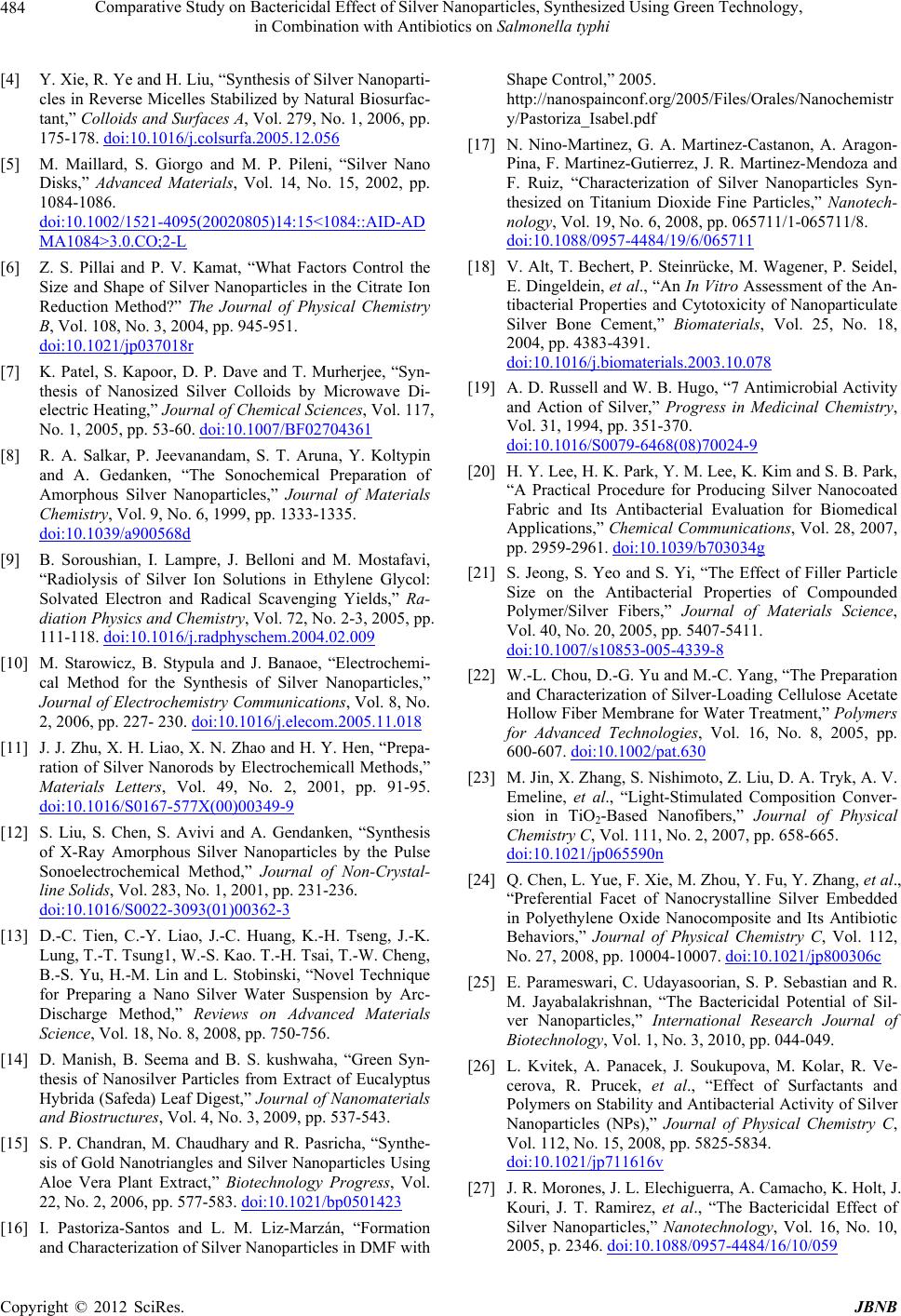
Comparative Study on Bactericidal Effect of Silver Nanoparticles, Synthesized Using Green Technology,
in Combination with Antibiotics on Salmonella typhi
484
[4] Y. Xie, R. Ye and H. Liu, “Synthesis of Silver Nanoparti-
cles in Reverse Micelles Stabilized by Natural Biosurfac-
tant,” Colloids and Surfaces A, Vol. 279, No. 1, 2006, pp.
