have no correlations among Sr, Ba and SiO2 (Figures 5 and 6). All granodiorites are characterized by Light Rare Earth Element (LREE) enrichment and Heavy Rare Earth Ele- ment (HREE) depletion, with a wide range of (La/Yb)N values (7.29 to 11.62) and slight negative Eu anomalies (Eu/Eu* = 0.42 to 0.57) (Table 3 and Figure 7(a)). In the primitive mantle-normalized trace element diagrams, the granodiorites show enrichment in Large Ion Lithophile Elements (LIL E) (i.e. , Rb, Pb and U) and depletion in Ba, Sr and High Field Strength Elements (HFSE) (i.e., Nb, Ta, P and Ti) (Figure 7(b)). 4.3. Sr-Nd and Pb Isotopes Sr-Nd isotopic data have been obtained from representa- tive granodiorite samples (Table 4). The felsic rocks show uniform (87Sr/86Sr)i values, ranging from 0.7231 to 0.7237 and relatively little variation in initial. εNd (t) va- lues from –29.1 to –30.4, suggesting an enriched source region. The Sr-Nd isotopic compositions (Figure 8) are also comparable with the upper crust. 5. Discussion 5.1. Mantle Contribution Figure 4. Classification of the granodiorites in Southeastern Yunnan province based on three diagrams. (a) TAS dia- gram. All major elemental data have been recalculated to 100% on a LOI-free basis [34-35]. (b) K2O vs Na2O dia- gram. The granodiorites are shown to be shoshonitic [36 ]. (c) Al2O3/(Na2O + K2O) molar vs Al2O3/(CaO + Na2O + K2O) molar plot. Most samples fall in the peraluminous field. However, one sample straddles the metaluminous field. Currently, the interaction between crust and mantle is very important for the genetic investigation of granitoid rocks. Previous studies suggest that mantle contribution (e.g., material and energy) during granitoid rock forma- tion cannot be ignored [19-21]. The REE of the granodiorites [REE = 181˚ ppm to 242˚ ppm, (La/Yb) N = 7.29 to 11.62, Eu = 0.42 to 0.57] has some visible differences with that of granitoid rocks formed by re-melting of the continental crust with high maturity, such as Suidong intrusion in Southern China [REE = 169˚ ppm to 268˚ ppm, (La/Yb)N = 6.44 to 10.74, Eu = 0.14 to 0.31 [22]. However, the REE can be comparable with that of syntactic-type granitic rocks involving obvious mantle material in their petrogenesis in southern China, e.g., Wuping intrusion [REE = 103˚ ppm to 395˚ ppm, (La/Yb) N = 5.3 to 38.7, Eu = 0.34 to 0.56] [23] and Longwo intrusion [REE = 103˚ ppm to 196˚ ppm, (La/Yb) N = 4.5 to 35.7, Eu = 0.41 to 0.62] [24]. The granodiorites in the present study have relatively higher compatible element contents (V = 58.6˚ ppm to 73.1˚ ppm, Cr = 29.9˚ ppm to 41.0˚ ppm, Ni = 14.8˚ ppm to 18.7˚ ppm) than some granitic rocks formed by the inter- action of crust and mantle in the Yangtze River and south- ern China (Wuping biotite monzogranite [23]; granodio- rites in Longwo [24,25]). In addition, the high Mg# (43 - 46; Table 2) of the rocks agrees with interaction of crust and mantle. Simutaneously, the Sr-Nd isotopic signatures
