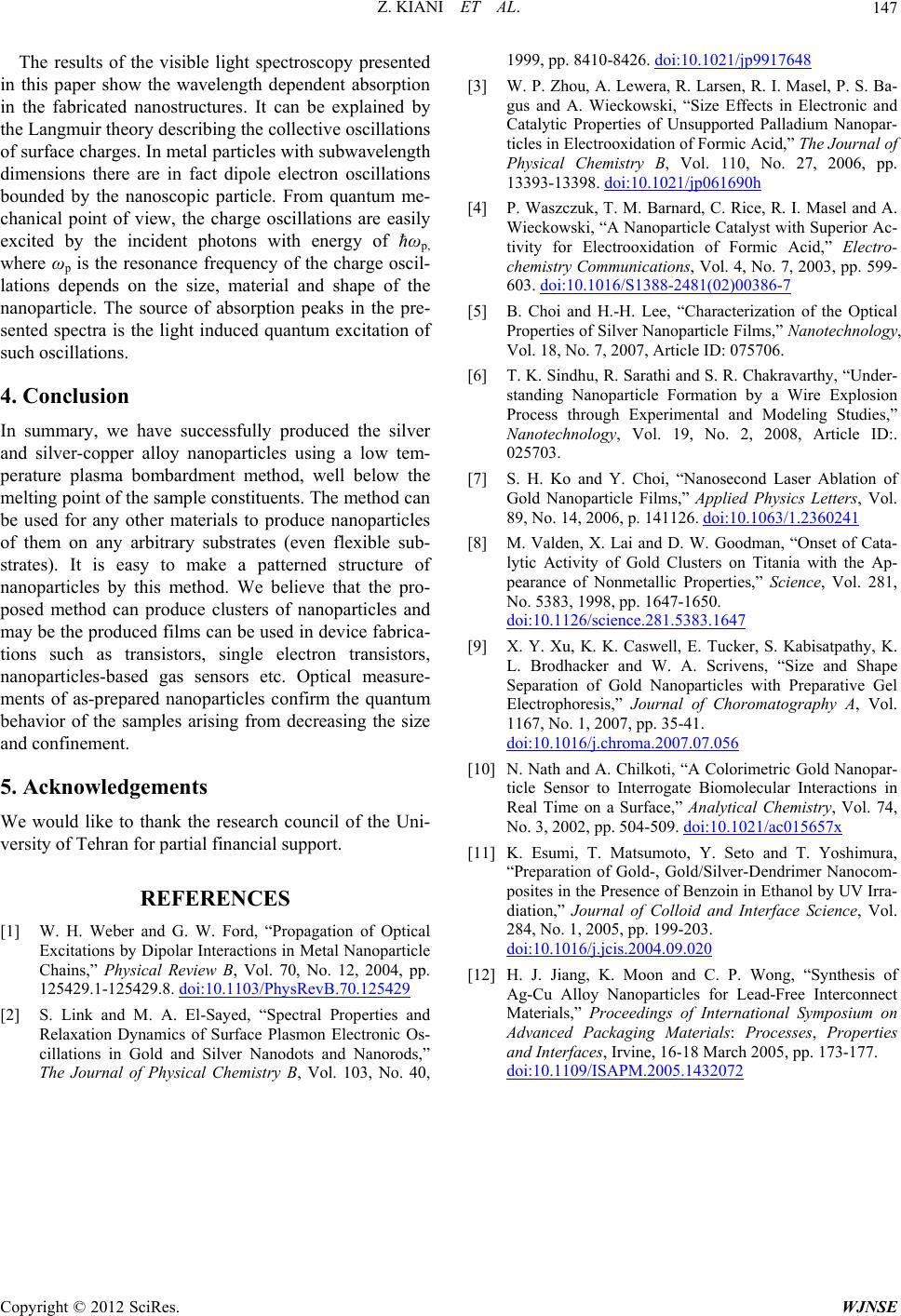
Z. KIANI ET AL.
Copyright © 2012 SciRes. WJNSE
147
The results of the visible light spectroscopy presented
in this paper show the wavelength dependent absorption
in the fabricated nanostructures. It can be explained by
the Langmuir theory describing the collective oscillations
of surface charges. In metal particles with subwavelength
dimensions there are in fact dipole electron oscillations
bounded by the nanoscopic particle. From quantum me-
chanical point of view, the charge oscillations are easily
excited by the incident photons with energy of ћωp,
where ωp is the resonance frequency of the charge oscil-
lations depends on the size, material and shape of the
nanoparticle. The source of absorption peaks in the pre-
sented spectra is the light induced quantum excitation of
such oscillations.