175-178. doi:10.1016/j.colsurfa.2005.12.056
[5] M. Maillard, S. Giorgo and M. P. Pileni, “Silver Nano
Disks,” Advanced Materials, Vol. 14, No. 15, 2002, pp.
1084-1086.
doi:10.1002/1521-4095(20020805)14:15<1084::AID-AD
MA1084>3.0.CO;2-L
[6] Z. S. Pillai and P. V. Kamat, “What Factors Control the
Size and Shape of Silver Nanoparticles in the Citrate Ion
Reduction Method?” The Journal of Physical Chemistry
B, Vol. 108, No. 3, 2004, pp. 945-951.
doi:10.1021/jp037018r
[7] K. Patel, S. Kapoor, D. P. Dave and T. Murherjee, “Syn-
thesis of Nanosized Silver Colloids by Microwave Di-
electric Heating,” Journal of Chemical Sciences, Vol. 117,
No. 1, 2005, pp. 53-60. doi:10.1007/BF02704361
[8] R. A. Salkar, P. Jeevanandam, S. T. Aruna, Y. Koltypin
and A. Gedanken, “The Sonochemical Preparation of
Amorphous Silver Nanoparticles,” Journal of Materials
Chemistry, Vol. 9, No. 6, 1999, pp. 1333-1335.
doi:10.1039/a900568d
[9] B. Soroushian, I. Lampre, J. Belloni and M. Mostafavi,
“Radiolysis of Silver Ion Solutions in Ethylene Glycol:
Solvated Electron and Radical Scavenging Yields,” Ra-
diation Physics and Chemistry, Vol. 72, No. 2-3, 2005, pp.
111-118. doi:10.1016/j.radphyschem.2004.02.009
[10] M. Starowicz, B. Stypula and J. Banaoe, “Electrochemi-
cal Method for the Synthesis of Silver Nanoparticles,”
Journal of Electrochemistry Communications, Vol. 8, No.
2, 2006, pp. 227- 230. doi:10.1016/j.elecom.2005.11.018
[11] J. J. Zhu, X. H. Liao, X. N. Zhao and H. Y. Hen, “Prepa-
ration of Silver Nanorods by Electrochemicall Methods,”
Materials Letters, Vol. 49, No. 2, 2001, pp. 91-95.
doi:10.1016/S0167-577X(00)00349-9
[12] S. Liu, S. Chen, S. Avivi and A. Gendanken, “Synthesis
of X-Ray Amorphous Silver Nanoparticles by the Pulse
Sonoelectrochemical Method,” Journal of Non-Crystal-
line Solids, Vol. 283, No. 1, 2001, pp. 231-236.
doi:10.1016/S0022-3093(01)00362-3
[13] D.-C. Tien, C.-Y. Liao, J.-C. Huang, K.-H. Tseng, J.-K.
Lung, T.-T. Tsung1, W.-S. Kao. T.-H. Tsai, T.-W. Cheng,
B.-S. Yu, H.-M. Lin and L. Stobinski, “Novel Technique
for Preparing a Nano Silver Water Suspension by Arc-
Discharge Method,” Reviews on Advanced Materials
Science, Vol. 18, No. 8, 2008, pp. 750-756.
[14] D. Manish, B. Seema and B. S. kushwaha, “Green Syn-
thesis of Nanosilver Particles from Extract of Eucalyptus
Hybrida (Safeda) Leaf Digest,” Journal of Nanomaterials
and Biostructures, Vol. 4, No. 3, 2009, pp. 537-543.
[15] S. P. Chandran, M. Chaudhary and R. Pasricha, “Synthe-
sis of Gold Nanotriangles and Silver Nanoparticles Using
Aloe Vera Plant Extract,” Biotechnology Progress, Vol.