 S. LIU ET AL. 236 Figure 5. Selected variation diagrams of major elemental oxides vs SiO2 plots for the felsic rocks in Southeastern Yunnan Province. of the granodiorites are comparable with those in the associated mafic dykes (lamprophyres) in the study area (Figure 1). In summary, this evidence indicates that evident man- tle materials contributed to the diagenesis of Guilong granodiorites in Yunnan Province. Copyright © 2012 SciRes. OJG
 S. LIU ET AL. 237 Figure 6. Selected variation diagrams of trace elements vs SiO2 plots for the felsic rocks in Southeastern Yunnan Province. Figure 7. (a) Chondrite-normalized REE diagrams; (b) Primitive mantle-normalized trace element spidergrams for the granodiorites in Southeastern Yunnan Province. The normalization values are from Sun and McDonough (1989) [37]. 5.2. Crustal Contamination Assimilation, crystal fractionation (AFC), or magma mixing is usually postulated to explain the occurrence of comagmatic felsic rocks [26-29]. AFC and magma mix- ing result in a positive correlation between SiO2 and Nd (t) values and a negative correlation between SiO2 and Copyright © 2012 SciRes. OJG
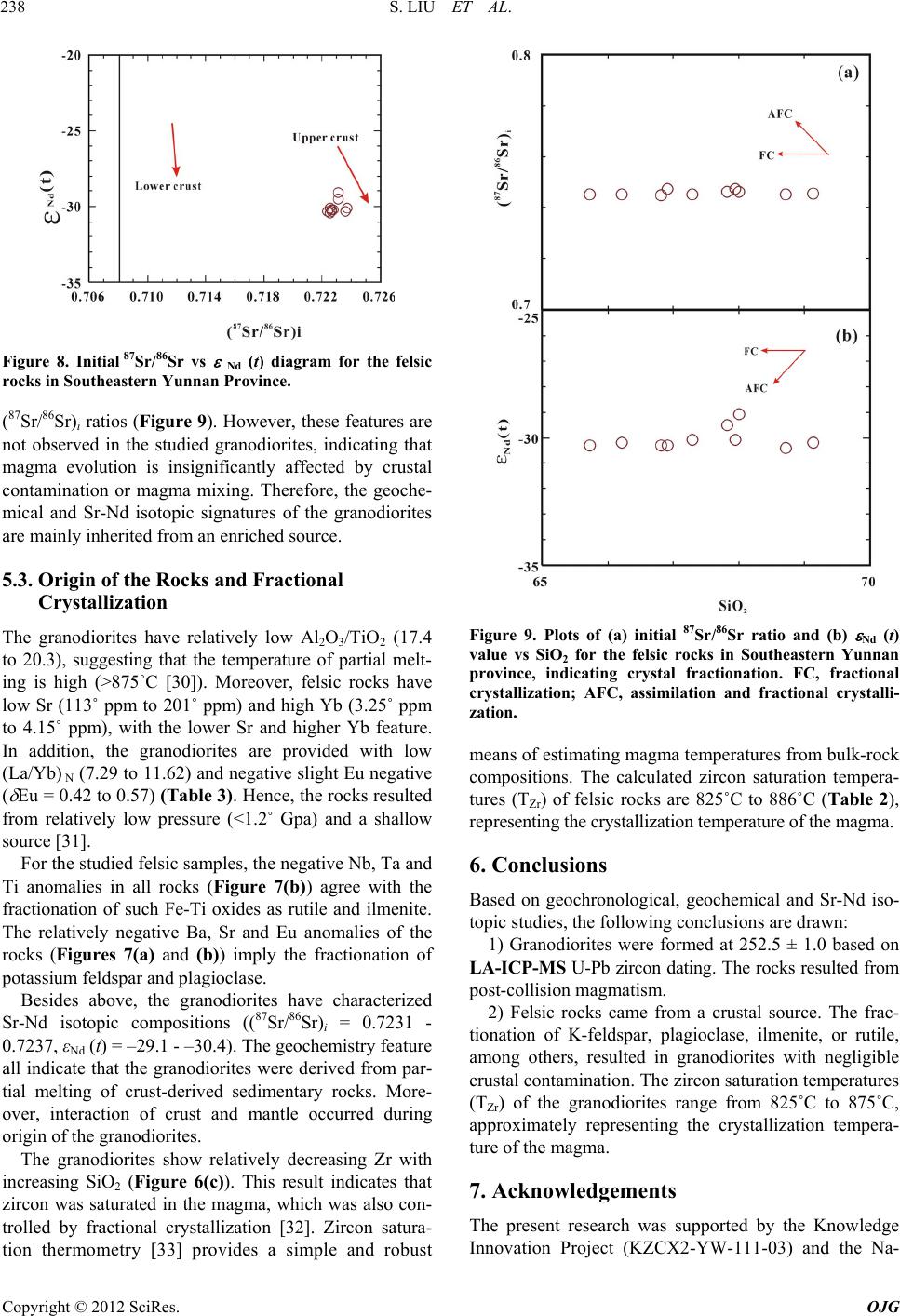 S. LIU ET AL. 238 Figure 8. Initial 87Sr/86Sr vs Nd (t) diagram for the felsic rocks in Southeastern Yunnan P rovince. (87Sr/86Sr)i ratios (Figure 9). However, these features are not observed in the studied granodiorites, indicating that magma evolution is insignificantly affected by crustal contamination or magma mixing. Therefore, the geoche- mical and Sr-Nd isotopic signatures of the granodiorites are mainly inherited from an enriched source. 5.3. Origin of the Rocks and Fractional Crystallization The granodiorites have relatively low Al2O3/TiO2 (17.4 to 20.3), suggesting that the temperature of partial melt- ing is high (>875˚C [30]). Moreover, felsic rocks have low Sr (113˚ ppm to 201˚ ppm) and high Yb (3.25˚ ppm to 4.15˚ ppm), with the lower Sr and higher Yb feature. In addition, the granodiorites are provided with low (La/Yb) N (7.29 to 11.62) and negative slight Eu negative ( Eu = 0.42 to 0.57) (Table 3). Hence, the rocks resulted from relatively low pressure (<1.2˚ Gpa) and a shallow source [31]. For the studied felsic samples, the negative Nb, Ta and Ti anomalies in all rocks (Figure 7(b)) agree with the fractionation of such Fe-Ti oxides as rutile and ilmenite. The relatively negative Ba, Sr and Eu anomalies of the rocks (Figures 7(a) and (b)) imply the fractionation of potassium feldspar and plagioclase. Besides above, the granodiorites have characterized Sr-Nd isotopic compositions ((87Sr/86Sr)i = 0.7231 - 0.7237, εNd (t) = –29.1 - –30.4). The geochemistry feature all indicate that the granodiorites were derived