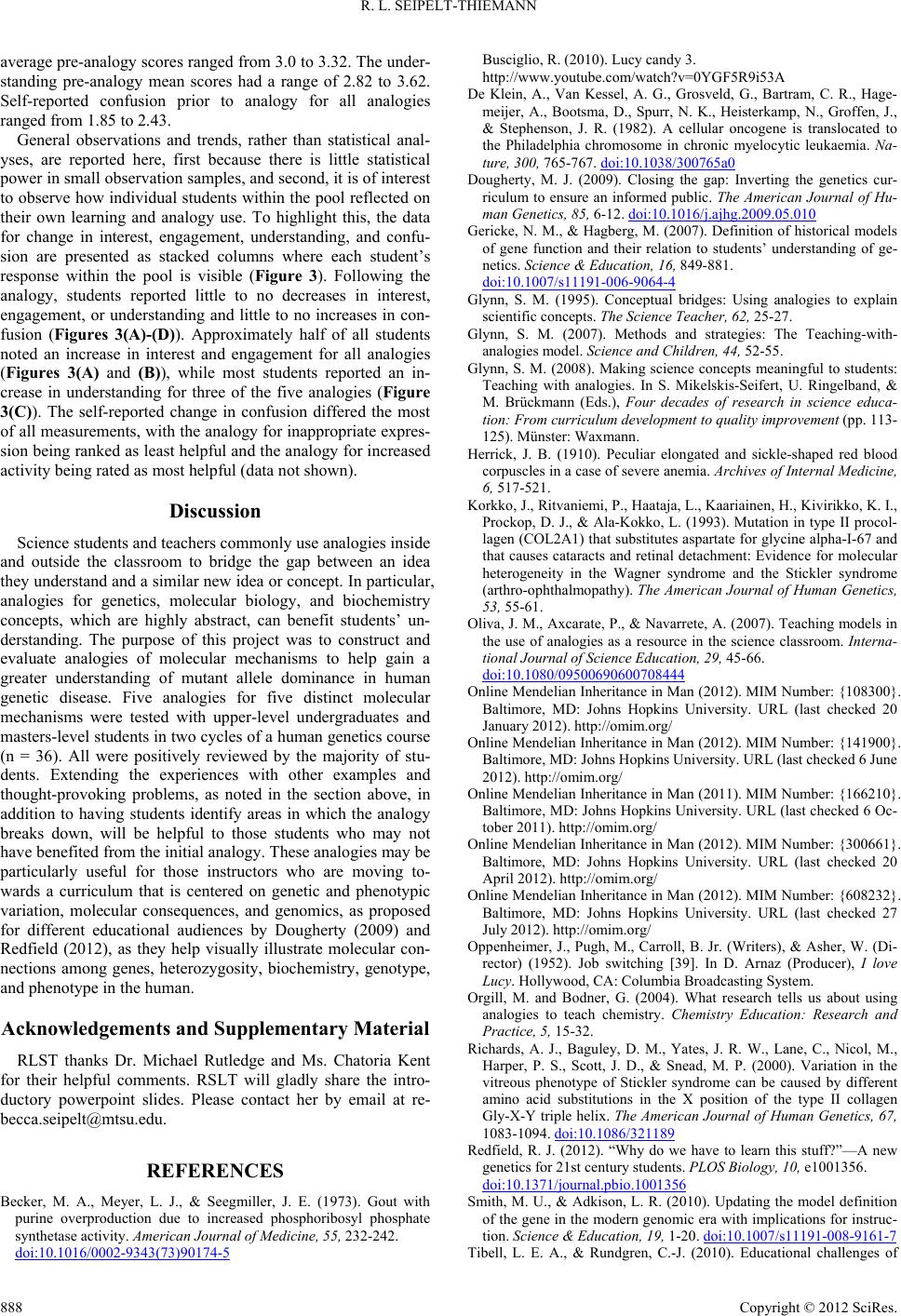
R. L. SEIPELT-THIEMANN
average pre-analogy scores ranged from 3.0 to 3.32. The under-
standing pre-analogy mean scores had a range of 2.82 to 3.62.
Self-reported confusion prior to analogy for all analogies
ranged from 1.85 to 2.43.
General observations and trends, rather than statistical anal-
yses, are reported here, first because there is little statistical
power in small observation samples, and second, it is of interest
to observe how individual students within the pool reflected on
their own learning and analogy use. To highlight this, the data
for change in interest, engagement, understanding, and confu-
sion are presented as stacked columns where each student’s
response within the pool is visible (Figure 3). Following the
analogy, students reported little to no decreases in interest,
engagement, or understanding and little to no increases in con-
fusion (Figures 3(A)-(D)). Approximately half of all students
noted an increase in interest and engagement for all analogies
(Figures 3(A) and (B)), while most students reported an in-
crease in understanding for three of the five analogies (Figure
3(C)). The self-reported change in confusion differed the most
of all measurements, with the analogy for inappropriate expres-
sion being ranked as least helpful and the analogy for increased
activity being rated as most helpful (data not shown).
Discussion
Science students and teachers commonly use analogies inside
and outside the classroom to bridge the gap between an idea
they understand and a similar new idea or concept. In particular,
analogies for genetics, molecular biology, and biochemistry
concepts, which are highly abstract, can benefit students’ un-
derstanding. The purpose of this project was to construct and
evaluate analogies of molecular mechanisms to help gain a
greater understanding of mutant allele dominance in human
genetic disease. Five analogies for five distinct molecular
mechanisms were tested with upper-level undergraduates and
masters-level students in two cycles of a human genetics course
(n = 36). All were positively reviewed by the majority of stu-
dents. Extending the experiences with other examples and
thought-provoking problems, as noted in the section above, in
addition to having students identify areas in which the analogy
breaks down, will be helpful to those students who may not
have benefited from the initial analogy. These analogies may be
particularly useful for those instructors who are moving to-
wards a curriculum that is centered on genetic and phenotypic
variation, molecular consequences, and genomics, as proposed
for different educational audiences by Dougherty (2009) and
Redfield (2012), as they help visually illustrate molecular con-
nections among genes, heterozygosity, biochemistry, genotype,
and phenotype in the human.