22, No. 2, 2006, pp. 577-583. doi:10.1021/bp0501423
[16] I. Pastoriza-Santos and L. M. Liz-Marzán, “Formation
and Characterization of Silver Nanoparticles in DMF with
Shape Control,” 2005.
http://nanospainconf.org/2005/Files/Orales/Nanochemistr
y/Pastoriza_Isabel.pdf
[17] N. Nino-Martinez, G. A. Martinez-Castanon, A. Aragon-
Pina, F. Martinez-Gutierrez, J. R. Martinez-Mendoza and
F. Ruiz, “Characterization of Silver Nanoparticles Syn-
thesized on Titanium Dioxide Fine Particles,” Nanotech-
nology, Vol. 19, No. 6, 2008, pp. 065711/1-065711/8.
doi:10.1088/0957-4484/19/6/065711
[18] V. Alt, T. Bechert, P. Stei nrücke, M. Wagener, P. Seidel,
E. Dingeldein, et al., “An In Vitro Assessment of the An-
tibacterial Properties and Cytotoxicity of Nanoparticulate
Silver Bone Cement,” Biomaterials, Vol. 25, No. 18,
2004, pp. 4383-4391.
doi:10.1016/j.biomaterials.2003.10.078
[19] A. D. Russell and W. B. Hugo, “7 Antimicrobial Activity
and Action of Silver,” Progress in Medicinal Chemistry,
Vol. 31, 1994, pp. 351-370.
doi:10.1016/S0079-6468(08)70024-9
[20] H. Y. Lee, H. K. Park, Y. M. Lee, K. Kim and S. B. Park,
“A Practical Procedure for Producing Silver Nanocoated
Fabric and Its Antibacterial Evaluation for Biomedical
Applications,” Chemical Communications, Vol. 28, 2007,
pp. 2959-2961. doi:10.1039/b703034g
[21] S. Jeong, S. Yeo and S. Yi, “The Effect of Filler Particle
Size on the Antibacterial Properties of Compounded
Polymer/Silver Fibers,” Journal of Materials Science,
Vol. 40, No. 20, 2005, pp. 5407-5411.
doi:10.1007/s10853-005-4339-8
[22] W.-L. Chou, D.-G. Yu and M.-C. Yang, “The Preparation
and Characterization of Silver-Loading Cellulose Acetate
Hollow Fiber Me mbrane for Water Treatme nt,” Polymers
for Advanced Technologies, Vol. 16, No. 8, 2005, pp.
600-607. doi:10.1002/pat.630
[23] M. Jin, X. Zhang, S. Nishimoto, Z. Liu, D. A. Tryk, A. V.
Emeline, et al., “Light-Stimulated Composition Conver-
sion in TiO2-Based Nanofibers,” Journal of Physical
Chemistry C, Vol. 111, No. 2, 2007, pp. 658-665.
doi:10.1021/jp065590n
[24] Q. Chen, L. Yue, F. Xie, M. Zhou, Y. Fu, Y. Zhang, et al.,
“Preferential Facet of Nanocrystalline Silver Embedded
in Polyethylene Oxide Nanocomposite and Its Antibiotic
Behaviors,” Journal of Physical Chemistry C, Vol. 112,
No. 27, 2008, pp. 10004-10007. doi:10.1021/jp800306c
[25] E. Parameswari, C. Udayasoorian, S. P. Sebastian and R.
M. Jayabalakrishnan, “The Bactericidal Potential of Sil-
ver Nanoparticles,” International Research Journal of
Biotechnology, Vol. 1, No. 3, 2010, pp. 044-049.
[26] L. Kvitek, A. Panacek, J. Soukupova, M. Kolar, R. Ve-
cerova, R. Prucek, et al., “Effect of Surfactants and
Polymers on Stability and Antibacterial Activity of Silver
Nanoparticles (NPs),” Journal of Physical Chemistry C,
Vol. 112, No. 15, 2008, pp. 5825-5834.
doi:10.1021/jp711616v
[27] J. R. Morones, J. L. Elechiguerra, A. Camacho, K. Holt, J.
Kouri, J. T. Ramirez, et al., “The Bactericidal Effect of
Silver Nanoparticles,” Nanotechnology, Vol. 16, No. 10,
2005, p. 2346. doi:10.1088/0957-4484/16/10/059
Copyright © 2012 SciRes. JBNB