from par- tial melting of crust-derived sedimentary rocks. More- over, interaction of crust and mantle occurred during origin of the granodiorites. The granodiorites show relatively decreasing Zr with increasing SiO2 (Figure 6(c)). This result indicates that zircon was saturated in the magma, which was also con- trolled by fractional crystallization [32]. Zircon satura- tion thermometry [33] provides a simple and robust Figure 9. Plots of (a) initial 87Sr/86Sr ratio and (b) Nd (t) value vs SiO2 for the felsic rocks in Southeastern Yunnan province, indicating crystal fractionation. FC, fractional crystallization; AFC, assimilation and fractional crystalli- zation. means of estimating magma temperatures from bulk-rock compositions. The calculated zircon saturation tempera- tures (TZr) of felsic rocks are 825˚C to 886˚C (Table 2), representing the crystallization temperature of the magma. 6. Conclusions Based on geochronological, geochemical and Sr-Nd iso- topic studies, the following conclusions are drawn: 1) Granodiorites were formed at 252.5 ± 1.0 based on LA-ICP-MS U-Pb zircon dating. The rocks resulted from post-collision magmatism. 2) Felsic rocks came from a crustal source. The frac- tionation of K-feldspar, plagioclase, ilmenite, or rutile, among others, resulted in granodiorites with negligible crustal contamination. The zircon saturation temperatures (TZr) of the granodiorites range from 825˚C to 875˚C, approximately representing the crystallization tempera- ture of the magma. 7. Acknowledgements The present research was supported by the Knowledge Innovation Project (KZCX2-YW-111-03) and the Na- Copyright © 2012 SciRes. OJG
 S. LIU ET AL. 239 tional Nature Science Foundation of China (40773020, 40972071, 90714010 and 40634020). The authors grate- fully acknowledge Lian Zhou for helping analyze the Sr-Nd isotopes and Yongsheng Liu and Zhaochu Hu for their help with the LA-ICP-MS zircon U-Pb dating. REFERENCES [1] Southwest Geological Exploration Corporation, “Geology of Tin Deposits in Gejiu,” Metallurgical Industry Pub- lishing House, Beijing, 1984. [2] J. Chen, C. Hall and C. J. Stanley, “Tin-Bearing Skarns of South China: Geological Setting and Mineralogy,” Ore Geology Reviews, Vol. 7, No. 3, 1992, pp. 225-248. doi:10.1016/0169-1368(92)90006-7 [3] Y. Q. Zhuang, R. Z. Wang and J. M. Yin, “Geology of the Gejiu Tin-Copper Olymetallic Deposit,” Geological Publishing House, Beijing, 1996. [4] Z. W. Jiang, N. H. S. Oliver, T. D. Barr W. L. Power and A. Ord, “Numerical Modeling of Fault-Controlled Fluid Flow in the Genesis of the Deposits of the Malage Ore District, Gejiu Mining District, China,” Economic Geo- logy, Vol. 92, No. 2. 