Acknowledgements and Supplementary Material
RLST thanks Dr. Michael Rutledge and Ms. Chatoria Kent
for their helpful comments. RSLT will gladly share the intro-
ductory powerpoint slides. Please contact her by email at re-
becca.seipelt@mtsu.edu.
REFERENCES
Becker, M. A., Meyer, L. J., & Seegmiller, J. E. (1973). Gout with
purine overproduction due to increased phosphoribosyl phosphate
synthetase activity. American Journal of Medicine, 55, 232-242.
doi:10.1016/0002-9343(73)90174-5
Busciglio, R. (2010). Lucy candy 3.
http://www.youtube.com/watch?v=0YGF5R9i53A
De Klein, A., Van Kessel, A. G., Grosveld, G., Bartram, C. R., Hage-
meijer, A., Bootsma, D., Spurr, N. K., Heisterkamp, N., Groffen, J.,
& Stephenson, J. R. (1982). A cellular oncogene is translocated to
the Philadelphia chromosome in chronic myelocytic leukaemia. Na-
ture, 300, 765-767. doi:10.1038/300765a0
Dougherty, M. J. (2009). Closing the gap: Inverting the genetics cur-
riculum to ensure an informed public. The American Journal of Hu-
man Genetics, 85, 6-12. doi:10.1016/j.ajhg.2009.05.010
Gericke, N. M., & Hagberg, M. (2007). Definition of historical models
of gene function and their relation to students’ understanding of ge-
netics. Science & Education, 16, 849-881.
doi:10.1007/s11191-006-9064-4
Glynn, S. M. (1995). Conceptual bridges: Using analogies to explain
scientific concepts. The Science Teacher, 62, 25-27.
Glynn, S. M. (2007). Methods and strategies: The Teaching-with-
analogies model. Science and Children, 44, 52-55.
Glynn, S. M. (2008). Making science concepts meaningful to students:
Teaching with analogies. In S. Mikelskis-Seifert, U. Ringelband, &
M. Brückmann (Eds.), Four decades of research in science educa-
tion: From curriculum development to quality improvement (pp. 113-
125). Münster: Waxmann.
Herrick, J. B. (1910). Peculiar elongated and sickle-shaped red blood
corpuscles in a case of severe anemia. Archives of Internal Medicine,
6, 517-521.
Korkko, J., Ritvaniemi, P., Haataja, L., Kaariainen, H., Kivirikko, K. I.,
Prockop, D. J., & Ala-Kokko, L. (1993). Mutation in type II procol-
lagen (COL2A1) that substitutes aspartate for glycine alpha-I-67 and
that causes cataracts and retinal detachment: Evidence for molecular
heterogeneity in the Wagner syndrome and the Stickler syndrome
(arthro-ophthalmopathy). The American Journal of Human Genetics,
53, 55-61.
Oliva, J. M., Axcarate, P., & Navarrete, A. (2007). Teaching models in
the use of analogies as a resource in the science classroom. Interna-
tional Journal of Science Education, 2 9, 45-66.
doi:10.1080/09500690600708444
Online Mendelian Inheritance in Man (2012). MIM Number: {108300}.
Baltimore, MD: Johns Hopkins University. URL (last checked 20
January 2012). http://omim.org/
Online Mendelian Inheritance in Man (2012). MIM Number: {141900}.
Baltimore, MD: Johns Hopkins University. URL (last checked 6 June
2012). http://omim.org/
Online Mendelian Inheritance in Man (2011). MIM Number: {166210}.
Baltimore, MD: Johns Hopkins University. URL (last checked 6 Oc-
tober 2011). http://omim.org/
Online Mendelian Inheritance in Man (2012). MIM Number: {300661}.
Baltimore, MD: Johns Hopkins University. URL (last checked 20
April 2012). http://omim.org/
Online Mendelian Inheritance in Man (2012). MIM Number: {608232}.
Baltimore, MD: Johns Hopkins University. URL (last checked 27
July 2012). http://omim.org/
Oppenheimer, J., Pugh, M., Carroll, B. Jr. (Writers), & Asher, W. (Di-
rector) (1952). Job switching [39]. In D. Arnaz (Producer), I love
Lucy. Hollywood, CA: Columbia Broadcasting System.
Orgill, M. and Bodner, G. (2004). What research tells us about using
analogies to teach chemistry. Chemistry Education: Research and
Practice, 5, 15-32.
Richards, A. J., Baguley, D. M., Yates, J. R. W., Lane, C., Nicol, M.,
Harper, P. S., Scott, J. D., & Snead, M. P. (2000). Variation in the
vitreous phenotype of Stickler syndrome can be caused by different
amino acid substitutions in the X position of the type II collagen
Gly-X-Y triple helix. The American Journal of Human Genetics, 67,
1083-1094. doi:10.1086/321189
Redfield, R. J. (2012). “Why do we have to learn this stuff?”—A new
genetics for 21st century students. PLOS Biology, 1 0 , e1001356.
doi:10.1371/journal.pbio.1001356
Smith, M. U., & Adkison, L. R. (2010). Updating the model definition
of the gene in the modern genomic era with implications for instruc-
tion. Science & Education, 19, 1-20. doi:10.1007/s11191-008-9161-7
Tibell, L. E. A., & Rundgren, C.-J. (2010). Educational challenges of
Copyright © 2012 SciRes.
888