4. Conclusion
In summary, we have successfully produced the silver
and silver-copper alloy nanoparticles using a low tem-
perature plasma bombardment method, well below the
melting point of the sample constituents. The method can
be used for any other materials to produce nanoparticles
of them on any arbitrary substrates (even flexible sub-
strates). It is easy to make a patterned structure of
nanoparticles by this method. We believe that the pro-
posed method can produce clusters of nanoparticles and
may be the produced films can be used in device fabrica-
tions such as transistors, single electron transistors,
nanoparticles-based gas sensors etc. Optical measure-
ments of as-prepared nanoparticles confirm the quantum
behavior of the samples arising from decreasing the size
and confinement.
5. Acknowledgements
We would like to thank the research council of the Uni-
versity of Tehran for partial financial support.
REFERENCES
[1] W. H. Weber and G. W. Ford, “Propagation of Optical
Excitations by Dipolar Interactions in Metal Nanoparticle
Chains,” Physical Review B, Vol. 70, No. 12, 2004, pp.
125429.1-125429.8. doi:10.1103/PhysRevB.70.125429
[2] S. Link and M. A. El-Sayed, “Spectral Properties and
Relaxation Dynamics of Surface Plasmon Electronic Os-
cillations in Gold and Silver Nanodots and Nanorods,”
The Journal of Physical Chemistry B, Vol. 103, No. 40,
1999, pp. 8410-8426. doi:10.1021/jp9917648
[3] W. P. Zhou, A. Lewera, R. Larsen, R. I. Masel, P. S. Ba-
gus and A. Wieckowski, “Size Effects in Electronic and
Catalytic Properties of Unsupported Palladium Nanopar-
ticles in Electrooxidation of Formic Acid,” The Journal of
Physical Chemistry B, Vol. 110, No. 27, 2006, pp.
13393-13398. doi:10.1021/jp061690h
[4] P. Waszczuk, T. M. Barnard, C. Rice, R. I. Masel and A.
Wieckowski, “A Nanoparticle Catalyst with Superior Ac-
tivity for Electrooxidation of Formic Acid,” Electro-
chemistry Communications, Vol. 4, No. 7, 2003, pp. 599-
603. doi:10.1016/S1388-2481(02)00386-7
[5] B. Choi and H.-H. Lee, “Characterization of the Optical
Properties of Silver Nanoparticle Films,” Nanotechnology,
Vol. 18, No. 7, 2007, Article ID: 075706.
[6] T. K. Sindhu, R. Sarathi and S. R. Chakravarthy, “Under-
standing Nanoparticle Formation by a Wire Explosion
Process through Experimental and Modeling Studies,”
Nanotechnology, Vol. 19, No. 2, 2008, Article ID:.
025703.
[7] S. H. Ko and Y. Choi, “Nanosecond Laser Ablation of
Gold Nanoparticle Films,” Applied Physics Letters, Vol.
89, No. 14, 2006, p. 141126. doi:10.1063/1.2360241
[8] M. Valden, X. Lai and D. W. Goodman, “Onset of Cata-
lytic Activity of Gold Clusters on Titania with the Ap-
pearance of Nonmetallic Properties,” Science, Vol. 281,
No. 5383, 1998, pp. 1647-1650.
doi:10.1126/science.281.5383.1647
[9] X. Y. Xu, K. K. Caswell, E. Tucker, S. Kabisatpathy, K.
L. Brodhacker and W. A. Scrivens, “Size and Shape
Separation of Gold Nanoparticles with Preparative Gel
Electrophoresis,” Journal of Choromatography A, Vol.
1167, No. 1, 2007, pp. 35-41.
doi:10.1016/j.chroma.2007.07.056
[10] N. Nath and A. Chilkoti, “A Colorimetric Gold Nanopar-
ticle Sensor to Interrogate Biomolecular Interactions in
Real Time on a Surface,” Analytical Chemistry, Vol. 74,
No. 3, 2002, pp. 504-509. doi:10.1021/ac015657x
[11] K. Esumi, T. Matsumoto, Y. Seto and T. Yoshimura,
“Preparation of Gold-, Gold/Silver-Dendrimer Nanocom-
posites in the Presence of Benzoin in Ethanol by UV Irra-
diation,” Journal of Colloid and Interface Science, Vol.
284, No. 1, 2005, pp. 199-203.
doi:10.1016/j.jcis.2004.09.020
[12] H. J. Jiang, K. Moon and C. P. Wong, “Synthesis of
Ag-Cu Alloy Nanoparticles for Lead-Free Interconnect
Materials,” Proceedings of International Symposium on
Advanced Packaging Materials: Processes, Properties
and Interfaces, Irvine, 16-18 March 2005, pp. 173-177.
doi:10.1109/ISAPM.2005.1432072