1997, pp. 228-247. doi:10.2113/gsecongeo.92.2.228 [5] G. P. Mo, “Genetic Type of Granites in Gejiu Super Large Tin Polymetallic Deposit,” Mineral Resources and Geo- logy, Vol. 20, No. 45, 2006, pp. 413-417. [6] Y. B. Cheng and J. W. Mao, “Age and Geochemistry of Granites in Gejiu Area, Yunnan Province, SW China: Constraints on Their Petrogenesis and Tectonic Setting,” Lithos, Vol. 120, No. 3-4, 2010, pp. 258-276. doi:10.1016/j.lithos.2010.08.013 [7] The Second Geological Team of Yunnan Geological and Mineral Urea, “The Report of Prospecting at the Region of Bainiuchang in Mengzi County, Yunnan Province,” Yunnan Ecological and Mineral Bureau, Mengzi County, 1990. [8] Z. Y. Gao, “On the Genesis of the Bainiuchang Silver- Polymetallic Deposit in Mengzi,” Yunnan Geology, Vol. 15, No. 4, 1996, pp. 91-102. [9] X. M. Chen, Z. Lin and F. H. Xie, “Geological and Geo- chemical Characteristics of the Bainiuehang Super Large Silver Polymetallic Deposit of Supreme Posed Minerali- zation, Yunnan Province,” Scientia Geologica Sinica, Vol. 33, No. 1, 1998, pp. 115-123. [10] X. B. Li, J. S. Liu, H. P. Zhang and G. Ma, “The Analysis to Ore-Controlling Factors of the Bainiuehang Ag Poly- metallic Deposit of Mengzi County in Yunnan Province,” Contribution to Geology and Mineral Resources Re- search, Vol. 20, No. 2, 2005, pp.111-114. [11] H. J. Xie, C. H. Zhu, Q. Zhang, Q. Wang and L. W. Fan, “Sulfur Isotopic Composition of the Bainiuchang Super Large Ag Polymetallic Deposit, Yunnan Province, China: Implications for the Source of Sulfur in Ore Forming Fl- uids,” Chinese Journal of Geochemistry, Vol. 28, No. 3, 2009, pp. 284-292. doi:10.1007/s11631-009-0284-6 [12] L. Ye, N. J. Cook, C. L. Ciobanu, Y. P. Liu, Q. Zhang, T. G. Liu, W. Gao, Y. L. Yang and L. Danyushevskiy, “Trace and Minor Elements in Sphalerite from Base Metal De- posits in South China: A La-Icpms Study,” Ore Geology Reviews, Vol. 39, No. 4, 2011, pp. 188-217. doi:10.1016/j.oregeorev.2011.03.001 [13] C. H. Zhu, Q. Zhang, S. X. Shao and D. P. Wang, “Origin of Bainiuchang Liver-Polymetallic Deposit in Yunnan, China,” Global Geology, Vol. 25, No. 4, 2006, pp. 353- 359. [14] C. H. Zhu, Q. Zhang, S. X. Shao, X. Q. Zhu and D. Q. Wang, “ Lead Isotopic Composition and Lead Source in the Bainiuchang Ag-Polymetallic Deposit, Yunnan Pro- vince, China,” Acta Geologica Sinica, Vol. 82, No. 5, 2008, pp. 845-857. [15] H. L. Yuan, S. Gao, X. M. Liu, H. M. Li, D. Gunther and F. Y. Wu, “Accurate U-Pb Age and Trace Element De- terminations of Zircon by Laser Ablation-Inductively Coupled Plasma Mass Spectrometry,” Geostandards Newsletter, Vol. 28, No. 3, 2004, pp. 353-370. doi:10.1111/j.1751-908X.2004.tb00755.x [16] T. Andersen, “Correction of Common Lead in U-Pb Ana- lyses That Do Not Report 204Pb,” Chemical Geology, Vol. 192, No. 1-2, 2002, pp. 59-79. doi:10.1016/S0009-2541(02)00195-X [17] K. R. Ludwig, “User’s Manual for Isoplot/Ex, Version 3.00. A Geochronological Toolkit for Microsoft Excel,” Berkeley Geochronology Center Special Publication, Vol. 4, No. 2, 2003, pp. 1-70. [18] L. Qi, J. Hu and D. C. Grégoire, “Determination of Trace Elements in Granites by Inductively Coupled Plasma Mass Spectrometry,” Talanta, Vol. 51, No. 6, 2000, pp. 507-513. [19] H. E. Huppert and R. S. J. Sparks, “The Generation of Granitic Magmas by Intrusion of Basalt into Continental Crust,” Journal of the Petrology, Vol. 29, No. 3, 1998, pp. 599-624. [20] C. Annen and R. S. J. Sparks, “Effects of Repetitive Em- placement of Basaltic Intrusions on Thermal Evolution and Melt Generation in the Crust,” Earth Planetary Sci- ence Letters, Vol. 203, No. 3-7, 2002, pp. 937-955. [21] F. Y. Wu, X. H. Li and J. H. Yang, “Discussions on the Petrogenesis of Granites,” Acta Petrologica Sinica, Vol. 23, No. 6, 2007, pp. 1217-1238. [22] H. F. Ling, W. Z. Shen and P. Deng, “Age, Geochemistry and Petrogenesis of the Sundong Granite, Northern Guangdong Province,” Acta Petrologica Sinica, Vol. 20, No. 3, 2004, pp. 413-424. [23] J. H. Yu, X. M. Zhou and L. Zhao, “Mantle-Crust Inter- action Generating the Wuping Granites: Evidence from Sr-Nd-Hf-U-Pb Isotopes,” Acta Petrologica Sinica, Vol. 21, No. 3, 2005, pp. 651-664. [24] J. S. Qiu, J. Hu and B. I. A. McInnes, “Geochronology, Geochemistry and Petrogenesis of the Longwo Granodio- ritic Pluton in Guangdong Province,” Acta Petrologica Sinica, Vol. 20, No. 6, 2004, pp. 1363-1374. [25] S. Y. Jiang, L. Li, B. Zhu, X. Ding, Y. H. Jiang, L. X. Gu and P. Ni, “Geochemical and Sr-Nd-Hf Isotopic Compo- sitions of Granodiorite from the Wushan Copper Deposit, Copyright © 2012 SciRes. OJG
 S. LIU ET AL. Copyright © 2012 SciRes. OJG 240 Jiangxi Province and Their Implications for Petrogene- sis,” Acta Petrologica Sinica, Vol. 24, No. 8, 2008, pp. 1679-1690. [26] D. J. DePaolo, “Trace Element and Isotopic Effects of Combined Wallrock Assimilation and Fractionation Cry- stallization,” Earth and Planetary Science Letters, Vol. 53, No. 2, 1981, pp. 189-202. doi:10.1016/0012-821X(81)90153-9 [27] C. W. Devey and K. G. Cox, “Relationships between Cru- stal Contamination and Crystallization in Continental Flood Basalt Magmas with Special Reference to the Dec- can Traps of the Western Ghats, India,” Earth and Plane- tary Science Letters, Vol. 84, No. 1, 1987, pp. 59-68. doi:10.1016/0012-821X(87)90176-2 [28] J. S. Marsh, “Geochemical Constraints on Coupled As- similation and Fractional Crystallization Involving Upper Crustal Compositions and Continental Tholeiitic Mag- ma,” Earth Planetary Science Letter, Vol. 92, No. 1, 1989, pp. 78-80. doi:10.1016/0012-821X(89)90021-6 [29] B. Mingram, R. B. Trumbull, S. Littman and H. Gerten- berger, “A Petrogenetic Study of Anorogenic Felsic Mag- matism in the Cretaceous Paresis Ring Complex, Namibia: Evidence for Mixing of Crust and Mantle-Derived Com- ponents,” Lithos, Vol. 54, No. 1-2, 2000, pp. 1-22. doi:10.1016/S0024-4937(00)00033-5 [30] P. J. Sylvester, “Post-Collisional Strongly Peraluminous Granites,” Lithos, Vol. 45, No. 1-4, 1998, pp. 29-34. doi:10.1016/S0024-4937(98)00024-3 [31] Q. Zhang, Y. Wang and C. D. Li, “Granite Classification on the Basis of Sr and Yb Contents and Its Implications,” Acta Petrologica Sinica, Vol. 22, No. 9, 2006, pp. 2249- 2269. [32] X. H. Li, Z. X. Li, W. X. Li, Y. Liu, C Yuan, G. J. Wei and C. S. Qi, “U-Pb Zircon, Geochemical and Sr-Nd-Hf Isotopic Constraints on Age and Origin of Jurassic I and A-Type Granites from Central Guangdong, SE China: A Major Igneous Event in Response to Foundering of a Subducted Flat-Slab,” Lithos, Vol. 96, No. 2, 2007, pp. 186-204. doi:10.1016/j.lithos.2006.09.018 [33] E. B. Watson and T. M. Harrison, “Zircon Saturation Revisited: Temperature and Composition Effects in a Va- riety of Crustal Magma Types,” Earth and Planetary Science Letters, Vol. 64, No. 2, 1983, pp. 295-304. doi:10.1016/0012-821X(83)90211-X [34] E. A. K Middlemost, “Naming Materials in the Magma/ Igneous Rock System,” Earth-Science Reviews, Vol. 37, No. 3-4, 1994, pp. 215-224. doi:10.1016/0012-8252(94)90029-9 [35] R. W. Le Maitre, “Igneous Rocks: A Classification and Glossary of Terms,” 2nd Edition, Cambridge University Press, Cambridge, 2002. [36] E. A. K. Middlemost, “A Simple Classification of Volca- nic Rocks,” Bulletin of Volcanology, Vol. 36, No. 2, 1972, pp. 382-397. doi:10.1007/BF02596878 [37] S. S. Sun and W. F. McDonough, “Chemical and Isotopic Systematics of Oceanic Basalts: Implications for Mantle Composition and Processes. Magmatism in the Ocean Basins,” In: A. D. Saunders and M. J. Norry, Eds., Geo- logical Society Special Publication, London, 1989, pp. 313-345. [38] Y. M. Wang, Y. S. Gao, H. M. Han and X. H. Wang, “Practical Handbook of Reference Materials for Geoana- lysis,” Geological Publishing House, Beijing, 2003. [39] M. Thompson, P. J. Potts, J. S. Kane and S. Wilson, “An International Proficiency Test for Analytical Geochemis- try Laboratories-Report on Round 5 (August 1999),” Geo- standards and Geoanalytical Research, Vol. 24, No. 1, 2000, pp. E1-E28. [40] P. J. Potts and J. S. Kane, “International Association of Geoanalysts Certificate of Analysis: Certified Reference Material OU-6 (Penrhyn Slate),” Geostandards and Geo- analytical Research, Vol. 29, No. 2, 2005, pp. 233-236. doi:10.1111/j.1751-908X.2005.tb00895.x [41] R. H. Steiger and E. Jäger, “Subcommission on Geochro- nology; Convention on the Use of Decay Constants in Geochronology and Cosmochronology,” Earth and Plane- tary Science Letters, Vol. 36, No. 3, 1977, pp. 359-362. [42] G. W. Lugmair and K. Harti, “Lunar Initial 143Nd/144Nd: Differential Evolution of the Lunar Crust and Mantle,” Earth and Planetary Science Letters, Vol. 39, No. 3, 1978, pp. 349-357. doi:10.1016/0012-821X(78)90021-3
|